The perils of poly- and perfluorinated chemicals on the reproductive health of humans, livestock, and wildlife
Mark P. Green A , Cameron Shearer B , Rebecca Patrick C , Shervin Kabiri D , Nicola Rivers

A
B
C
D
E
F
G
Abstract
Poly- and perfluoroalkyl substances (PFAS) are a prominent class of persistent synthetic compound. The widespread use of these substances in various industrial applications has resulted in their pervasive contamination on a global scale. It is therefore concerning that PFAS have a propensity to accumulate in bodily tissues whereupon they have been linked with a range of adverse health outcomes. Despite this, the true extent of the risk posed by PFAS to humans, domestic animals, and wildlife remains unclear. Addressing these questions requires a multidisciplinary approach, combining the fields of chemistry, biology, and policy to enable meaningful investigation and develop innovative remediation strategies. This article combines the perspectives of chemists, soil scientists, reproductive biologists, and health policy researchers, to contextualise the issue of PFAS contamination and its specific impact on reproductive health. The purpose of this article is to describe the challenges associated with remediating PFAS-contaminated soils and waters and explore the consequences of PFAS contamination on health and reproduction. Furthermore, current actions to promote planetary health and protect ecosystems are presented to instigate positive social change among the scientific community.
Keywords: emerging contaminants, human health, livestock, persistent synthetic compounds, planetary health, poly- and perfluoroalkyl substances (PFAS), remediation, reproduction, reproductive health, wildlife.
Introduction
Optimal reproductive function requires an environment that adequately supports critical processes such as gametogenesis, mating, pregnancy, and the nurturing of offspring. Humans are having an increasingly negative influence on the environment with knock on consequences for the reproductive health of numerous species (Aulsebrook et al. 2020). The extent to which human activity has impacted Earth’s geology and ecosystems has likely crossed an epoch-scale boundary resulting in the proposal of a new epoch of geological time, the ‘Anthropocene’ (Zalasiewicz et al. 2011).
In the hope of promoting environmental stability, nine ‘planetary boundaries’ were defined as a framework to quantify and monitor the impact of human activity on the Earth’s biophysical systems (Rockstrom et al. 2009). The planetary boundaries define thresholds that should not be breached to ensure sustainable human activity without causing significant harm to the ecosystems upon which humans rely for our prosperity and wellbeing (Steffen et al. 2015). In 2022, the threshold for novel entities (i.e. chemical pollution) was exceeded due to the large production and release of human-derived chemicals outweighing the capacity to monitor and breakdown such compounds (Persson et al. 2022). Of particular concern are compounds deemed to be resistant to typical methods of breakdown, causing them to be classified as ‘persistent’. These persistent compounds can bioaccumulate in the tissues of humans, wildlife, and agricultural species resulting in potential long-term effects on biological function.
Poly- and perfluoroalkyl substances (PFAS) are a prominent class of synthetic chemicals that fall into the category of persistent compounds (Buck et al. 2011). These substances have been extensively used in various industrial applications since the 1950s, ranging from non-stick coatings to firefighting foams and, unfortunately, the widespread use and disposal of PFAS have resulted in their pervasive contamination on a global scale (Kurwadkar et al. 2022). Current evidence suggests PFAS contamination can result in elevated cholesterol, ulcerative colitis, and thyroid and kidney disease (Steenland et al. 2020). It is estimated that the disease burden and social costs associated with PFAS exposure in the USA could amount to between US$5.52 billion and US$62.6 billion annually (Obsekov et al. 2023). In response to the mounting evidence of the negative impacts of PFAS on health, PFAS contamination has recently featured at the centre of two large class action settlements in 2023, one in Australia (Australian Associated Press 2023) and one in the USA (Friedman and Giang 2023).
Despite now being ubiquitous in the environment, clear data regarding the impact of PFAS on reproduction in humans, domestic animals, and wildlife is lacking. Emerging evidence indicates that PFAS can be passed to the next generation through the placenta and lactation (Gronnestad et al. 2017). The effects of PFAS on reproductive function is of particular concern for species that are already at risk of extinction due to declining population numbers. The potential far-reaching consequences of PFAS contamination on reproductive health necessitates urgent action to identify and address the pervasiveness of PFAS in the environment.
Addressing the persistent nature of chemicals like PFAS requires a multidisciplinary approach, combining the fields of chemistry, biology, and policy to adequately investigate and develop innovative remediation strategies. This article combines the perspectives of chemists, soil scientists, reproductive biologists, and health policy researchers, to contextualise the issue of PFAS contamination and its impact on reproduction. The purpose of this article is to describe the challenges associated with remediating PFAS-contaminated soils and waters and explore the consequences of PFAS contamination on health and reproduction. Furthermore, current actions to promote planetary health and protect ecosystems are presented to instigate positive social change among the scientific community.
What makes some chemicals persistent?
The conversion of chemicals is a fundamental aspect of nature. Examples include the conversion of CO2 into sugars during photosynthesis, microbial breakdown of complex organic matter into rich soils (de Vries and Wallenstein 2017), and the weathering of limestone and rock by water to create natural landmarks. Chemicals in nature are generally converted through one (or a combination) of reactions with water, sunlight, air, or microbial action.
Chemicals that are resistant to conversion through any of these means are generally persistent in nature. The Stockholm Convention (2023) maintains a list of organic chemicals that a panel of experts have deemed persistent, widespread, bioaccumulate, and harmful. Notably, all listed chemicals contain carbon to halogen bonds (C-F, C-Cl, C-Br). These bonds are very strong; sunlight does not have sufficient energy to break them (Stockholm Convention 2023), they react very slowly in water and are rare in nature, so efficient microbial processes have not yet developed to breakdown these human-made chemicals.
Poly- and perfluoroalkyl substances
Poly- and perfluoroalkyl substances are a class of synthetic chemicals which consist of a hydrophobic tail with either a fully or partially fluorinated carbon chain and a hydrophilic head group, such as a carboxylic acid or sulfonate (Buck et al. 2011) (Fig. 1a). PFAS most commonly consist of a single carbon chain with 4–10 carbons (c4–c10), with the most prevalent being perfluorooctanoic acid (PFOA) and perfluorooctanesulfonic acid (PFOS) (Fig. 1a). However, more than 4000 different PFAS have been used in industry including straight chains, branched isomers, as well as production by-products and precursors (Wang et al. 2018).
Summary of common poly- and perfluoroalkyl substances (PFAS) structure, nomenclature, and representative concentrations in contaminated groundwater and compositional profile in human blood. (a) PFAS are a class of synthetic chemicals consisting of a hydrophobic tail with either a fully or partially fluorinated carbon chain, which are broadly grouped on the basis of their hydrophilic head group consisting of different functional group e.g. carboxylic acid or sulfonate. PFAS most commonly consist of a single carbon chain with 4–10 carbons, with two of the most prevalent being perfluorooctanesulfonic acid (PFOS) and perfluorooctanoic acid (PFOA). The extensive use and disposal of PFAS has resulted in significant release to the environment where their inherent stability has led to pervasive contamination on a global scale, with representative levels of 10 major PFAS analytes found in groundwater (natural, undiluted) from a monitoring well located at Williamtown Airforce Base, NSW, Australia (adapted from Turner et. al. 2019); all of which are orders of magnitude above that considered safe drinking water levels (i.e. 0.004 ng/L for PFOA and 0.02 ng/L for PFOS; United States Environmental Protection Agency (US EPA) 2022). (b) A concerning consequence of this contamination is that PFAS are readily detected in human blood sampled from populations across the globe (adapted from Yeung et al. 2006).
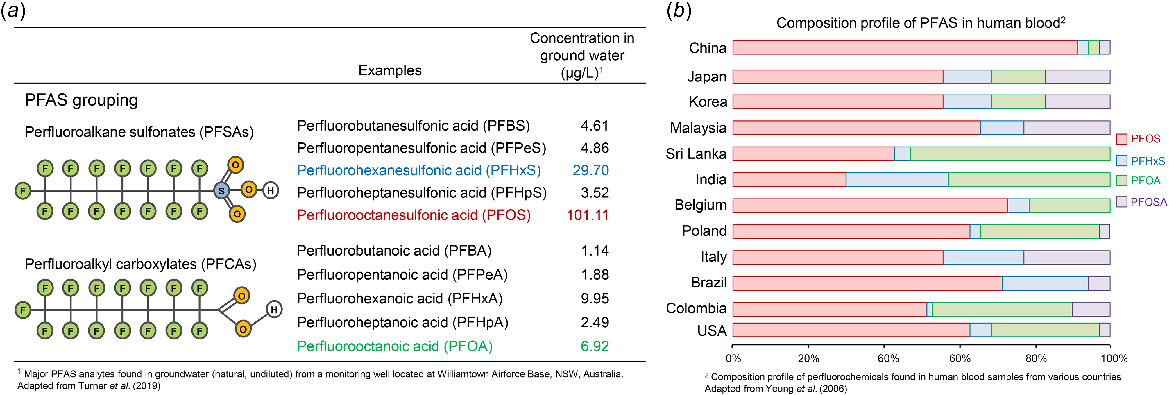
Poly- and perfluoroalkyl substances have found a myriad of applications since the 1950s including adhesives, non-stick cooking surfaces (e.g. Teflon), water-repelling coatings (e.g. Scotch Guard), anti-corrosive coatings on metals (e.g. Zonyl FSP), anti-fogging coatings on glass and mirror, cosmetics, inks, lubricants, leather treatments, emulsifiers, wetting agents in paints, herbicides, insecticides and coatings, and as aqueous film-forming foams (AFFF) for fire-fighting foams (Kissa 2001). The extensive use and disposal of PFAS has resulted in significant release to the environment where their inherent stability has led to pervasive contamination on a global scale (Moody and Field 1999; Weiß et al. 2012; Hepburn et al. 2019; Turner et al. 2019) (Fig. 1a).
