Floral biology, pollination vectors and breeding system of Zieria granulata (Rutaceae), an endangered shrub endemic to eastern Australia
Laura C. Lopresti


A Centre for Sustainable Ecosystem Solutions and School of Earth, Atmospheric and Life Sciences, University of Wollongong, Wollongong, NSW 2522, Australia.
B The Australian PlantBank, Australian Institute of Botanical Science, The Royal Botanic Gardens and Domain Trust, Mount Annan, NSW 2567, Australia.
C Hawkesbury Institute for the Environment, Western Sydney University, Locked Bag 1797, Penrith, NSW 2751, Australia.
Australian Journal of Botany 71(5) 252-268 https://doi.org/10.1071/BT22055
Submitted: 25 May 2022 Accepted: 2 June 2023 Published: 23 June 2023
© 2023 The Author(s) (or their employer(s)). Published by CSIRO Publishing. This is an open access article distributed under the Creative Commons Attribution-NonCommercial-NoDerivatives 4.0 International License (CC BY-NC-ND)
Abstract
Context: Understanding the factors that influence viable-seed production is crucial in the conservation of threatened plant species, yet these factors are often poorly understood.
Aims: We investigated the reproductive biology of Zieria granulata C.Moore ex Benth., an endangered Australian endemic with a limited distribution, with the intent of improving conservation and restoration outcomes.
Methods: Components of floral biology, including floral ontogeny and nectar production, were quantified to determine the pollination syndrome and the likely breeding system. Flower-visitor surveys (using both digital video recordings and human observations), a manipulative wind-pollination experiment and hand-pollination experiments were conducted to investigate pollination vectors and confirm the breeding system.
Key results: Z. granulata flowers were small, white, protandrous and produced highly ornamented pollen grains and small quantities of nectar; these characteristics suggest that the species fits the general entomophily syndrome. All floral visitors were arthropod species and of the 72 visitors observed, predominantly from the Dipteran and Hymenopteran families, 18 could be regarded as potential pollinators. Failure of simulated wind gusts (40 km h−1) to transport pollen ≥5 cm indicated that anemophily is unlikely for this species. Autonomous and manipulative selfing did not result in viable seed set, indicating that this is an obligate outcrossing species. However, fruit and viable-seed production was highly variable within and among some other treatments. Pre-dispersal seed predation was recorded at all study sites.
Conclusions: Pre-dispersal seed predation was recorded at all study sites and is a likely factor inhibiting viable-seed production.
Implications: This knowledge will be used to improve seed yield for collections used for ex situ conservation and restoration programs for the endangered Z. granulata.
Keywords: entomophilous, entomophily, Illawarra Zieria, insect–plant mutualism, mating systems, parasitoid, plant–animal interaction, pollination syndrome, seed viability.
Introduction
The reproductive success of angiosperms hinges on the production of viable seed. That is, seeds housing an embryo that is live and capable of germinating under suitable environmental conditions (Bradbeer 1988). The production of viable seed in angiosperms is known to be highly variable, and a wide range of factors has been suggested to contribute to this variation (Burd 1994; Fenner and Thompson 2005; Chen and Zuo 2019). The most common factors limiting viable-seed production include inbreeding (Baskin and Baskin 2015), whereby inbreeding depression lowers seed viability, pollen limitation (Stephenson 1981), resource limitation (Lee 1988), and seed predation (Auld 2001; Armstrong 2002).
Non-clonal angiosperms are typically dependent on a pollination vector for reproduction, and pollen limitation can influence fruit set and thus seed production (Stephenson 1981; Knight et al. 2005; Chen and Zuo 2019). Pollen limitation may be the result of an inadequate supply of quality pollen or a lack of suitable biotic pollinators (Chen and Zuo 2019). Despite their importance in the plant lifecycle, such basic biological and ecological interactions remain unstudied for many species, including whether a pollination vector is needed and, if so, what type of vector is utilised (Williams and Adam 2001; Bennett et al. 2018). Lack of knowledge in these areas prevents detailed understanding of the factors influencing viable-seed production and, in turn, can hinder successful conservation and restoration efforts (Martyn et al. 2009; Chen and Zuo 2019).
Viable-seed production is known to be variable in some plant taxa, particularly the Rutaceae (Auld 2001; Martyn et al. 2009). Within that family, seed collections for the genus Zieria have been observed to have seed fill (i.e. the proportion of seeds containing an embryo) ranging from 0 to 100% (Martyn et al. 2009). In total, 18 of the 39 taxa in this genus occurring in New South Wales are currently listed as endangered (Biodiversity Conservation Act 2016) (DPIE 2021), and habitat for Zieria species is generally poorly conserved (Armstrong 2002). Ex situ conservation is vital to support the long-term conservation of these species, while simultaneously supporting in situ restoration (Sommerville et al. 2017). Seed banking is considered the most effective and cost-efficient ex situ conservation technique (Martyn Yenson et al. 2021). However, the value of the seed store is dependent on the availability of viable seed and the capacity to successfully preserve that seed for future germination (Martyn et al. 2009). Nevertheless, the factors influencing viable-seed production in endangered Zieria species have not been examined (Auld 2001; Armstrong 2002; Martyn et al. 2009).
Zieria granulata (Rutaceae) is a tall, bushy shrub listed as endangered under both the Commonwealth Environment Protection and Biodiversity Conservation Act 1999 and the New South Wales (NSW) Biodiversity Conservation Act 2016. It is endemic to the Illawarra region in NSW, Australia, where it is restricted to a few isolated populations in the Shellharbour and Kiama local government areas (DPIE 2018). Much of the natural habitat of the species has been destroyed by land-clearing and the remaining habitat is highly fragmented (Mills and Jackeman 1995). A recovery plan developed for the species in 2005 highlighted the need for an ex situ seed conservation program to safeguard genetic material from extinction (DEC 2005). A single study on Z. granulata seeds held at The Australian PlantBank found that up to 58% of filled seeds stored at 15°C for 2.3 years were not viable (Martyn et al. 2009). The factors contributing to this remain unknown. A study examining the floral and pollen morphology of the species, coupled with opportunistic observations of nectar-seeking flies foraging on its flowers, suggested that it is likely that Z. granulata is pollinated by insects (Armstrong 2002). There have been no studies on the breeding system, floral phenology, or pollination vectors of this species (but see Armstrong 2002).
We combined an investigation on the floral biology, pollination vectors and breeding system to determine the factors contributing to viable-seed production in the endangered species Z. granulata. This study aimed to (1) examine the floral biology of Z. granulata to provide insights into the likely pollination syndrome and breeding system, (2) identify the full suite of floral visitors (diurnal and nocturnal) throughout the entire natural distribution of Z. granulata and, by observing the foraging behaviour and pollen load of those visitors, to identify potential pollinators, (3) determine whether anemophily plays a role in pollination of this species and (4) confirm the breeding system by quantifying fruit and seed set in response to hand-pollination experiments.
Materials and methods
Study sites
This study was conducted between August and December 2020, at six locations: Jerrara Dam Reserve (34°40′19″S, 150°48′22″E) (hereafter Jerrara), Spring Creek Wetland Kiama (34°39′41″S, 150°50′48″E) (hereafter Kiama), Spring Creek Wetland South Kiama (34°39′48″S, 150°50′40″E) (hereafter South Kiama), Killalea Reserve Shellharbour (34°35′28″S, 150°51′40″E) (hereafter Shellharbour), Austinmer (34°18′20″S, 150°55′34″E), and Wollongong Botanic Gardens (34°24′34″S, 150°52′30″E) (hereafter Wollongong) (Fig. 1). Study sites located at Jerrara, Kiama, South Kiama and Shellharbour contain natural populations located in remnant and regrowth rainforest, study sites at Austinmer and Wollongong contain two and five individuals respectively, planted in a garden bed.
Locations of populations used to study the reproductive biology of Zieria granulata in New South Wales, Australia. Occurrence records of Zieria granulata depict the species’ entire natural distribution (data sourced from DPIE 2020).
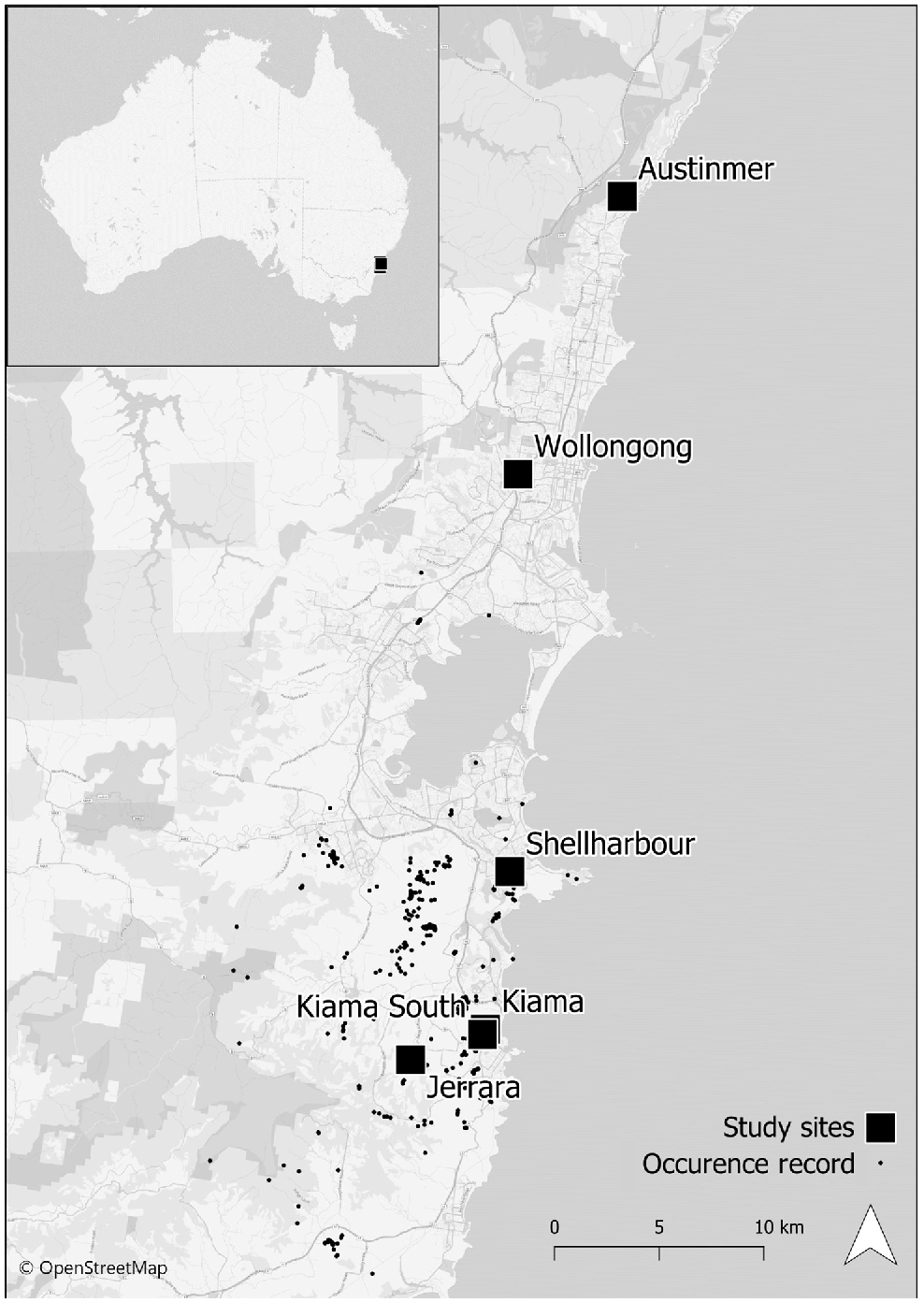
The methods for investigating the floral biology, floral visitors and pollinators, and breeding system followed those described in Lopresti et al. (2023).
Floral biology
To examine the floral phenology of Z. granulata, 10 flowers from two shrubs at both Wollongong and Austinmer were observed daily from floral opening to abscission or fertilisation (following Kearns and Inouye 1993). For both androecium and gynoecium development, reproductive structures were defined as immature, mature or senesced for each flower. The androecium was considered mature when anther heads began dehiscing and pollen grains were visible (when viewed through a hand-lens). Gynoecium maturity was based on the size and colour of the stigma. The first day that the androecium and gynoecium matured, duration of maturity, and the total anthesis period were recorded.
To gain insight into potential vectors of pollination and the breeding system, pollen morphology and exine characteristics were observed and described as outlined by Halbritter et al. (2018). Key morphological characteristics described included pollen shape, from both equatorial (E) and polar (P) views, pollen shape in relation to the P:E ratio (length of the polar axis between the two poles compared with the equatorial diameter), pollen unit (the dispersal unit of mature pollen grains), and ornamentation of the aperture and exine regions. Stamens from three flowers located at Wollongong were examined under a scanning electron microscope (JEOL JSM-6490LA, Japan) on the day of collection. Each stamen was mounted on a metal stub, using conductive double-sided carbon tape, and sputter-coated with 20 nm gold using an Edwards AUTO 306 Sputter Coater (Edwards Australia, Yatala, Queensland). Imaging was completed in high-vacuum mode at 10 mm working distance using secondary electrons and backscattered electrons imaging (SEI/BSE) at 15 kV operating voltage and with a spot-size setting of 45.
Nectar abundance was measured to determine whether flowers provided a reward to potential pollinators. Inflorescences that housed at least five buds near to opening were selected haphazardly and enclosed within bags made of polyorganza fabric (10 cm wide × 5 cm long) to prevent biotic visitors extracting nectar prior to the flowers opening. Nectar was then extracted from five flowers with a mature androecium and five flowers with a mature gynoecium, at Jerrara, Kiama, South Kiama and Shellharbour. Nectar was extracted into a 1 μL microcapillary tube (following Morrant et al. 2009), and the volume withdrawn was determined by calculating the proportion of the column that was filled. Differences in nectar production between flowers with a mature androecium and those with a mature gynoecium were analysed using a Student’s t-test on untransformed data after ensuring that the data met assumptions of normality. Because of the small sample sizes, data were pooled across sites.
Floral visitors and biotic pollination vectors
Floral visitors were defined as species contacting any part of the flower and visitation was assessed through a combination of human observations (diurnal and nocturnal), digital video recordings, time-lapse photography, and nocturnal infrared motion-sensor imaging. Sampling was conducted on 3–10 shrubs at three to four sites per method (see Supplementary Table S1 for sampling details). For all sampling methods, observations of floral visitors were made on days with minimal cloud cover, wind speeds not exceeding 20 km h−1 and temperatures ranging from 17°C to 32°C.
Preliminary assessments to guide the survey effort were undertaken on floral visitors to Sarcomelicope simplicifolia (Endl.) T.G.Hartley subsp. simplicifolia (Rutaceae) flowers at Jerrara, Kiama and South Kiama. Brinno TLC200 Pro HDR (Brinno Incorporated, Taiwan) cameras were set to record floral visitors between sunrise and sunset over three different days at each site prior to Z. granulata flowers opening (August 2020).
Diurnal human observations were conducted from 09:00 hours to 15:00 hours as preliminary assessment indicated that visitation was reduced outside this time. Digital video recordings (DVRs) were also employed during the same period (09:00 hours to 15:00 hours) to increase total observation time and capture cryptic species that may be deterred by human presence (as described by Dafni (1992)). TLP was conducted between first light and last light, to observe rare faunal species that may be active outside the period from 09:00 hours to 15:00 hours and guide the survey effort for human observations (diurnal and nocturnal) and DVR. Infrared cameras were employed between last light (approximately 18:15 hours) and first light (approximately 05:20 hours) to guide the survey effort for nocturnal human observations, and nocturnal human observations were conducted over a 3-h period after last light. For all methods, sampling was conducted on accessible canopy with high floral density and with the flowers housing mature androecia or gynoecia. All sampling methods were conducted within 2 m of the ground on the outermost layer of the canopy.