In humans, PFAS are routinely detected in blood and organs in people without industrial exposure (Hansen et al. 2001; Yeung et al. 2006; Calafat et al. 2007; Perez et al. 2013; Ye et al. 2018) (Fig. 1b). In June 2022, the US Environmental Protection Agency announced an interim health advisory, which reduced the safe drinking water level of some PFAS (i.e. PFOA and PFOS) to 0.004 ng/L for PFOA and 0.02 ng/L for PFOS (previous value was 70 ng/L for each) (United States Environmental Protection Agency (US EPA) 2022). The large decrease in the safe drinking water level of PFAS will require tremendous improvements in PFAS remediation considering the combined PFOA and PFOS concentration is currently measured at 1 ng/L or greater in the drinking water for upwards of 200 million people in the USA alone (Andrews and Naidenko 2020). Notably, no alternative to substantial remediation projects is possible considering PFAS are detected in bottled water at quantities greater than the new safe limits (Chow et al. 2021).
Perspective for soil and water rehabilitation
The extensive application of PFAS in the last seven decades has resulted in near-ubiquitous contamination of soils, sediments, groundwater, and surface water including drinking water with several studies showing that soils contaminated with PFAS can serve as a significant long-term source of PFAS release (Strynar et al. 2012; Brusseau et al. 2020). Soil contamination occurs with the direct application of PFAS-laden AFFFs (Nickerson et al. 2020), via atmospheric deposition or with the deposition of PFAS-contaminated materials including biosolids, municipal sludges, and irrigation water (Bolan et al. 2021). Similarly, an abundance of landfilled PFAS-containing products has led to landfill leachates becoming another source of soil and groundwater contamination.
Once present in the soil matrix, PFAS can interact with different soil components before leaching down to groundwater or moving laterally to surface waters in runoff from rainfall. PFAS interactions with soil are complex, but are always related to the soil’s physical and chemical properties (e.g. organic carbon content, texture (sand, silt, clay content), pH, salt and mineral contents of the soil), and PFAS chemistry (carbon-fluorine chain length, functional head group and charge) (Li et al. 2018; Kabiri et al. 2022). Critically, many PFAS, such as PFOA and PFOS, are anionic at environmental pH and can be repelled by soil or groundwater’s negatively charged components such that they are relatively mobile in the soil and groundwater and can travel kilometres from the source zone (Interstate Technology and Regulatory Council (ITRC) 2023). Another major challenge related to PFAS presence in soil or water is the transformation of precursors (polyfluorinated PFAS) to perfluorinated PFAS. Many precursor chemicals bind strongly to soils, creating a stable long-term source zone that consistently releases more mobile PFAS species as they transform (Lenka et al. 2021). Furthermore, many of these compounds go undetected in the environment as most of them are not detectable by conventional analytical techniques (Ross et al. 2018).
One of the major routes of human exposure to PFAS is the consumption of PFAS-contaminated food or water (Brown et al. 2020) (Fig. 2). Literature-derived data has revealed elevated concentrations of PFAS being detected in certain foods, including eggs (Bao et al. 2019), grains (Noorlander et al. 2011), vegetables, fruits (Herzke et al. 2013; Li et al. 2019) milk, and meat (Guruge et al. 2008; Li et al. 2019) that are produced close to contaminated sites. Dietary survey data and toxicokinetic modelling has also estimated that seafood accounts for up to 86% of total PFOS exposure for adults (EFSA CONTAM Panel et al. 2020). By contrast, in infants, eggs and egg products were the most important source of chronic exposure to PFOS (up to 42%) and drinking water was the dominant source of PFOA exposure (up to 60%) (EFSA CONTAM Panel et al. 2020). Food contamination occurs when foods are grown in contaminated soils or fished from contaminated waters, and in the former case may be further intensified by the application of contaminated water and fertiliser products (Sunderland et al. 2019). Therefore, widespread presence of PFAS in foods and their hazard to human health demands their removal from diverse soil and water sources. However, the varied chemistry of PFAS, complex environmental interactions, and the extremely high standard of removal (parts per trillion) required to reduce their hazard, complicate their clean-up. Practical approaches to clean-up PFAS from the environment contain the following steps (CRC CARE 2018): (1) preliminary and detailed investigation of contaminated sites; (2) development of conceptual site models to identify the source of PFAS and potential migration pathway; (3) risk assessment of the contaminated site; (4) site management and remediation technology; and (5) management of the residual contamination.
Summary of human PFAS exposure pathways. Human exposure to PFAS may occur through multiple pathways including food, consumer products, food packaging, cleaning products, personal care products, household dust, and contact with a variety of other contaminated media. While dietary intake resulting from the ingestion of contaminated drinking water and/or foods grown in contaminated groundwater rank among the key exposure routes for the general population, emerging evidence indicates that plastic containers, such as those used for storage of food, drinks, personal care products, pharmaceuticals, and cleaning and industrial products, may represent a major new PFAS exposure source. Figure adapted from European Environment Agency – Emerging chemical risks in Europe – ‘PFAS’ (2019).
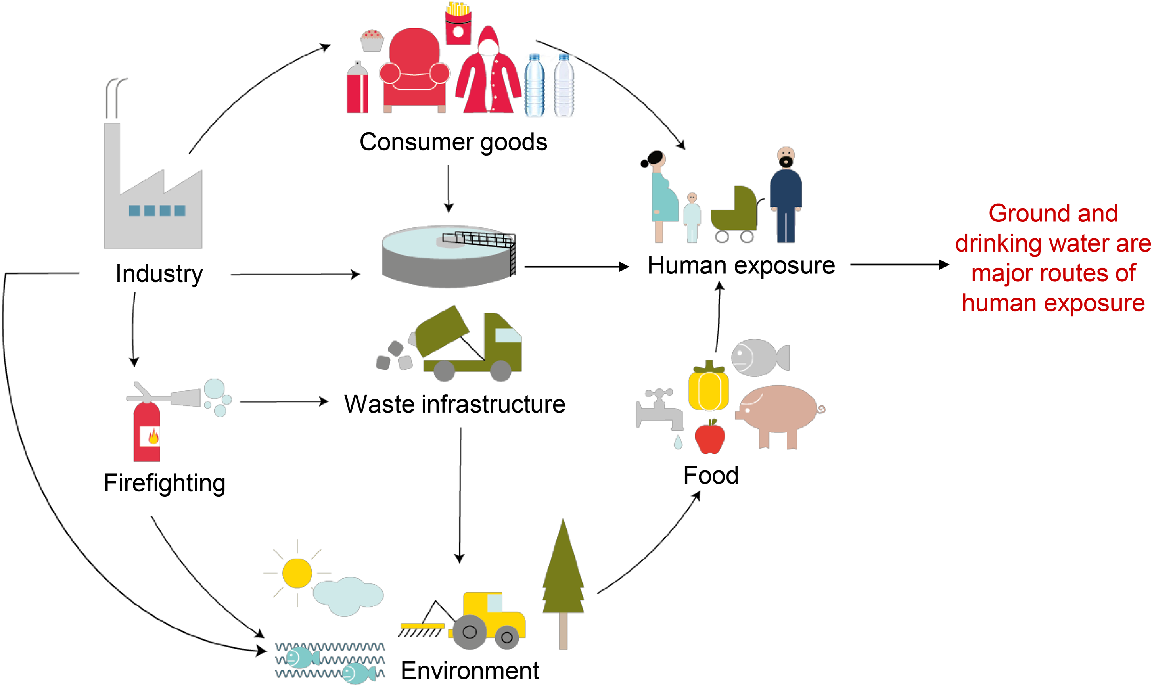
If the preliminary site investigation and conceptual site model shows the contaminated site presents an exposure risk to people or the environment, then a detailed site assessment and treatment is required (CRC CARE 2018). To date, a wide array of PFAS treatment technologies have been applied to treat contaminated soil and water. Overall, these strategies can be divided into destructive and non-destructive technologies that can be applied either in situ or ex situ to immobilise, separate, or more rarely, destroy PFAS at a site (CRC CARE 2018). The proving and scaling up of technologies for soil and water remediation remains an area of extensive research. In a field study, a soil washing technique was able to remove up to 96% of PFOS from soil (Swedish EPA 2018), while others demonstrated the remediation of 1000 tonnes of PFAS-contaminated soil by stabilising PFAS in soil using a mixed mode sorbent material (Swedish EPA 2018). Other non-destructive techniques, such as phytoremediation, and destructive techniques, such as thermal oxidation and chemical oxidation, have been applied at field scale, but with varying efficacy (Bolan et al. 2021). For contaminated water sources, activated carbon, ion exchange resins, and reverse osmosis have been used widely and at scale to remove PFAS (Bolan et al. 2021). However, there remains a considerable mismatch between the scale and breadth of PFAS contamination, and the applicability and cost-effectiveness of the tools required to resolve this pollution. For this reason, achieving large scale/throughput and cost-effectiveness with short-term measures, such as landfilling contaminated soil, will be key benchmarks for new technologies.
In the last two decades, the life cycle regulation of PFAS has improved, particularly with the discontinued manufacture of the most demonstrably harmful PFAS species. These regulatory changes have had the dual effect of somewhat reducing the influx of PFAS into the environment and raising the standard of treatment of water and soil required, thereby incentivising further innovation in remediation technology. However, decades of PFAS use without regulation or monitoring mean that regardless of future inputs, the volumes of water and soil requiring clean-up are enormous and globally distributed, thus demanding a correspondingly concerted response.