Diurnal human observations were conducted by observing six fixed sections (25 cm × 25 cm) of canopy per shrub. Section area was determined on the basis of the ability of an observer to confidently track the movements of floral visitors. Floral visitors were opportunistically caught in specimen jars, then euthanised on ice for subsequent identification and pollen-presence identification. Euthanised insect specimens were identified under a dissecting microscope at ×40 magnification.
Digital video recordings (DVR, 1080 p resolution) were obtained using GoPro Hero4 Session CHDHS-101 digital cameras (GoPro Australia) attached to a tripod. This method was previously identified by Gilpin et al. (2017) as an effective method for conducting simultaneous observations of flower-visiting insects. Observations were completed in 2-h blocks, with up to six DVRs in operation at one time. The field of view of each camera captured a 15 cm × 30 cm area of canopy.
Time-lapse photographs (TLP) were captured using Brinno TLC200 Pro HDR (Brinno Incorporated, Taiwan) cameras. Three cameras were set to capture an image every 2 s for the full period of daylight on three non-consecutive days at four study sites (Table S1). To determine whether floral visitors foraged outside the primary survey period from 09:00 hours to 15:00 hours, floral visitor presence and the time of day were recorded for all species detected on TLP. Floral visitors detected on TLP were not identified to a high taxonomic level because of low image quality.
Nocturnal human observations were made over three non-consecutive nights at three sites. Sampling was conducted in one 3 h block each night, starting at 20:30 hours. An initial 10-min waiting period was adopted, prior to using a battery-operated torch to scan randomly selected floral patches for 5 s (following Hermansen et al. 2014). Scans were completed by two observers every 55 s and a new shrub was surveyed each hour. Nocturnal human observations were not made at Wollongong owing to evening access restrictions.
Nocturnal infrared-camera recordings (ICR) were made using Bushnell Trophy Cam HD Essential E3 Trail motion sensor cameras. Two cameras were set to record a single inflorescence (camera field of view 15 cm × 30 cm) from last light to first light over two nights at each of the four study sites (Table S1). To determine whether floral visitors foraged throughout the night, floral visitor presence and time of night were recorded for each species detected on ICR.
A floral visitor was defined as a potential pollinator if it carried the pollen of the target species anywhere on its body, determined through inspection under a dissecting microscope (Kearns and Inouye 1993), or made contact with the stigmatic surface of at least one flower of the target species (Dafni 1992). To examine whether a visitor detected by DVR acted as a potential pollinator, any contact with the stigmatic surface was recorded for all visiting species. During in-person observations, floral visitors were captured using a jar and then placed in a cool box filled with ice and transferred to the freezer. The presence of pollen on captured individuals was detected using a dissecting microscope at ×40 magnification. Individuals carrying pollen were then examined under a scanning electron microscope (SEM) (JEOL JSM-6490LA, Japan) to determine whether the pollen carried matched specimens previously collected from Z. granulata. For scanning electron microscopy, specimens were prepared and imaged as previously described.
To determine whether any potential pollinator was dominant compared with others, the percentage of sampling intervals in which a pollinator of a given species was present (hereafter referred to as ‘presence’) was compared across taxonomic groups. Presence was calculated for each of the observational methods in which potential pollinators were detected, namely, human observations and DVR. The duration of each sampling interval on which the presence calculation was based was dependent on the method, being 5 min for human observations and 2 h for DVR. This variable provided an estimation of the frequency of visitation for each potential pollinator observed under each method.
To examine whether there was a significant difference in presence among pollinating species, a multi-factor generalised linear model (GLM) was used. A binomial error structure and logit-link function were used for the model, where presence or absence of each pollinating taxon was the response variable and pollinator species, sampling method (DVR or human observations) and site were the predictor variables. The reference level for species was Apis mellifera, DVR for sampling, and Jerrara for site. Many species exhibited complete separation (i.e. absence from some sites) when the interaction term (species × site) was included in this analysis. The interaction term was therefore excluded from the model when examining overall patterns of pollinator presence across all sites. Analyses were conducted using the statistical software R release 2022.07.1 (R Core Team 2022).
Wind pollination
To determine whether wind may act as a pollination vector, the pedicels of 20 open flowers were each taped separately to a rod so that the filaments were exposed. A 9 cm diameter Petri dish smeared with petrolium jelly (Vaseline) was placed 5 cm behind each flower and a fan was used to direct a 40 km h−1 burst of wind onto each flower and dish pair for 10 s. Wind speed was selected on the basis of average wind speed recorded at Sydney Airport from 2001 to 2020 (weather station ID 066037, Bureau of Meterology 2020a) and was measured using a digital anemometer (Kestrel 3500, accuracy ± 3%). Following exposure to wind, the Petri dishes were observed under a light microscope at ×100 magnification to determine whether pollen was present. Flowers were sourced from two shrubs at Kiama and two at Wollongong.
Breeding system
To determine the breeding system of Z. granulata, a series of pollination experiments was conducted on two to six shrubs at each site (depending on the population size). Inflorescences on each shrub housing a minimum of five flower buds near the opening were haphazardly selected and subjected to one of the following five randomly selected treatments: (1) manipulative outcross, (2) autonomous selfing, (3) manipulative selfing, (4) open control (no bag), or (5) procedural control (partial bag). Treatments were applied and replicated 10 times per shrub at each site (n = 210; Table S2 details the number of replicates retrieved per shrub per treatment at each site). The procedural control (Treatment 5) was applied only at one study site (Jerrara) because of time constraints. To ensure synchronicity of stigma receptivity within and among the treatments, inflorescences on a given shrub were selected on the same day for all five treatments, and any open flowers or very young buds were removed. White polyorganza bags (10 cm wide × 7.5 cm long) were used to exclude insects during the experiment.
Manipulative outcrossing involved bagging an inflorescence prior to flowers opening, and then hand-pollinating each flower with pollen sourced from a different shrub within 1 h of collecting the pollen. Eight filaments that housed visible pollen (viewed through a hand-lens) were removed with forceps from one or two flowers that were not assigned a pollination treatment. Anther heads were brushed onto the receptive stigma of each flower that was assigned a hand-pollination treatment. This was undertaken over three consecutive days, with pollen sourced from the same donor plant each day, to maximise the likelihood of viable pollen being deposited on a receptive stigma. The autonomous selfing treatment involved bagging an inflorescence without manipulating the pollen. Control inflorescences were not bagged and were thus open to pollinators. The procedural control had three 5 cm × 10 cm openings cut into each bag which allowed for pollinator visitation and tested whether the presence of a bag could have an effect on experimental outcomes. Bags that were compromised during the experimental period, such as those where the branch broke, the bag tore, or there were signs of insect activity (such as frass), were excluded from the final analysis.
Following the set-up of these experiments, insects (particularly A. melliferaLinnaeus 1758) were observed sequestering nectar through the polyorganza bags. Therefore, teabags (4 cm × 15 cm), which were lighter in weight and smaller in mesh size, were used in addition to polyorganza bags to better exclude biotic pollinators. Up to five buds were enclosed in each teabag and the bags were sealed with twist ties. Only the autonomous selfing treatment was completed at one study site (Kiama) by using this method because few flowers were still in the bud stage when teabags were implemented.
All inflorescences were monitored for reproductive success from the time of pollination to fruit maturation. Fruit development was defined as the swelling of the ovary after abscission of petals and stamens. Fruit was classed as mature when swelling had ceased and a colour change was observed in the exocarp. At this point, fruits were harvested and examined for viable seed. Reproductive success was defined as (1) the percentage of flowers that produced fruit, and (2) the percentage of flowers that produced viable seed. These measures were used to compare the effect of pollination treatments.
Z. granulata fruits each contained up to four cocci, i.e. individual segments of the fruit that each contain a single seed (sometimes called a mericarp; PlantNET 2022). Fruit matured within 3 months of pollination and was collected in December 2020. Mature fruits and seeds from each site were dissected and examined under a microscope (×40 magnification) to determine (a) the number of seeds per fruit, and (b) the proportion of filled seeds. A ‘filled’ seed was defined as one which contained an embryo and surrounding endosperm filling the entire space enclosed by the seed coat, whereas an ‘empty’ seed lacked both embryo and endosperm. The total number of seeds in each seed class was presented as a percentage of the seed count for each treatment. Additionally, because some seed dispersed prior to opening, individual cocci were classed as predated or not predated. A predated cocci had signs of insect predation, including an exit hole (in the seed coat or pericarp), frass (within the seed, where the seed was examined), or an insect present. The total number of predated seeds was presented as a percentage of the total number of cocci examined per treatment.
Many replicates were compromised during the pollination experiment by broken branches, loss or damage of pollination bags, death of the entire shrub, or signs of insect activity within the bags (e.g. frass). More so, vandals damaged all treatments at Shellharbour in the early stages of flowering. The experiment recommenced at Shellharbour, but no manipulative treatments were applied because few buds remained on the shrubs at this late stage of the flowering season. As not all treatments could be completed on all shrubs, multiple analyses were undertaken.
To determine reproductive success, differences in fruit production (calculated as a percentage of flowers) among treatments were analysed by ANOVA. For each model, fruit production and treatment were considered the response and predictor variables respectively. Untransformed data were analysed for all ANOVAs because assumptions of heterogeneity and normality were met. All data were analysed at α = 0.05 using the statistical software JMP Pro 15 (ver. 2.1, https://www.jmp.com/en_au/home.html). If a significant difference arose, a Tukey HSD analysis was undertaken to determine where the difference lay.
To test for bagging effects, a Student’s t-test was undertaken to compare fruit production between the procedural control (partial bag) and the open control (no bag) treatments.
To examine differences in fruit production among treatments (autonomous selfing, manipulative selfing, outcross and open control), a one-way ANOVA was applied. For this analysis, data were combined from all sites, shrubs and inflorescences, and the design was unbalanced. A randomly selected subset of the data was analysed to determine whether the same statistical outcomes were achieved with a balanced design (n = 24). Because the same trend in fruit production among treatments for each plant species was apparent in the second analysis, the complete data set was presented.
A separate three-factor nested ANOVA including site as a factor, and using a subset of the data (to ensure a balanced design), was performed to determine whether spatial factors influenced fruit production among treatments. This analysis was applied to two Z. granulata shrubs from both Austinmer (ID: 1, 2) and Jerrara (ID: 7, 8) that had three replicates of each of the four treatments (autonomous selfing, manipulative selfing, outcross and open control). ‘Plant’ and ‘site’ were considered random factors and ‘plant’ was nested within ‘site’. Interactions between treatments and ‘plant’ or ‘site’ were examined to determine whether fruit production was influenced by spatial factors.
Results
Floral biology
The sequence of floral development and maturation of reproductive structures indicated that this species is protandrous (Fig. 2). The androecium was mature and usually dehisced within 1 day of floral opening. Anthers dehisced and pollen was visible, on average, 3 (± 0.08 s.e.) days after floral opening (with pollen availability lasting between 1 and 7 days). The stigma became receptive between 4 and 17 days after floral opening (average 8 ± 0.16 days) and remained receptive for a maximum of 10 days (average 6 ± 0.13 days). There was no overlap between androecium maturity and gynoecium maturity; but temporal separation was variable, ranging from 1 to 8 days (average 3 ± 0.10). The anthesis period was, on average, 14 ± 0.25 days.
Zieria granulata flowers (a) with the mature androecium and immature gynoecium, and (b) housing an old androecium and mature gynoecium. The scale bar = 1 mm (in both images).
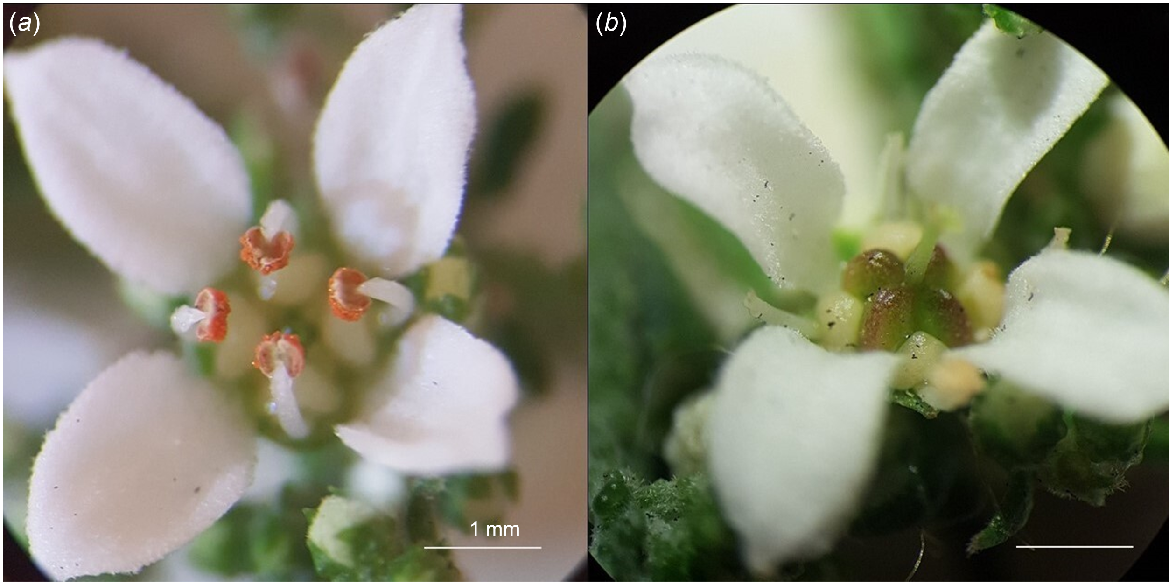
All pollen grains presented as discrete single units (monads) and were elliptical in shape. On the basis of the P:E ratio, the pollen shape was prolate. From polar view, the pollen grains were three-lobed circular and isopolar. Exine ornamentation was heterobrochate. Each grain contained three equidistant apertures, with two distinct aperture types, namely, endoaperture present (colporus aperture type) or endoaperture absent (colpus aperture type). Z. granulata pollen grains had a diameter of 25 μm and fit within the range considered small (PalDat 2020).
All flowers produced nectar, but nectar production did not exceed 0.1 μL (Fig. 3). Flowers with a mature gynoecium produced more than twice the volume of nectar produced by flowers with a mature androecium (t1,38 = 2.23, P = 0.032).
Floral visitors and biotic pollination vectors
Floral visitors of Z. granulata were diverse (72 species) and consisted predominantly of insects, with a few observations of other arthropods (spiders and millipedes; Table 1). Dipterans were the most species-rich taxon found visiting (30 species), followed by coleopterans (17 species), hymenopterans (12 species), lepidopterans (2 species), hemipterans (2 species), a neuropteran (1 species), a blattodean (1 species) and an orthopteran (1 species). One polydesmidan (millipede) species and one arachnid species were also recorded as visitors. In all, 12 of the 72 floral visitors were detected at more than one study site, with A. mellifera being present at all study sites (Table 1) and the ant (Dolichoderinae species) present at four study sites (Wollongong, Shellharbour, Jerrara and Kiama). Only two insects were detected visiting during human nocturnal surveys, namely, an ant (Myrmecia species) and an unidentified moth (Species 53; Table 1). Nocturnal visitors were not detected by ICR.