Impacts of PFAS on human health
Recent changes to the US EPA health advisory concerning PFAS consumption come in the wake of the Scientific Committee on Health labelling PFAS as a potential risk for humans and the environment (Scientific Committee on Health, Environmental and Emerging Risks (SCHEER) 2018). Such advisories reflect increasing awareness of the propensity of PFAS to accumulate in human tissues combined with mounting epidemiological evidence supporting negative associations between PFAS exposure and an array of human health conditions (Calvert et al. 2021). Despite endeavours to phase out the so-called long-chain PFAS, the inherent stability of these compounds has resulted in omnipresence in the global environment. Thus, as discussed later, many industrialised nations are seeking to implement additional measures to limit, detect, and eradicate PFAS contamination. Although all paths of human exposure remain to be identified, it is thought that dietary intake from food packaging or environmental contamination remains a key exposure pathway for the general population (Xing et al. 2023; Zhu et al. 2023) (Fig. 2). In this context, recent evidence suggests that plastic containers, such as those used for storage of food, drinks, personal care products, pharmaceuticals, and cleaning and industrial products, may represent a major new exposure point (Landrigan et al. 2023) (Fig. 2). Such contamination has been linked to the use of PFAS as lubricants during the plastic manufacturing process as well as from the practice of fluorination, whereby plastic containers are treated with fluorine gas to improve their stability and reduce their permeability. This creates the potential for several troubling scenarios, including the contamination of plastic recycling streams and unintentional leeching of PFAS into the consumer product.
Irrespective of the route of exposure, upon entering the body, PFAS bind to proteins in the blood stream and thereafter accumulate within the body’s protein-rich tissues (Jensen and Leffers 2008; Perez et al. 2013). Consequently, PFAS are readily detectable in most bodily fluids (including urine, breast milk, blood, and seminal plasma) as well as throughout the human body and may take several years to be fully excreted (Calafat et al. 2007; Jian et al. 2018). Despite this knowledge, it has proven challenging to definitively link PFAS exposure to impacts on human health owing to factors such as variations in chemistries and potential biological activities among the different sub-classes of PFAS, the duration and degree of exposure, and potential synergistic or antagonistic effects of PFAS combinations in the body (Rand and Mabury 2017). This situation is further compounded by differences in the mechanisms and routes of PFAS exposure as well as the genetic constitution of exposed individuals, the latter of which may influence PFAS clearance rates and susceptibility to the biological effects of these chemicals.
Notwithstanding these limitations, the balance of evidence supports the potential for PFAS exposure to elicit adverse health sequelae across the life course (Kirk et al. 2018). This conclusion is also borne out by the findings of the C8 Health Project; a comprehensive investigation of an entire community of more than 69,000 people exposed to PFAS via consumption of contaminated drinking water (Frisbee et al. 2009). This study revealed probable links between PFOA exposure and six diseases: kidney and testicular cancer, thyroid disease, high cholesterol, ulcerative colitis, and pregnancy-induced hypertension. Building on this evidence, the most consistently reported metabolic consequence of PFAS exposure is dyslipidemia, with several notable studies finding links between serum PFAS and dysregulated lipid profiles, including increased low-density lipoprotein, triglycerides, and total cholesterol, in addition to diminished high-density lipoprotein (Olsen and Zobel 2007; Sakr et al. 2007a, 2007b; Costa et al. 2009; Frisbee et al. 2009).
Epidemiological evidence has also linked PFAS exposure to the prevalence of testicular cancer, with the International Agency for Research on Cancer (IARC) concluding PFOA is possibly carcinogenic to humans (IARC 2023) and the US EPA declaring it a likely carcinogen (United States Environmental Protection Agency (US EPA) 2021, 2022). The potential significance of these positive associations is highlighted by parallel correlations between the widespread increase in worldwide PFAS usage and the rising prevalence of testicular cancer; a pathology that has significantly increased in recent times to become the most common malignancy in young men aged 20–40 years (Skakkebaek et al. 2007; McIver et al. 2013). Although the characterisation of testicular cancer remains incomplete, there is speculation that environmental factors, as opposed to genetic factors, are a key contributor to the aetiology of this form of cancer. Further evidence of testicular dysfunction is supported by large cohort studies, which have revealed associations between PFAS exposure and several indicators of human sperm quality, including reductions in total sperm count, normal sperm morphology, and sperm motility (Joensen et al. 2009; Vested et al. 2013; Governini et al. 2015; Louis et al. 2015; Song et al. 2018). PFAS have also been linked a variety of additional reproductive characteristics, including direct disturbance of testicular steroidogenic cells and an attendant dysregulation of reproductive hormone profiles (Olsen et al. 1998; Zhang et al. 2014; Cui et al. 2020). Taken together, such correlations raise the prospect that the testis is a vulnerable organ for PFAS-induced damage.
These findings highlight the requirement for further investigation and the identification of reliable biological models that can inform health risks, allowing sensitive assessment of the spectrum of effects of PFAS exposure on humans. In this regard, there is clearly much to learn from the study of pre-clinical animal models, wherein PFAS exposure can be controlled to mitigate many of the confounders that continue to plague the study of human cohorts.
Impacts of PFAS on animals
Exposure to high levels of PFAS poses a health risk not just for humans but for domestic animals and wildlife. Understanding the abundance, distribution, and effects of PFAS on livestock and wildlife species can help identify any adverse impacts on top of other anthropogenic-created issues faced by animal species on their health, population dynamics, and habitat. Notably, PFAS exposure is another anthropogenic factor thought to drive population decline and extinction (Kannan et al. 2006; Ishibashi et al. 2008).
In terms of habitat and the exposure of wildlife to PFAS, it is well documented that the long-chain (≥8 carbons) PFAS, such as PFOA and PFOS, as well as shorter chain PFAS are transported long distances to remote environments via air (Zhou et al. 2022) and water currents (De Silva et al. 2021). Atmospheric pathways are particularly important as many PFAS are water soluble, so are highly persistent in surface and groundwater (Szabo et al. 2018; Zhao et al. 2020; Szabo et al. 2023), as well as oceans (Gonzalez-Gaya et al. 2014). Hence, transport by ocean currents is thought to be the main pathway for the global distribution of PFOS and PFOA, with PFAS commonly being redistributed from lower latitudes to the poles (Yamashita et al. 2008; Armitage et al. 2009a, 2009b; Stemmler and Lammel 2010). Loss of PFAS from the water column through settling and mixing to the deep waters of the ocean is estimated to remove approximately 25% of the total global PFOA emissions (Armitage et al. 2009a), resulting in PFAS being present and having the ability to circulate in the world’s oceans for many centuries after their production ends. Despite a large percentage of PFOA and PFOS entering the sediment, concentrations in the ocean are known to vary from 57 to 200 ng/L in coastal waters around cities to 1–15 pg/L in the central Pacific Ocean (Yamashita et al. 2005).
Detection of PFAS in the oceans and waterways unsurprisingly has resulted in their uptake by wildlife (Letcher et al. 2010). PFAS in wildlife was first reported by Giesy and Kannan (2001) who demonstrated the global distribution of PFOS in fish and birds, as well as in marine and terrestrial mammals. In addition, that PFOS concentrations in animals from relatively more populated and industrialised regions, such as the North American Great Lakes, Baltic Sea, and Mediterranean Sea, were generally greater than those in animals from remote locations. Equally, temporal trends in the concentration of various PFAS are evident (Martin et al. 2004; Houde et al. 2011), which along with diet, feeding behaviour and habitat, as well as trophic order and metabolic rate all contribute to variation in PFAS profiles and concentrations in wildlife (Lein 1972; Hobson and Welch 1992; Robuck et al. 2020). Despite this, PFOS remains the predominant PFAS found in all wildlife species, tissues, and locations analysed around the world (Houde et al. 2006; Houde et al. 2011). It stands to reason that if PFAS are in the environment and detectable in wildlife and in humans, they will also be present in livestock, potentially affecting their health and reproduction. This troubling scenario has been confirmed whereby the migration of PFAS onto agricultural properties has been documented to result in accumulation in livestock, also likely contributing to the impact on humans through the food chain (Death et al. 2021; Drew et al. 2021; Jha et al. 2021; Mikkonen et al. 2023).
Indeed, movement of PFAS through food webs and the bioaccumulation potential of PFOA and other long-chain PFAS (≥8 carbons), especially in animals with a high body fat content, is evident and of great concern (Kelly et al. 2009; Ahrens 2011; Houde et al. 2011). Consequently, apex predators, such as killer whales, polar bears, and bald eagles, harbour PFAS concentrations higher than those measured in their diet (Smithwick et al. 2006; Kelly et al. 2009; Ahrens 2011; Lindstrom et al. 2011), indicating the bioaccumulation and biomagnification of PFAS in food webs (Giesy and Kannan 2001; Kannan et al. 2005). Species’ differences in the concentration and ability to bioaccumulate specific PFAS congeners are apparent. For example, PFOS concentrations are generally lower, longer-chain perfluoroalkyl carboxylic acids (PFCA) concentrations are greater, and short-chain PFCA are more commonly detected in birds than in marine mammals (Letcher et al. 2010; Muir et al. 2019). In addition, within an animal the distribution of PFAS in body fluids and tissues is congener-specific (Gebbink and Letcher 2012), underpinned by PFAS interactions with proteins (Bangma et al. 2022). Increasing body burden with age and differences between sexes are also evident (Bangma et al. 2022), especially for egg-laying species, due to associations of PFAS with lipids and proteins in the yolk; hence, this is a major excretion route for PFAS in females (Newsted et al. 2007; Tartu et al. 2014; Lopez-Antia et al. 2019). The high PFAS concentration in the egg is thus likely to impact in ovo fetal growth and potentially long-term health of that offspring. Equally, species that consume eggs, including humans, risk ingesting highly concentrated PFAS in their diet. Further well-documented maternal PFAS transfer routes include via in utero gestation (Ma et al. 2022) and lactation (Gronnestad et al. 2017), as evidenced by high PFAS concentration in young and juveniles (Ishibashi et al. 2008). Collectively, these studies highlight how reproduction, food webs, and agricultural practices can exacerbate PFAS intake and their effects on subsequent generations.