Class | Family | Taxon | Insect | Method detectedA | Number captured | Number detected by DVR | Pollen present | Stigma contact (%) (n) | Number of sites observed |
---|---|---|---|---|---|---|---|---|---|
Arachnid | Thomisidae | Species 1 | Spider | Direct | 4 | 0 | No | – | 1 |
Coleoptera | Buprestidae | Species 2 | Jewel beetle | Direct | 2 | 0 | No | – | 1 |
Coleoptera | Buprestidae | Species 3 | Jewel beetle | Direct | 1 | 0 | No | – | 1 |
Coleoptera | Cerambycidae | Species 4 | Bug | Direct | 1 | 0 | No | – | 1 |
Coleoptera | Chrysomelidae | Luperini species | Beetle | Direct | 2 | 0 | No | – | 1 |
Coleoptera | Chrysomelidae | Species 5 | Leaf beetle | Direct | 1 | 0 | No | – | 1 |
Coleoptera | Coccinellidae | Species 6 | Ladybug | Direct | 1 | 0 | No | – | 1 |
Coleoptera | Dermestidae | Species 7B | Skin beetle | Direct | 1 | 0 | Yes | – | 1 |
Coleoptera | Dermestidae | Species 8B | Skin beetle | Direct | 1 | 0 | Yes | – | 1 |
Coleoptera | Dermestidae | Species 9 | Skin beetle | Direct | 1 | 0 | No | – | 1 |
Coleoptera | Lycidae | Porrostoma species | Beetle | Direct | 1 | 0 | No | – | 1 |
Coleoptera | Unknown | Species 10–17 | Beetle | Both | 7 | 3 | No | – | – |
Diptera | Calliphoridae | Amenia chrysmae Walker, 1849B | Blow fly | Direct | 1 | 0 | Yes | – | 1 |
Diptera | Calliphoridae | Calliphora ochracea Schiner, 1868 | Blow fly | Direct | 1 | 0 | No | – | 1 |
Diptera | Calliphoridae | Species 19B | Blow fly | Direct | 10 | 0 | Yes | – | 3 |
Diptera | Calliphoridae | Species 20B | Blow fly | Direct | 1 | 0 | Yes | – | 1 |
Diptera | Muscidae | Dichaetomyia speciesB | Fly | Direct | 4 | 0 | No | – | 2 |
Diptera | Muscidae | Species 21B | Fly | DVR | 0 | 2 | – | 100 (1) | 1 |
Diptera | Muscidae | Species 22B | Fly | Direct | 4 | 0 | Yes | – | 3 |
Diptera | Muscidae | Species 23 | Fly | Direct | 2 | 0 | No | – | 1 |
Diptera | Muscidae | Species 24 | Fly | Direct | 5 | 0 | No | – | 3 |
Diptera | Sciaridae | Species 25 | Fungus gnat | Direct | 7 | 0 | No | – | 1 |
Diptera | Syrphidae | Eristaliinae species | Hover fly | Direct | 1 | 0 | – | – | 1 |
Diptera | Syrphidae | Melangyna speciesB | Hover fly | Both | 6 | 7 | Yes | 63 (7) | 3 |
Diptera | Tachinidae | Species 26B | Fly | Direct | 2 | 0 | Yes | – | 1 |
Diptera | Tipulidae | Species 27B | Fly | Direct | 1 | 0 | Yes | – | 1 |
Diptera | Unknown | Species 28B | Fly | DVR | 0 | 1 | – | 100 (1) | 1 |
Diptera | Unknown | Species 29B | Fly | DVR | 0 | 1 | – | 100 (1) | 1 |
Diptera | Unknown | Species 30–45 | Fly | Both | 14 | 1 | No | 0 (1) | – |
Hemiptera | Unknown | Species 46 | Beetle | Direct | 1 | 0 | No | – | 1 |
Hemiptera | Unknown | Species 47 | Beetle | Direct | 1 | 0 | No | – | 1 |
Hymenoptera | Apidae | Apis melliferaLinnaeus 1758B | Bee | Both | 83 | 13 | Yes | 85 (13) | 6 |
Hymenoptera | Apidae | Exoneura speciesB | Bee | Both | 17 | 9 | Yes | 100 (1) | 3 |
Hymenoptera | Colletidae | Hylaeus species | Bee | Direct | 2 | 0 | No | – | 2 |
Hymenoptera | Formicidae | Dolichoderinae species | Ant | Direct | 11 | 0 | No | – | 4 |
Hymenoptera | Formicidae | Myrmecia species | Bull Ant | DirectA | 1 | 0 | Yes | – | 1 |
Hymenoptera | Formicidae | Species 48 | Ant | DVR | 0 | 94 | – | 0.7 (94) | 1 |
Hymenoptera | Halictidae | Lasioglossum pecies 49B | Bee | Direct | 1 | 0 | Yes | – | 1 |
Hymenoptera | Halictidae | Lasioglossum species 50B | Bee | Direct | 15 | 0 | Yes | – | 3 |
Hymenoptera | Gasteruptiidae | Species 51 | Wasp | Direct | 4 | 0 | No | – | 2 |
Hymenoptera | Halictidae | Halictus speciesB | Bee | Direct | 9 | 0 | Yes | – | 3 |
Hymenoptera | Ichneumonidae | Labium species | Wasp | Direct | 1 | 0 | Yes | – | 1 |
Hymenoptera | Vespidae | Species 52 | Wasp | Direct | 1 | 0 | Yes | – | 1 |
Lepidoptera | Lycaenidae | Candalides species | Butterfly | Direct | 1 | 0 | No | – | 1 |
Lepidoptera | Unknown | Species 53 | Moth | DirectA | 1 | 0 | No | – | 1 |
Neuroptera | Unknown | Species 54 | Lacewing | Direct | 3 | 0 | No | – | 1 |
Orthoptera | Unknown | Species 55 | Grasshopper | Direct | 1 | 0 | No | – | 1 |
Polydesmida | Unknown | Species 56 | Millipede | DirectA | 1 | 0 | No | – | 1 |
Blattodea | Termitoidae | Species 57 | Termite | Direct | 1 | 0 | No | – | 1 |
Insects detected visiting flowers of Zieria granulata by human observation only (direct), digital video recordings or time-lapse photography (DVR/TLP) or both methods (both). Captured individuals were examined for pollen grains and found to carry pollen of the target plant species (yes) or not carry pollen (no). The percentage of individuals of a given species that contacted the stigmatic surface was calculated through a behavioural analysis of insects detected on DVR and TLP. As the stigmatic surface was not present in all recordings, visits where the stigma was not visible were excluded from this analysis. ‘Stigma contact’ is the percentage of flowers contacted where the stigma was visible (n is the number of individuals observed). The number of study sites where the species was observed is detailed.
–, no data.
ASpecies detected in nocturnal survey.
BInsects contacting the stigma or carrying pollen were considered potential pollinators.
In all, 18 of the 72 floral visitors contacted the stigma or carried pollen of Z. granulata and, therefore, were classified as potential pollinators (hereafter referred to as pollinators; Table 1). Across all four sites, three species carried pollen and contacted the stigma during foraging bouts, including two bees (A. mellifera and Exoneura species) and a fly (Melangyna species). Four additional species contacted the stigma while foraging, including three flies (Muscidae species 21, Diptera species 28 and Diptera species 29), and an ant (Formicidae species 48). Seventeen species carried pollen on their bodies, including two beetles (Dermestidae species 7 and 8), six flies (Amenia chrysame Walker, 1849, Calliphoridae species 19 and 20, Muscidae species 22, Tachinidae species 26 and Tipulidae species 27), three native bees (Halictus species and Lasioglossum species 49 and 50), two wasps (Labium species and Vespidae species 52) and one ant (Myrmecia species). The ant (Myrmecia species) and wasps (Labium and Vespidae species 52) were each opportunistically captured once and found to carry few pollen grains. Similarly, the ant (Formicidae species 48) contacted the stigma in <1% of floral visits (n = 94). Consequently, these floral visitors are unlikely to be pollinators and were excluded from the logistic regression.
Dipteran and Hymenopteran species were observed as the most frequent floral visitors across both sampling methods. A. mellifera was present in the greatest number of sampling intervals, being present 14% of the time for human observations (Fig. 4). The Hymenopterans, A. mellifera and Exoneura species, were present in a greater number of sampling intervals than any other pollinator detected by human observations (Fig. 4a). A. mellifera was also the most frequent pollinator detected by DVR, being present 33% of the time (Fig. 4b).
Mean (± s.e.) percentage of sampling intervals at all sites where a pollinator species was detected on Zieria granulata by (a) human observations for 5-min intervals (n = 144), or (b) digital video recordings for 2 h intervals (n = 28). Note that the pollinators detected differed between the methods. Standard error bars represent the total variation across the four sites.
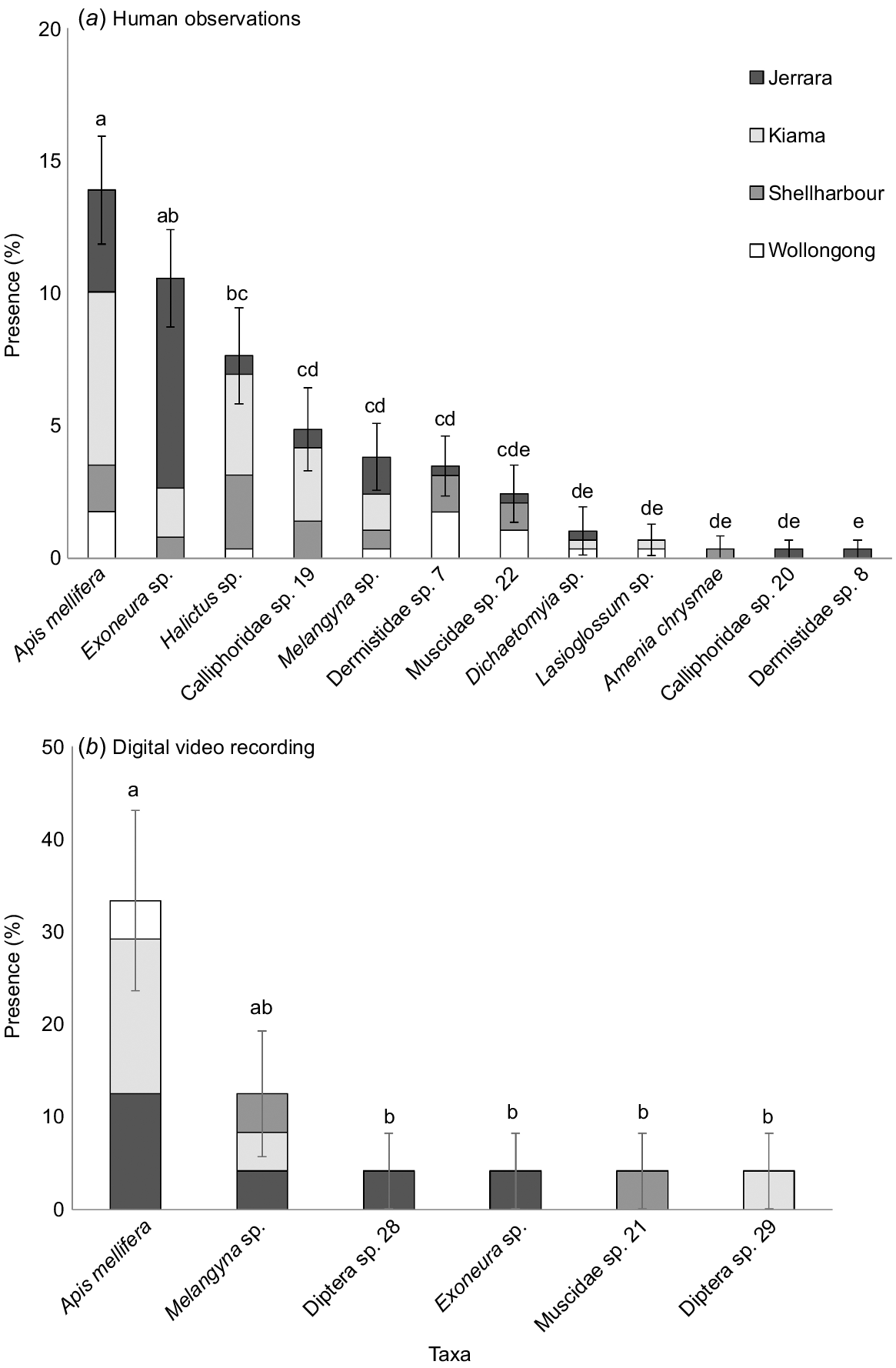
The logistic regression showed that pollinator species compositions varied significantly among sites (Fig. 5). The complete absence of some pollinator taxa from most sites caused complete separation of species in the statistical model, and the site × species interaction term was excluded from the final analysis as a result (Fig. 5). We have provided the analysis of deviance summary outputs both with (Table S3a) and without (Table S3b) the site × species interaction term.
Coefficient plot generated by multi-factor generalised linear model of Zieria granulata pollinator presence in relation to sampling method and site. Estimates for the model are on the logit scale. Error bars represent 95% confidence interval. The reference level for species is Apis mellifera, digital video recording for sampling, and Jerrara for site. Circle, coefficient value; horizontal line, standard error.
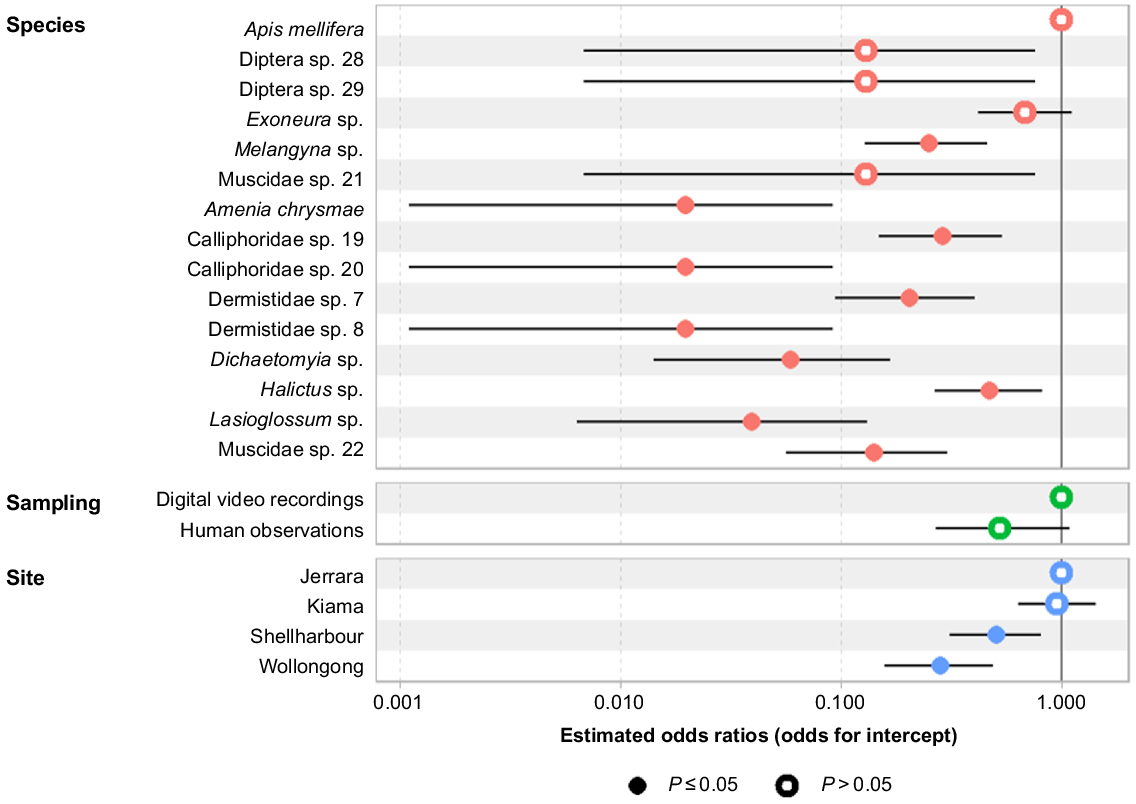
TLP showed that few individuals foraged outside of the primary survey period from 09:00 hours to 15:00 hours (Supplementary material Fig. S1). Three honeybees (A. mellifera) and five unidentified Dipterans were active earlier in the mornings or later in the afternoons (between 07:50 hours and 17:30 hours; Fig. S1). Floral visitors captured on TLP were from the orders Diptera, Hymenoptera and Coleoptera.