Once PFAS is in the body, adverse effects to the genealogical, neurological, and endocrinological systems of wildlife species are reported (Dietz et al. 2019; Bangma et al. 2022), resulting in potential detrimental immunotoxicological, histopathological, and reproductive effects (Kannan et al. 2006; Letcher et al. 2010; Dietz et al. 2019; Bangma et al. 2022). This is supported by studies of laboratory animals showing associations between exposure to PFAS and adverse health and reproductive effects across generations (Kato et al. 2015; Chen et al. 2017; Haimbaugh et al. 2022). Attribution of these impacts to a single PFAS or the total PFAS burden is unclear, because most wildlife studies are based on association and observation, with limitations in accurate quantification and ability to measure all PFAS congeners. To comprehensively summarise the current data and our understanding of PFAS exposure effects on wildlife, novel systematic evidence maps and bibliometric analyses are being developed (Vendl et al. 2021). However, there is still clearly an urgent need for studies to identify PFAS exposure and impacts across wildlife species. Alongside this, the development of better non-invasive sampling methodologies is required to minimise sampling stress on wildlife. Identifying the effect of PFAS on wildlife is therefore fundamental in helping to regulate and legislate their use, not to mention how consumption of wildlife and domesticated animals by humans is a considerable exposure route (Fig. 2).
Social impacts and health policies
As documented above, there is mounting evidence that PFAS contamination has the potential to harm ecosystem health as well as human health and reproduction. In this capacity, PFAS may already be contributing to the biodiversity crisis, climate change, and degradation of fundamental ecosystem services including food and water supplies. PFAS pollution is therefore a public health issue globally, with clearly traceable physical health outcomes (as forementioned), as well as psychosocial impacts for those living in impacted areas (Banwell et al. 2021). There is now compelling evidence from social and psychological science that fear of and anxiety about uncertain futures posed by planetary health disasters, such as chemical pollution, is driving new mental health symptomology such as ‘eco-anxiety’ and ‘ecological grief’ (Patrick et al. 2023a, 2023b). For young people, anxiety about the future of the planet is causing functional and cognitive impairment at levels which are affecting their work, family, and social lives (Patrick et al. 2023a, 2023a). Indeed, planetary health concerns are now factoring into young peoples’ reproductive choices in many countries (Schneider-Mayerson and Leong 2020). Conversely, taking action on planetary health issues can promote mental and social wellbeing and enhance environmental stewardship (Gunasiri et al. 2022).
An urgent and concerted global effort is needed to the respond to the complex drivers of these planetary health issues, including those arising from chemical pollution. In the Australian context, current actions to promote human health and protect ecosystems include:
The PFAS policy solutions: The Australian PFAS National Environmental Management Plan (PFAS NEMP) 2020 provides nationally agreed guidance on the management of PFAS contamination in the environment, including prevention of the spread of contamination (HEPA 2020). It supports collaborative action on PFAS by the Commonwealth, state and territory and local governments around Australia.
The Climate and Health Policy solutions: A coalition of leading Australian health experts and organisations, along with federal parliamentarians, have launched a Framework for a National Strategy on Climate, Health and Well-being for Australia (Climate and Health Alliance 2021). This framework for a National Strategy on Climate, Health and Well-being for Australia provides a roadmap to support the Commonwealth Government in taking a leadership role in protecting the health and wellbeing of Australian communities from climate change, and in fulfilling its international obligations under the Paris Agreement.
The circular economy and plastics solutions: A World Health Organization circular economy approach offers an avenue to sustainable economy and good health, whilst saving the environment and its natural resources (WHO 2018). Key elements of a circular economy approach for plastics include: eliminating of problematic/unnecessary plastic packaging through redesign; reuse where relevant, reducing the need for single-use plastics; ensuring all plastic packaging is 100% reusable, recyclable, or compostable; plastic is decoupled from the consumption of finite natural resources; and plastic packaging is free of hazardous chemicals ensuring the health and safety of all involved in the supply chain (Ellen MacArthur Foundation 2022).
Scientists and plastics leadership: The Commonwealth Scientific and Industrial Research Organisation (CSIRO) in Australia has set a target of an 80% reduction in plastic waste entering the Australian environment by 2030 (CSIRO 2023). Strategies include human behaviour change and incentives, waste innovation, and developing best practice and standards.
Summary and future considerations
Gradually, institutions are moving to phase out the use of PFAS. In Australia, an action plan to voluntarily phase out all PFAS from fibre-based food packaging is underway (APCO 2023). However, the Australian Government is still working to align with the Stockholm Convention, which would subject PFAS to enhanced regulation including restriction, waste management, and elimination (Department of Climate Change, Energy, the Environment and Water (DCCEEW) 2023). While limiting new sources of PFAS exposure is a positive step, remediating already contaminated environments will be challenging due to the complex interactions between these chemicals and the environment. As such, there is an urgent need to understand the impacts of PFAS on reproductive function in humans, domestic species, and wildlife.
Collaborative efforts are needed to minimise the adverse effects of PFAS on the environment and reproductive health. Such efforts include monitoring the effects of compounds like PFAS and designing methods to eliminate or manage their presence in the environment. Importantly, scientists have an important role to play in terms of acting as advocates and effectively educating the public and policy makers on the complexity of the issues surrounding persistent compounds like PFAS and the potential consequences to the health of all species.
Data availability
This is a Viewpoint article in which all relevant data are available in the cited publications.
References
Ahrens L (2011) Polyfluoroalkyl compounds in the aquatic environment: a review of their occurrence and fate. Journal of Environmental Monitoring 13, 20-31.
| Crossref | Google Scholar | PubMed |
Andrews DQ, Naidenko OV (2020) Population-wide exposure to per- and polyfluoroalkyl substances from drinking water in the United States. Environmental Science & Technology Letters 7, 931-936.
| Crossref | Google Scholar |
APCO (2023) Action plan to phase out PFAS in fibre-based food contact packaging. Australian Packaging Covenant Organisation, Sydney, Australia. Available at https://documents.packagingcovenant.org.au/public-documents/Action%20Plan%20to%20Phase%20Out%20PFAS%20in%20Fibre-Based%20Food%20Contact%20Packaging [Accessed February 2024]
Armitage JM, MacLeod M, Cousins IT (2009a) Modeling the global fate and transport of perfluorooctanoic acid (PFOA) and perfluorooctanoate (PFO) emitted from direct sources using a multispecies mass balance model. Environmental Science & Technology 43, 1134-1140.
| Crossref | Google Scholar | PubMed |
Armitage JM, Schenker U, Scheringer M, Martin JW, MacLeod M, Cousins IT (2009b) Modeling the global fate and transport of perfluorooctane sulfonate (PFOS) and precursor compounds in relation to temporal trends in wildlife exposure. Environmental Science & Technology 43, 9274-9280.
| Crossref | Google Scholar | PubMed |
Aulsebrook LC, Bertram MG, Martin JM, Aulsebrook AE, Brodin T, Evans JP, Hall MD, O’Bryan MK, Pask AJ, Tyler CR, Wong BBM (2020) Reproduction in a polluted world: implications for wildlife. Reproduction 160, R13-R23.
| Crossref | Google Scholar | PubMed |
Australian Associated Press (2023) Australian government reaches $132.7m class action settlement with landowners over PFAS contamination. The Guardian Sydney, NSW, Australia. Available at https://www.theguardian.com/environment/2023/may/15/australian-government-reaches-1327m-class-action-settlement-with-landowners-over-pfas-contamination [Accessed February 2024].
| Google Scholar |
Bangma J, Guillette TC, Bommarito PA, Ng C, Reiner JL, Lindstrom AB, Strynar MJ (2022) Understanding the dynamics of physiological changes, protein expression, and PFAS in wildlife. Environment International 159, 107037.
| Crossref | Google Scholar | PubMed |
Banwell C, Housen T, Smurthwaite K, Trevenar S, Walker L, Todd K, Rosas M, Kirk M (2021) Health and social concerns about living in three communities affected by per- and polyfluoroalkyl substances (PFAS): a qualitative study in Australia. PLoS ONE 16, e0245141.
| Crossref | Google Scholar | PubMed |
Bao J, Yu W-J, Liu Y, Wang X, Jin Y-H, Dong G-H (2019) Perfluoroalkyl substances in groundwater and home-produced vegetables and eggs around a fluorochemical industrial park in China. Ecotoxicology and Environmental Safety 171, 199-205.
| Crossref | Google Scholar | PubMed |
Bolan N, Sarkar B, Yan Y, Li Q, Wijesekara H, Kannan K, Tsang DCW, Schauerte M, Bosch J, Noll H, Ok YS, Scheckel K, Kumpiene J, Gobindlal K, Kah M, Sperry J, Kirkham MB, Wang H, Tsang YF, Hou D, Rinklebe J (2021) Remediation of poly- and perfluoroalkyl substances (PFAS) contaminated soils – to mobilize or to immobilize or to degrade? Journal of Hazardous Materials 401, 123892.