Wind pollination
No pollen grains were detected on any of the experimental pollen traps and there were no obvious signs that wind had dislodged pollen from the anthers.
Breeding system
Many replicates were compromised during the pollination experiment by broken branches, loss of pollination bags or death of the entire shrub. After excluding compromised inflorescences from the final analysis, each treatment was replicated between 0 and 9 times per shrub, at each site. The final number of replicates of each treatment at each site is provided in Table S2.
Fruit was produced in all Z. granulata pollination treatments, although fruit production was overall low and the percentage of flowers that produced fruits differed among treatments (2–33%; Fig. 6). Fruit production between the partial bag and open treatment with no bag did not differ significantly (t1,25 = 0.190, P = 0.891), indicating that bags had no substantial effect on fruit production and, therefore, open controls could be legitimately compared with treatments with bags.
Mean (± s.e.) percentage of Z. granulata flowers producing fruit following pollination treatments: (a) all treatments on all plants (outcross n = 24; manipulative selfing n = 28; autonomous selfing n = 63; control n = 48) and (b) a subset of balanced data for two plants at two sites, each containing three replicates of each treatment analysed by a nested three-way ANOVA (n = 2). In each panel, bars surmounted by different letters are significantly different, according to Tukey HSD analysis after ANOVA.
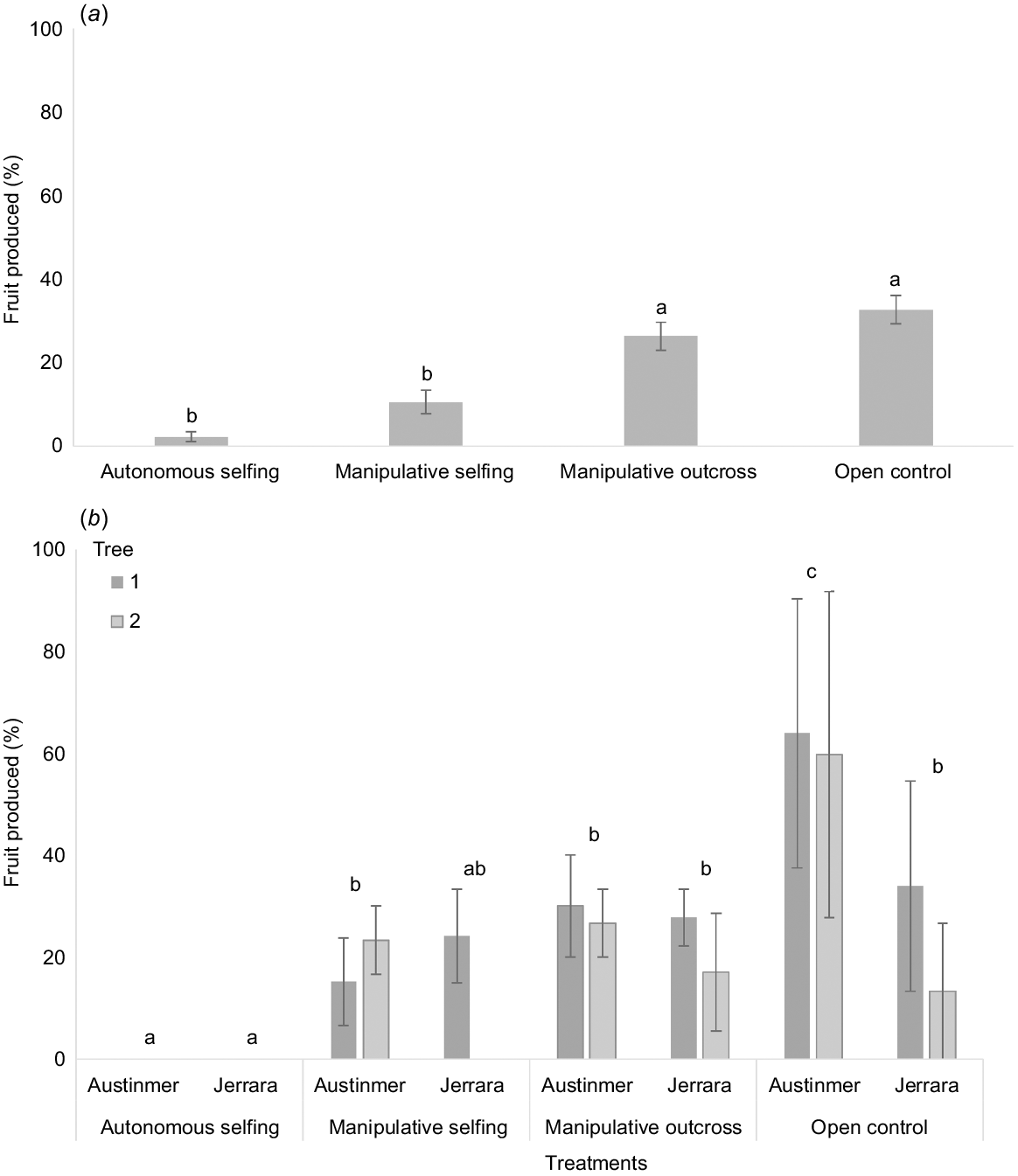
When data from all shrubs and sites were combined, flowers that were self-pollinated (manipulative and autonomous selfing) produced less than half the amount of fruit (2–11%) produced by those that were outcrossed (26–33%; F3,159 = 35.685, P < 0.001; Fig. 6a). There was no significant difference between flowers that received experimental pollen from a different shrub (manipulative outcross) and flowers that were exposed to natural pollinators (open control) (Fig. 6a).
Fruit production within a treatment did not vary significantly among shrubs (0–24%; Table 2, Fig. 6b), but differences among treatments varied significantly between sites (Table 2, Fig. 6b). When flowers were exposed to natural pollinators (open control), fruit production at Austinmer was significantly greater than at Jerrara (Fig. 6b). In contrast, fruit set for the selfing treatments (autonomous and manipulative selfing) did not differ significantly between the sites (Fig. 6b). Importantly, at Austinmer, flowers exposed to natural pollinators (open control) produced significantly more fruit than did flowers that were pollinated with pollen from a different shrub (manipulative outcross), whereas at Jerrara, no significant difference between these treatments was detected (Fig. 6b).
Source | d.f. | d.f. for F | MS | F-ratio | P-value |
---|---|---|---|---|---|
Site: S | 1 | 1, P(S) | 1315.61 | 3.418 | 0.206 |
Plant(site): P(S) | 2 | 2, E | 384.89 | 1.088 | 0.361 |
Treatment: TM | 3 | 3, P(S) × TM | 2561.19 | 34.413 | <0.001 |
S × TM | 3 | 3, P(S) × TM | 591.18 | 7.920 | 0.017 |
P(S) × TM | 6 | 6, E | 74.64 | 0.211 | 0.968 |
Error: E | 16 | 353.76 |
Analysis of fruit production in Zieria granulata at two sites (Wollongong and Kiama) in response to pollination treatments. ‘Plant’ and ‘site’ were treated as random factors (with ‘plant’ nested within ‘site’) and ‘Treatment’ was fixed. Data were arcsine transformed to meet assumptions.
d.f., degrees of freedom.