| Crossref | Google Scholar | PubMed |
Brown JB, Conder JM, Arblaster JA, Higgins CP (2020) Assessing human health risks from per- and polyfluoroalkyl substance (PFAS)-impacted vegetable consumption: a tiered modeling approach. Environmental Science & Technology 54, 15202-15214.
| Crossref | Google Scholar | PubMed |
Brusseau ML, Anderson RH, Guo B (2020) PFAS concentrations in soils: background levels versus contaminated sites. Science of The Total Environment 740, 140017.
| Crossref | Google Scholar | PubMed |
Buck RC, Franklin J, Berger U, Conder JM, Cousins IT, de Voogt P, Jensen AA, Kannan K, Mabury SA, van Leeuwen SPJ (2011) Perfluoroalkyl and polyfluoroalkyl substances in the environment: terminology, classification, and origins. Integrated Environmental Assessment and Management 7, 513-541.
| Crossref | Google Scholar | PubMed |
Calafat AM, Wong L-Y, Kuklenyik Z, Reidy JA, Needham LL (2007) Polyfluoroalkyl chemicals in the U.S. population: data from the National Health and Nutrition Examination Survey (NHANES) 2003-2004 and comparisons with NHANES 1999-2000. Environmental Health Perspectives 115, 1596-1602.
| Crossref | Google Scholar | PubMed |
Calvert L, Green MP, De Iuliis GN, Dun MD, Turner BD, Clarke BO, Eamens AL, Roman SD, Nixon B (2021) Assessment of the emerging threat posed by perfluoroalkyl and polyfluoroalkyl substances to male reproduction in humans. Frontiers in Endocrinology 12, 799043.
| Crossref | Google Scholar |
Chen Y, Zhou L, Xu J, Zhang L, Li M, Xie X, Xie Y, Luo D, Zhang D, Yu X, Yang B, Kuang H (2017) Maternal exposure to perfluorooctanoic acid inhibits luteal function via oxidative stress and apoptosis in pregnant mice. Reproductive Toxicology 69, 159-166.
| Crossref | Google Scholar | PubMed |
Chow SJ, Ojeda N, Jacangelo JG, Schwab KJ (2021) Detection of ultrashort-chain and other per- and polyfluoroalkyl substances (PFAS) in U.S. bottled water. Water Research 201, 117292.
| Crossref | Google Scholar | PubMed |
Climate and Health Alliance (2021) Healthy, regenerative and just: framework for a national strategy on climate, health and well-being for Australia. (CAHA: Melbourne, Australia) Available at https://d3n8a8pro7vhmx.cloudfront.net/caha/pages/1947/attachments/original/1639623234/caha-framework-2.0-FA.pdf?1639623234 [Accessed February 2024]
Costa G, Sartori S, Consonni D (2009) Thirty years of medical surveillance in perfluooctanoic acid production workers. Journal of Occupational & Environmental Medicine 51, 364-372.
| Crossref | Google Scholar | PubMed |
CRC CARE (2018) Practitioner guide to risk-based assessment, remediation and management of PFAS site contamination. CRC CARE Technical Report no. 43. (CRC for Contamination Assessment and Remediation of the Environment, Newcastle University: Newcastle, Australia) Available at https://www.remediationframework.com.au/national-remediation-framework/toolbox/pfas/30-practitioner-guide-assessment-management-and-remediation-of-pfas/file [Accessed February 2024]
CSIRO (2023) Tackling plastic waste. Commonwealth Scientific and Industrial Research Organisation. Available at https://www.csiro.au/en/research/environmental-impacts/recycling/Plastics [Accessed February 2024]
Cui Q, Pan Y, Wang J, Liu H, Yao B, Dai J (2020) Exposure to per- and polyfluoroalkyl substances (PFASs) in serum versus semen and their association with male reproductive hormones. Environmental Pollution 266, 115330.
| Crossref | Google Scholar | PubMed |
De Silva AO, Armitage JM, Bruton TA, Dassuncao C, Heiger-Bernays W, Hu XC, Karrman A, Kelly B, Ng C, Robuck A, Sun M, Webster TF, Sunderland EM (2021) PFAS exposure pathways for humans and wildlife: a synthesis of current knowledge and key gaps in understanding. Environmental Toxicology and Chemistry 40, 631-657.
| Crossref | Google Scholar | PubMed |
de Vries FT, Wallenstein MD (2017) Below-ground connections underlying above-ground food production: a framework for optimising ecological connections in the rhizosphere. Journal of Ecology 105, 913-920.
| Crossref | Google Scholar |
Death C, Bell C, Champness D, Milne C, Reichman S, Hagen T (2021) Per- and polyfluoroalkyl substances (PFAS) in livestock and game species: a review. Science of The Total Environment 774, 144795.
| Crossref | Google Scholar | PubMed |
Department of Climate Change, Energy, the Environment and Water (DCCEEW) (2023) Per- and poly-fluoroalkyl substances (PFASs). Australian Government, Canberra, Australia. Available at https://www.dcceew.gov.au/environment/protection/chemicals-management/pfas [Accessed February 2024]
Dietz R, Letcher RJ, Desforges J-P, Eulaers I, Sonne C, Wilson S, Andersen-Ranberg E, Basu N, Barst BD, Bustnes JO, Bytingsvik J, Ciesielski TM, Drevnick PE, Gabrielsen GW, Haarr A, Hylland K, Jenssen BM, Levin M, McKinney MA, Nørregaard RD, Pedersen KE, Provencher J, Styrishave B, Tartu S, Aars J, Ackerman JT, Rosing-Asvid A, Barrett R, Bignert A, Born EW, Branigan M, Braune B, Bryan CE, Dam M, Eagles-Smith CA, Evans M, Evans TJ, Fisk AT, Gamberg M, Gustavson K, Hartman CA, Helander B, Herzog MP, Hoekstra PF, Houde M, Hoydal K, Jackson AK, Kucklick J, Lie E, Loseto L, Mallory ML, Miljeteig C, Mosbech A, Muir DCG, Nielsen ST, Peacock E, Pedro S, Peterson SH, Polder A, Rigét FF, Roach P, Saunes H, Sinding M-HS, Skaare JU, Søndergaard J, Stenson G, Stern G, Treu G, Schuur SS, Víkingsson G (2019) Current state of knowledge on biological effects from contaminants on arctic wildlife and fish. Science of The Total Environment 696, 133792.
| Crossref | Google Scholar |
Drew R, Hagen TG, Champness D (2021) Accumulation of PFAS by livestock – determination of transfer factors from water to serum for cattle and sheep in Australia. Food Additives & Contaminants: Part A 38, 1897-1913.
| Crossref | Google Scholar | PubMed |
EFSA Panel on Contaminants in the Food Chain (EFSA CONTAM), Schrenk D, Bignami M, Bodin L, Chipman JK, Del Mazo J, Grasl-Kraupp B, Hogstrand C, Hoogenboom LR, Leblanc J-C, Nebbia CS, Nielsen E, Ntzani E, Petersen A, Sand S, Vleminckx C, Wallace H, Barregard L, Ceccatelli S, Cravedi J-P, Halldorsson TI, Haug LS, Johansson N, Knutsen HK, Rose M, Roudot A-C, Van Loveren H, Vollmer G, Mackay K, Riolo F, Schwerdtle T (2020) Risk to human health related to the presence of perfluoroalkyl substances in food. EFSA Journal 18, e06223.
| Crossref | Google Scholar |
Ellen MacArthur Foundation (2022) Plastics and the circular economy – deep dive. Ellen MacArthur Foundation, Isle of Wight, UK. Available at https://www.ellenmacarthurfoundation.org/plastics-and-the-circular-economy-deep-dive [Accessed February 2024]
European Environment Agency – Emerging chemical risks in Europe – ‘PFAS’ (2019) Briefing no. 12/2019. doi:10.2800/48621. Available at https://www.eea.europa.eu/publications/emerging-chemical-risks-in-europe/emerging-chemical-risks-in-europe
Friedman L, Giang V (2023) 3M reaches $10.3 billion settlement in ‘forever chemicals’ suits. The New York Times New York, USA. Available at https://www.nytimes.com/2023/06/22/business/3m-settlement-forever-chemicals-lawsuit.html [Accessed February 2024].
| Google Scholar |
Frisbee SJ, Brooks AP, Jr, Maher A, Flensborg P, Arnold S, Fletcher T, Steenland K, Shankar A, Knox SS, Pollard C, Halverson JA, Vieira VM, Jin C, Leyden KM, Ducatman AM (2009) The C8 health project: design, methods, and participants. Environmental Health Perspectives 117, 1873-1882.
| Crossref | Google Scholar | PubMed |
Gebbink WA, Letcher RJ (2012) Comparative tissue and body compartment accumulation and maternal transfer to eggs of perfluoroalkyl sulfonates and carboxylates in Great Lakes herring gulls. Environmental Pollution 162, 40-47.
| Crossref | Google Scholar | PubMed |
Giesy JP, Kannan K (2001) Global distribution of perfluorooctane sulfonate in wildlife. Environmental Science & Technology 35, 1339-1342.
| Crossref | Google Scholar | PubMed |
Gonzalez-Gaya B, Dachs J, Roscales JL, Caballero G, Jimenez B (2014) Perfluoroalkylated substances in the global tropical and subtropical surface oceans. Environmental Science & Technology 48, 13076-13084.
| Crossref | Google Scholar | PubMed |
Governini L, Guerranti C, De Leo V, Boschi L, Luddi A, Gori M, Orvieto R, Piomboni P (2015) Chromosomal aneuploidies and DNA fragmentation of human spermatozoa from patients exposed to perfluorinated compounds. Andrologia 47, 1012-1019.
| Crossref | Google Scholar | PubMed |
Gronnestad R, Villanger GD, Polder A, Kovacs KM, Lydersen C, Jenssen BM, Borga K (2017) Maternal transfer of perfluoroalkyl substances in hooded seals. Environmental Toxicology and Chemistry 36, 763-770.
| Crossref | Google Scholar | PubMed |
Gunasiri H, Wang Y, Watkins EM, Capetola T, Henderson-Wilson C, Patrick R (2022) Hope, coping and eco-anxiety: young people’s mental health in a climate-impacted Australia. International Journal of Environmental Research and Public Health 19, 5528.
| Crossref | Google Scholar |
Guruge KS, Manage PM, Yamanaka N, Miyazaki S, Taniyasu S, Yamashita N (2008) Species-specific concentrations of perfluoroalkyl contaminants in farm and pet animals in Japan. Chemosphere 73, S210-S215.
| Crossref | Google Scholar | PubMed |
Haimbaugh A, Wu C-C, Akemann C, Meyer DN, Connell M, Abdi M, Khalaf A, Johnson D, Baker TR (2022) Multi- and transgenerational effects of developmental exposure to environmental levels of PFAS and PFAS mixture in Zebrafish (Danio rerio). Toxics 10, 334.
| Crossref | Google Scholar |
Hansen KJ, Clemen LA, Ellefson ME, Johnson HO (2001) Compound-specific, quantitative characterization of organic fluorochemicals in biological matrices. Environmental Science & Technology 35, 766-770.
| Crossref | Google Scholar | PubMed |
HEPA (2020) PFAS National Environmental Management Plan (PFAS NEMP), Version 2.0. Heads of Environmental Protection Agencies Australia and New Zealand, Canberra, Australia. Available at https://www.dcceew.gov.au/sites/default/files/documents/pfas-nemp-2.pdf [Accessed February 2024]
Hepburn E, Madden C, Szabo D, Coggan TL, Clarke B, Currell M (2019) Contamination of groundwater with per- and polyfluoroalkyl substances (PFAS) from legacy landfills in an urban re-development precinct. Environmental Pollution 248, 101-113.
| Crossref | Google Scholar | PubMed |
Herzke D, Huber S, Bervoets L, D’Hollander W, Hajslova J, Pulkrabova J, Brambilla G, De Filippis SP, Klenow S, Heinemeyer G, de Voogt P (2013) Perfluorinated alkylated substances in vegetables collected in four European countries; occurrence and human exposure estimations. Environmental Science and Pollution Research International 20, 7930-7939.
| Crossref | Google Scholar | PubMed |
Hobson KA, Welch HE (1992) Determination of trophic relationships within a high Arctic marine food web using δ13C and δ15N analysis. Marine Ecology Progress Series 84, 9-18.
| Crossref | Google Scholar |
Houde M, Martin JW, Letcher RJ, Solomon KR, Muir DCG (2006) Biological monitoring of polyfluoroalkyl substances: a review. Environmental Science & Technology 40, 3463-3473.
| Crossref | Google Scholar | PubMed |
Houde M, De Silva AO, Muir DCG, Letcher RJ (2011) Monitoring of perfluorinated compounds in aquatic biota: an updated review. Environmental Science & Technology 45, 7962-7973.
| Crossref | Google Scholar | PubMed |
IARC (2023) Evaluate the carcinogenicity of perfluorooctanoic acid (PFOA) and perfluorooctanesulfonic acid (PFOS). IARC Monographs. International Agency for Resesearch on Cancer, Lyon, France. Available at https://www.iarc.who.int/news-events/iarc-monographs-evaluate-the-carcinogenicity-of-perfluorooctanoic-acid-pfoa-and-perfluorooctanesulfonic-acid-pfos/ [Accessed February 2024]
Interstate Technology and Regulatory Council (ITRC) (2023) Environmental fate and transport for per- and polyfluoroalkyl substances. PFAS Technical and Regulatory Guidance Document and Fact Sheets. Washington, DC, USA. Available at https://pfas-1.itrcweb.org/ [Accessed February 2024]
Ishibashi H, Iwata H, Kim E-Y, Tao L, Kannan K, Tanabe S, Batoev VB, Petrov EA (2008) Contamination and effects of perfluorochemicals in Baikal seal (Pusa sibirica). 2. Molecular characterization, expression level, and transcriptional activation of peroxisome proliferator-activated receptor alpha. Environmental Science & Technology 42, 2302-2308.
| Crossref | Google Scholar | PubMed |
Jensen AA, Leffers H (2008) Emerging endocrine disrupters: perfluoroalkylated substances. International Journal of Andrology 31, 161-169.
| Crossref | Google Scholar | PubMed |
Jha G, Kankarla V, McLennon E, Pal S, Sihi D, Dari B, Diaz D, Nocco M (2021) Per- and Polyfluoroalkyl Substances (PFAS) in integrated crop-livestock systems: environmental exposure and human health risks. International Journal of Environmental Research and Public Health 18, 12550.
| Crossref | Google Scholar |
Jian J-M, Chen D, Han F-J, Guo Y, Zeng L, Lu X, Wang F (2018) A short review on human exposure to and tissue distribution of per- and polyfluoroalkyl substances (PFASs). Science of The Total Environment 636, 1058-1069.
| Crossref | Google Scholar | PubMed |
Joensen UN, Bossi R, Leffers H, Jensen AA, Skakkebaek NE, Jorgensen N (2009) Do perfluoroalkyl compounds impair human semen quality? Environmental Health Perspectives 117, 923-927.
| Crossref | Google Scholar | PubMed |
Kabiri S, Tucker W, Navarro DA, Braunig J, Thompson K, Knight ER, Nguyen TMH, Grimison C, Barnes CM, Higgins CP, Mueller JF, Kookana RS, McLaughlin MJ (2022) Comparing the leaching behavior of per- and polyfluoroalkyl substances from contaminated soils using static and column leaching tests. Environmental Science & Technology 56, 368-378.
| Crossref | Google Scholar | PubMed |
Kannan K, Tao L, Sinclair E, Pastva SD, Jude DJ, Giesy JP (2005) Perfluorinated compounds in aquatic organisms at various trophic levels in a Great Lakes food chain. Archives of Environmental Contamination and Toxicology 48, 559-566.
| Crossref | Google Scholar | PubMed |
Kannan K, Perrotta E, Thomas NJ (2006) Association between perfluorinated compounds and pathological conditions in southern sea otters. Environmental Science & Technology 40, 4943-4948.
| Crossref | Google Scholar | PubMed |
Kato H, Fujii S, Takahashi M, Matsumoto M, Hirata-Koizumi M, Ono A, Hirose A (2015) Repeated dose and reproductive/developmental toxicity of perfluorododecanoic acid in rats. Environmental Toxicology 30, 1244-1263.
| Crossref | Google Scholar |
Kelly BC, Ikonomou MG, Blair JD, Surridge B, Hoover D, Grace R, Gobas FAPC (2009) Perfluoroalkyl contaminants in an Arctic marine food web: trophic magnification and wildlife exposure. Environmental Science & Technology 43, 4037-4043.
| Crossref | Google Scholar |
Kirk M, Smurthwaite K, Bräunig J, Trevenar S, D’Este C, Lucas R, Lal A, Korda R, Clements A, Mueller J, Armstrong B (2018) The PFAS health study: systematic literature review. pp. 1–256. (The Australian National University: Canberra, Australia). Available at https://nceph.anu.edu.au/files/PFAS%20Health%20Study%20Systematic%20Review_1.pdf
Kurwadkar S, Dane J, Kanel SR, Nadagouda MN, Cawdrey RW, Ambade B, Struckhoff GC, Wilkin R (2022) Per- and polyfluoroalkyl substances in water and wastewater: a critical review of their global occurrence and distribution. Science of The Total Environment 809, 151003.
| Crossref | Google Scholar | PubMed |
Landrigan PJ, Raps H, Cropper M, Bald C, Brunner M, Canonizado EM, Charles D, Chiles TC, Donohue MJ, Enck J, Fenichel P, Fleming LE, Ferrier-Pages C, Fordham R, Gozt A, Griffin C, Hahn ME, Haryanto B, Hixson R, Ianelli H, James BD, Kumar P, Laborde A, Law KL, Martin K, Mu J, Mulders Y, Mustapha A, Niu J, Pahl S, Park Y, Pedrotti ML, Pitt JA, Ruchirawat M, Seewoo BJ, Spring M, Stegeman JJ, Suk W, Symeonides C, Takada H, Thompson RC, Vicini A, Wang Z, Whitman E, Wirth D, Wolff M, Yousuf AK, Dunlop S (2023) The Minderoo-Monaco Commission on plastics and human health. Annals of Global Health 89, 23.
| Crossref | Google Scholar | PubMed |
Lein MR (1972) A trophic comparison of avifaunas. Systematic Biology 21, 135-150.
| Crossref | Google Scholar |
Lenka SP, Kah M, Padhye LP (2021) A review of the occurrence, transformation, and removal of poly- and perfluoroalkyl substances (PFAS) in wastewater treatment plants. Water Research 199, 117187.
| Crossref | Google Scholar | PubMed |
Letcher RJ, Bustnes JO, Dietz R, Jenssen BM, Jorgensen EH, Sonne C, Verreault J, Vijayan MM, Gabrielsen GW (2010) Exposure and effects assessment of persistent organohalogen contaminants in arctic wildlife and fish. Science of The Total Environment 408, 2995-3043.
| Crossref | Google Scholar | PubMed |
Li Y, Oliver DP, Kookana RS (2018) A critical analysis of published data to discern the role of soil and sediment properties in determining sorption of per and polyfluoroalkyl substances (PFASs). Science of The Total Environment 628–629, 110-120.
| Crossref | Google Scholar | PubMed |
Li P, Oyang X, Zhao Y, Tu T, Tian X, Li L, Zhao Y, Li J, Xiao Z (2019) Occurrence of perfluorinated compounds in agricultural environment, vegetables, and fruits in regions influenced by a fluorine-chemical industrial park in China. Chemosphere 225, 659-667.
| Crossref | Google Scholar | PubMed |
Lindstrom AB, Strynar MJ, Libelo EL (2011) Polyfluorinated compounds: past, present, and future. Environmental Science & Technology 45, 7954-7961.
| Crossref | Google Scholar | PubMed |
Lopez-Antia A, Groffen T, Lasters R, AbdElgawad H, Sun J, Asard H, Bervoets L, Eens M (2019) Perfluoroalkyl Acids (PFAAs) concentrations and oxidative status in two generations of great tits inhabiting a contamination hotspot. Environmental Science & Technology 53, 1617-1626.
| Crossref | Google Scholar | PubMed |
Louis GMB, Chen Z, Schisterman EF, Kim S, Sweeney AM, Sundaram R, Lynch CD, Gore-Langton RE, Barr DB (2015) Perfluorochemicals and human semen quality: the LIFE study. Environmental Health Perspectives 123, 57-63.
| Crossref | Google Scholar | PubMed |
Ma D, Lu Y, Liang Y, Ruan T, Li J, Zhao C, Wang Y, Jiang G (2022) A critical review on transplacental transfer of per- and polyfluoroalkyl substances: prenatal exposure levels, characteristics, and mechanisms. Environmental Science & Technology 56, 6014-6026.
| Crossref | Google Scholar | PubMed |
Martin JW, Smithwick MM, Braune BM, Hoekstra PF, Muir DCG, Mabury SA (2004) Identification of long-chain perfluorinated acids in biota from the Canadian Arctic. Environmental Science & Technology 38, 373-380.
| Crossref | Google Scholar | PubMed |
McIver SC, Roman SD, Nixon B, Loveland KL, McLaughlin EA (2013) The rise of testicular germ cell tumours: the search for causes, risk factors and novel therapeutic targets. F1000Research 2, 55.
| Crossref | Google Scholar | PubMed |
Mikkonen AT, Martin J, Upton RN, Barker AO, Brumley CM, Taylor MP, Mackenzie L, Roberts MS (2023) Spatio-temporal trends in livestock exposure to per- and polyfluoroalkyl substances (PFAS) inform risk assessment and management measures. Environmental Research 225, 115518.
| Crossref | Google Scholar | PubMed |
Moody CA, Field JA (1999) Determination of perfluorocarboxylates in groundwater impacted by fire-fighting activity. Environmental Science & Technology 33, 2800-2806.
| Crossref | Google Scholar |
Muir D, Bossi R, Carlsson P, Evans M, De Silva A, Halsall C, Rauert C, Herzke D, Hung H, Letcher R, Rigét F, Roos A (2019) Levels and trends of poly- and perfluoroalkyl substances in the Arctic environment – an update. Emerging Contaminants 5, 240-271.
| Crossref | Google Scholar |
Newsted JL, Coady KK, Beach SA, Butenhoff JL, Gallagher S, Giesy JP (2007) Effects of perfluorooctane sulfonate on mallard and northern bobwhite quail exposed chronically via the diet. Environmental Toxicology and Pharmacology 23, 1-9.
| Crossref | Google Scholar | PubMed |
Nickerson A, Maizel AC, Kulkarni PR, Adamson DT, Kornuc JJ, Higgins CP (2020) Enhanced extraction of AFFF-associated PFASs from source zone soils. Environmental Science & Technology 54, 4952-4962.
| Crossref | Google Scholar | PubMed |
Noorlander CW, van Leeuwen SPJ, Te Biesebeek JD, Mengelers MJB, Zeilmaker MJ (2011) Levels of perfluorinated compounds in food and dietary intake of PFOS and PFOA in the Netherlands. Journal of Agricultural and Food Chemistry 59, 7496-7505.
| Crossref | Google Scholar | PubMed |
Obsekov V, Kahn LG, Trasande L (2023) Leveraging systematic reviews to explore disease burden and costs of per- and polyfluoroalkyl substance exposures in the United States. Exposure and Health 15, 373-394.
| Crossref | Google Scholar | PubMed |
Olsen GW, Zobel LR (2007) Assessment of lipid, hepatic, and thyroid parameters with serum perfluorooctanoate (PFOA) concentrations in fluorochemical production workers. International Archives of Occupational and Environmental Health 81, 231-246.
| Crossref | Google Scholar | PubMed |
Olsen GW, Gilliland FD, Burlew MM, Burris JM, Mandel JS, Mandel JH (1998) An epidemiologic investigation of reproductive hormones in men with occupational exposure to perfluorooctanoic acid. Journal of Occupational & Environmental Medicine 40, 614-622.
| Crossref | Google Scholar | PubMed |
Patrick R, Noy S, Henderson-Wilson C, Hayward J, Allender S, Gunasiri H, Bruges N, Snell T, Capetola T (2023a) Minding our futures: understanding climate-related mental wellbeing using systems science. Health & Place 84, 103134.
| Crossref | Google Scholar | PubMed |
Patrick R, Snell T, Gunasiri H, Garad R, Meadows G, Enticott J (2023b) Prevalence and determinants of mental health related to climate change in Australia. Australian & New Zealand Journal of Psychiatry 57, 710-724.
| Crossref | Google Scholar | PubMed |
Perez F, Nadal M, Navarro-Ortega A, Fabrega F, Domingo JL, Barcelo D, Farre M (2013) Accumulation of perfluoroalkyl substances in human tissues. Environment International 59, 354-362.
| Crossref | Google Scholar | PubMed |
Persson L, Carney Almroth BM, Collins CD, Cornell S, de Wit CA, Diamond ML, Fantke P, Hassellov M, MacLeod M, Ryberg MW, Sogaard Jorgensen P, Villarrubia-Gomez P, Wang Z, Hauschild MZ (2022) Outside the safe operating space of the planetary boundary for novel entities. Environmental Science & Technology 56, 1510-1521.
| Crossref | Google Scholar | PubMed |
Rand AA, Mabury SA (2017) Is there a human health risk associated with indirect exposure to perfluoroalkyl carboxylates (PFCAs)? Toxicology 375, 28-36.
| Crossref | Google Scholar | PubMed |
Robuck AR, Cantwell MG, McCord JP, Addison LM, Pfohl M, Strynar MJ, McKinney R, Katz DR, Wiley DN, Lohmann R (2020) Legacy and novel per- and polyfluoroalkyl substances in juvenile seabirds from the U.S. atlantic coast. Environmental Science & Technology 54, 12938-12948.
| Crossref | Google Scholar | PubMed |
Rockstrom J, Steffen W, Noone K, Persson A, Chapin FS, III, Lambin EF, Lenton TM, Scheffer M, Folke C, Schellnhuber HJ, Nykvist B, de Wit CA, Hughes T, van der Leeuw S, Rodhe H, Sorlin S, Snyder PK, Costanza R, Svedin U, Falkenmark M, Karlberg L, Corell RW, Fabry VJ, Hansen J, Walker B, Liverman D, Richardson K, Crutzen P, Foley JA (2009) A safe operating space for humanity. Nature 461, 472-475.
| Crossref | Google Scholar | PubMed |
Ross I, McDonough J, Miles J, Storch P, Thelakkat Kochunarayanan P, Kalve E, Hurst J, Dasgupta SS, Burdick J (2018) A review of emerging technologies for remediation of PFASs. Remediation Journal 28, 101-126.
| Crossref | Google Scholar |
Sakr CJ, Kreckmann KH, Green JW, Gillies PJ, Reynolds JL, Leonard RC (2007a) Cross-sectional study of lipids and liver enzymes related to a serum biomarker of exposure (ammonium perfluorooctanoate or APFO) as part of a general health survey in a cohort of occupationally exposed workers. Journal of Occupational & Environmental Medicine 49, 1086-1096.
| Crossref | Google Scholar | PubMed |
Sakr CJ, Leonard RC, Kreckmann KH, Slade MD, Cullen MR (2007b) Longitudinal study of serum lipids and liver enzymes in workers with occupational exposure to ammonium perfluorooctanoate. Journal of Occupational & Environmental Medicine 49, 872-879.
| Crossref | Google Scholar | PubMed |
Schneider-Mayerson M, Leong KL (2020) Eco-reproductive concerns in the age of climate change. Climatic Change 163, 1007-1023.
| Crossref | Google Scholar |
Scientific Committee on Health, Environmental and Emerging Risks (SCHEER) (2018) Statement on emerging health and environmental issues (2018). DG Health and Food Safety, European Commission, Luxembourg. Available at https://health.ec.europa.eu/system/files/2019-02/scheer_s_002_0.pdf [Accessed February 2024]
Skakkebaek NE, Rajpert-De Meyts E, Jorgensen N, Main KM, Leffers H, Andersson A-M, Juul A, Jensen TK, Toppari J (2007) Testicular cancer trends as ‘whistle blowers’ of testicular developmental problems in populations. International Journal of Andrology 30, 198-205.
| Crossref | Google Scholar | PubMed |
Smithwick M, Norstrom RJ, Mabury SA, Solomon K, Evans TJ, Stirling I, Taylor MK, Muir DCG (2006) Temporal trends of perfluoroalkyl contaminants in polar bears (Ursus maritimus) from two locations in the North American Arctic, 1972-2002. Environmental Science & Technology 40, 1139-1143.
| Crossref | Google Scholar | PubMed |
Song X, Tang S, Zhu H, Chen Z, Zang Z, Zhang Y, Niu X, Wang X, Yin H, Zeng F, He C (2018) Biomonitoring PFAAs in blood and semen samples: investigation of a potential link between PFAAs exposure and semen mobility in China. Environment International 113, 50-54.
| Crossref | Google Scholar | PubMed |
Steenland K, Fletcher T, Stein CR, Bartell SM, Darrow L, Lopez-Espinosa M-J, Barry Ryan P, Savitz DA (2020) Review: evolution of evidence on PFOA and health following the assessments of the C8 Science Panel. Environment International 145, 106125.
| Crossref | Google Scholar | PubMed |
Steffen W, Richardson K, Rockstrom J, Cornell SE, Fetzer I, Bennett EM, Biggs R, Carpenter SR, de Vries W, de Wit CA, Folke C, Gerten D, Heinke J, Mace GM, Persson LM, Ramanathan V, Reyers B, Sorlin S (2015) Planetary boundaries: guiding human development on a changing planet. Science 347, 1259855.
| Crossref | Google Scholar | PubMed |
Stemmler I, Lammel G (2010) Pathways of PFOA to the Arctic: variabilities and contributions of oceanic currents and atmospheric transport and chemistry sources. Atmospheric Chemistry and Physics 10, 9965-9980.
| Crossref | Google Scholar |
Stockholm Convention (2023) Protecting human health and the environment from persistent organic pollutants. United Nations Environmental Programme, Geneva, Switzerland. Available at https://chm.pops.int/Implementation/IndustrialPOPs/PFAS/Overview/tabid/5221/Default.aspx [Accessed February 2024]
Strynar MJ, Lindstrom AB, Nakayama SF, Egeghy PP, Helfant LJ (2012) Pilot scale application of a method for the analysis of perfluorinated compounds in surface soils. Chemosphere 86, 252-257.
| Crossref | Google Scholar | PubMed |
Sunderland EM, Hu XC, Dassuncao C, Tokranov AK, Wagner CC, Allen JG (2019) A review of the pathways of human exposure to poly- and perfluoroalkyl substances (PFASs) and present understanding of health effects. Journal of Exposure Science & Environmental Epidemiology 29, 131-147.
| Crossref | Google Scholar | PubMed |
Szabo D, Coggan TL, Robson TC, Currell M, Clarke BO (2018) Investigating recycled water use as a diffuse source of per- and polyfluoroalkyl substances (PFASs) to groundwater in Melbourne, Australia. Science of The Total Environment 644, 1409-1417.
| Crossref | Google Scholar | PubMed |
Szabo D, Marchiandi J, Samandra S, Johnston JM, Mulder RA, Green MP, Clarke BO (2023) High-resolution temporal wastewater treatment plant investigation to understand influent mass flux of per- and polyfluoroalkyl substances (PFAS). Journal of Hazardous Materials 447, 130854.
| Crossref | Google Scholar | PubMed |
Tartu S, Gabrielsen GW, Blevin P, Ellis H, Bustnes JO, Herzke D, Chastel O (2014) Endocrine and fitness correlates of long-chain perfluorinated carboxylates exposure in Arctic breeding black-legged kittiwakes. Environmental Science & Technology 48, 13504-13510.
| Crossref | Google Scholar | PubMed |
Turner BD, Sloan SW, Currell GR (2019) Novel remediation of per- and polyfluoroalkyl substances (PFASs) from contaminated groundwater using Cannabis Sativa L. (hemp) protein powder. Chemosphere 229, 22-31.
| Crossref | Google Scholar | PubMed |
United States Environmental Protection Agency (US EPA) (2021) EPA advances science to protect the public from PFOA and PFOS in drinking water. EPA Press Office, Washington DC, USA. Available at https://www.epa.gov/newsreleases/epa-advances-science-protect-public-pfoa-and-pfos-drinking-water [Accessed February 2024]
United States Environmental Protection Agency (US EPA) (2022) Technical fact sheet: drinking water health advisories for four PFAS (PFOA, PFOS, GenX chemicals, and PFBS). EPA Press Office, Washington, DC, USA. Available at https://www.epa.gov/system/files/documents/2022-06/technical-factsheet-four-PFAS.pdf [Accessed February 2024]
Vendl C, Taylor MD, Braunig J, Gibson MJ, Hesselson D, Neely GG, Lagisz M, Nakagawa S (2021) Profiling research on PFAS in wildlife: protocol of a systematic evidence map and bibliometric analysis. Ecological Solutions and Evidence 2, e12106.
| Crossref | Google Scholar |
Vested A, Ramlau-Hansen CH, Olsen SF, Bonde JP, Kristensen SL, Halldorsson TI, Becher G, Haug LS, Ernst EH, Toft G (2013) Associations of in utero exposure to perfluorinated alkyl acids with human semen quality and reproductive hormones in adult men. Environmental Health Perspectives 121, 453-458.
| Crossref | Google Scholar | PubMed |
Wang Z, DeWitt J, Higgins CP, Cousins IT (2018) Correction to “a never-ending story of per- and polyfluoroalkyl substances (PFASs)?”. Environmental Science & Technology 52, 3325.
| Crossref | Google Scholar | PubMed |
Weiß O, Wiesmuller GA, Bunte A, Goen T, Schmidt CK, Wilhelm M, Holzer J (2012) Perfluorinated compounds in the vicinity of a fire training area – human biomonitoring among 10 persons drinking water from contaminated private wells in Cologne, Germany. International Journal of Hygiene and Environmental Health 215, 212-215.
| Crossref | Google Scholar | PubMed |
WHO (2018) Circular economy and health: opportunities and risks. World Health Organization. Geneva, Switzerland. Available at https://www.medbox.org/document/circular-economy-and-health-opportunities-and-risks#GO [Accessed February 2024]
Xing Y, Zhou Y, Zhang X, Lin X, Li J, Liu P, Lee HK, Huang Z (2023) The sources and bioaccumulation of per- and polyfluoroalkyl substances in animal-derived foods and the potential risk of dietary intake. Science of The Total Environment 905, 167313.
| Crossref | Google Scholar | PubMed |
Yamashita N, Kannan K, Taniyasu S, Horii Y, Petrick G, Gamo T (2005) A global survey of perfluorinated acids in oceans. Marine Pollution Bulletin 51, 658-668.
| Crossref | Google Scholar | PubMed |
Yamashita N, Taniyasu S, Petrick G, Wei S, Gamo T, Lam PKS, Kannan K (2008) Perfluorinated acids as novel chemical tracers of global circulation of ocean waters. Chemosphere 70, 1247-1255.
| Crossref | Google Scholar | PubMed |
Ye X, Kato K, Wong L-Y, Jia T, Kalathil A, Latremouille J, Calafat AM (2018) Per- and polyfluoroalkyl substances in sera from children 3 to 11 years of age participating in the National Health and Nutrition Examination Survey 2013-2014. International Journal of Hygiene and Environmental Health 221, 9-16.
| Crossref | Google Scholar | PubMed |
Yeung LWY, So MK, Jiang G, Taniyasu S, Yamashita N, Song M, Wu Y, Li J, Giesy JP, Guruge KS, Lam PKS (2006) Perfluorooctanesulfonate and related fluorochemicals in human blood samples from China. Environmental Science & Technology 40, 715-720.
| Crossref | Google Scholar | PubMed |
Zalasiewicz J, Williams M, Haywood A, Ellis M (2011) The Anthropocene: a new epoch of geological time? Philosophical Transactions of the Royal Society A: Mathematical, Physical and Engineering Sciences 369, 835-841.
| Crossref | Google Scholar | PubMed |
Zhang H, Lu Y, Luo B, Yan S, Guo X, Dai J (2014) Proteomic analysis of mouse testis reveals perfluorooctanoic acid-induced reproductive dysfunction via direct disturbance of testicular steroidogenic machinery. Journal of Proteome Research 13, 3370-3385.
| Crossref | Google Scholar | PubMed |
Zhao Z, Cheng X, Hua X, Jiang B, Tian C, Tang J, Li Q, Sun H, Lin T, Liao Y, Zhang G (2020) Emerging and legacy per- and polyfluoroalkyl substances in water, sediment, and air of the Bohai Sea and its surrounding rivers. Environmental Pollution 263, 114391.
| Crossref | Google Scholar | PubMed |
Zhou J, Baumann K, Surratt JD, Turpin BJ (2022) Legacy and emerging airborne per- and polyfluoroalkyl substances (PFAS) collected on PM2.5 filters in close proximity to a fluoropolymer manufacturing facility. Environmental Science: Processes & Impacts 24, 2272-2283.
| Crossref | Google Scholar | PubMed |
Zhu Y, Pan X, Jia Y, Yang X, Song X, Ding J, Zhong W, Feng J, Zhu L (2023) Exploring route-specific pharmacokinetics of PFAS in mice by coupling in vivo tests and physiologically based toxicokinetic models. Environmental Health Perspectives 131, 127012.
| Crossref | Google Scholar | PubMed |