Many fruits dropped prior to maturation, immediately following a heatwave event (Bureau of Meterology 2020b), and some seed dispersed prior to collection. When the seed dispersed, the fruit remained open on the plant and data on the number of seed per fruit (counted by the presence of cocci which are single-seeded capsules) and insect predation were obtained. No fruit matured when pollen was sourced from the same individual (autonomous or manipulative selfing) and no seed from these treatments was collected for subsequent analysis. The seed dissection results indicated that most seed from all non-selfing treatments was not viable because 89% of seeds from the outcross treatment, and 82% of seeds from the open control treatment were not filled (Table 3). Some seeds from all sites were insect-predated. Overall, 46% of seeds were predated. One insect predator from Jerrara was identified as a parasitic wasp (Megastigmus species).
Treatment | Site | Number of fruit | Number of cocci | Number of seeds | % Filled | % Empty | % Predated |
---|---|---|---|---|---|---|---|
Outcross | Jerrara | 5 | 11 | 10 | 11 | 89 | 55 |
Shellharbour | 0 | 0 | 0 | – | – | – | |
South Kiama | 0 | 0 | 0 | – | – | – | |
Austinmer | 0 | 0 | 0 | – | – | – | |
Total | 11 | 89 | 55 | ||||
Open control | Jerrara | 8 | 14 | 2 | 0 | 100 | 36 |
Shellharbour | 1 | 3 | 3 | 0 | 100 | 100 | |
South Kiama | 14 | 35 | 28 | 56 | 44 | 29 | |
Austinmer | 0 | 0 | 0 | – | – | – | |
Total | 18 | 82 | 55 | ||||
No treatment | Jerrara | 0 | 0 | 0 | – | – | – |
Shellharbour | 0 | 0 | 0 | – | – | – | |
South Kiama | 0 | 0 | 0 | – | – | – | |
Austinmer | 7 | 15 | 15 | 0 | 100 | 27 | |
Total | 0 | 100 | 27 |
Number of fruits and seeds collected per treatment, per site, following application of outcross and control pollination treatments, or no treatment (where fruit were opportunistically collected). The total numbers of cocci and seeds are presented. Note that each coccus bears a single seed; because some seeds were dispersed prior to collection, only data on the coccus were obtained. Dissected seeds were classed as filled or empty. Evidence of insect predation was recorded for all cocci. The total number of seeds in each seed class is presented as a percentage of the seed count below the respective treatment. The total number of predated seeds is presented as a percentage of the total number of cocci examined below the respective treatment. Shrubs were located at Austinmer, Jerrara Dam Reserve (Jerrara), Spring Creek Wetland Kiama (Kiama), Spring Creek Wetland South Kiama (South Kiama) and Killalea Reserve (Shellharbour).
–, no data obtained.
Discussion
Floral biology
The floral biology of Z. granulata indicates that this species best fits the general entomophilous pollination syndrome. Observations of floral phenology demonstrated that Z. granulata is protandrous. Protandrous species are typically self-incompatible (Bertin and Newman 1993), indicating that this species is likely to require a pollen vector for seed set (Bertin and Newman 1993). The highly ornamented pollen wall of Z. granulata suggested that this species is unlikely to utilise an abiotic pollination vector (Halbritter et al. 2018). The heterobrochate structures on the exine wall are key indicators of insect-pollinated plants (Walker 1976; Sannier et al. 2009), whereas the reticulate structures of the exine wall are thought to be indicative of beetles and bees as key pollinators (Faegri and Van der Pijl 1979). The production of nectar provided further evidence for biotic pollination (Dafni 1992) because nectar is the primary reward for many pollinators (Kearns and Inouye 1993) and deceptive or abiotic pollinated plant species do not produce it (Dafni 1992). Nectar production was significantly gender biased towards the female phase, potentially to provide a visitation reward and increase the chance of pollen deposition on a receptive stigma during this pollen-lacking phase. These indicators of an entomophilous pollination syndrome were supported by the study of potential pollinators.
Pollination vectors
This study has highlighted the large discrepancy between the number of floral visitors and the number of likely pollinators of Z. granulata. Visitor assemblages were rich across all study sites, with a total of 72 arthropod species being identified as floral visitors. One quarter (18 of 72) of these species was classed as potential pollinators on the basis of foraging behaviour or pollen load, indicating that seed production in this species is not likely to be limited by pollinator scarcity. Pollinators were predominately from the orders Hymenoptera (bee and wasp) and Diptera (flies), but included a couple of Coleopteran (beetle) species. These taxa, along with the Lepidopterans, are common insect orders observed foraging on Zieria (Auld 2001; Armstrong 2002) and other Rutaceae (Armstrong 1979; Clifford and Drake 1981) flowers in the Greater Sydney region.
This study detected a suite of species that could be mistaken for pollinators if visitation alone was used as the metric for potential pollinators, as was common in earlier studies of pollination vectors of Rutaceae species (e.g. Armstrong 2002; Sgolastra et al. 2016; Pradhan and Devy 2019). Studies that have conducted further analysis to confirm whether species visiting flowers act as pollinators of Australian plants have typically found that a large proportion of visitors do not act as effective pollinators, and thus may limit viable-seed production (Williams and Adam 1994; Boulter et al. 2005; Grant 2020).
Pollinating taxa were variable between the observation methods. A. mellifera was the most frequent visitor detected by both DVR and human observations. However, flies (Melangyna sp. and Diptera sp. 28) were frequent floral visitors detected by DVR, whereas native bees (Exoneura and Halictus species) were frequently detected by human observations. Some taxa may have been deterred by human presence contributing to this variation. Many Dipteran species were identified during human observations, but some species, including Melangyna sp., foraged away from humans during the field surveys. Interestingly, native bees were infrequently captured on DVR, but were present in a large proportion of human observations. Differences in the species richness and presence of pollinating taxa between the methods highlighted the importance of using a combined survey approach when examining plant–pollinator networks. A recent study comparing these two methods (human observations and video recordings) when investigating arthropod plant–pollinator networks found differing but complementary results between the methods (Bonelli et al. 2020). Bonelli et al. (2020) suggested several factors that may contribute to this variation, including difficulties in identifying pollinators detected on DVR to a high taxonomic level, and human presence altering pollinators’ foraging behaviour. Our results align with this study, in that the species richness and visitation frequency of pollinators was variable between the methods, but results from both of the methods were complementary, namely, a broad suite of Hymenopteran and Dipteran species were the main pollinators of Z. granulata.
Hymenopterans were frequently observed visiting flowers by all sampling methods and thus are likely to be the dominant pollinators of Z. granulata. A. mellifera had the greatest pollination potential, on the basis of the greatest visitation frequency detected by both sampling methods and the presence of pollen on body parts. Bees are known to be significant pollinators of native flora (Williams and Adams 1994) and are frequently observed foraging on Rutaceae species (Auld 2001), but their role and function are variable depending on climate, latitude and vegetation structure (Armstrong 1979; Williams and Adams 1994). Four native bee species from the genera Exoneura, Halictus, and Lasioglossum were identified as pollinators of Z. granulata. Exoneura and Halictus species were present in more sampling intervals than either of the Lasioglossum species; however, A. mellifera, a non-native bee, was present in more sampling intervals than any other Hymenopteran. The invasive A. mellifera is thought to compete with native pollinators for floral resources (Pyke and Balzer 1985; Williams and Adam 1994), increase pathogen transmission and change native plant populations and communities (reviewed by Mallinger et al. (2017)). A. mellifera presence has also been correlated with a reduction or absence in Australian native bees. For example, a study on the pollinators of Bursaria spinosa Cav. (Pittosporaceae), a native Australian shrub that exhibits the general entomophily pollination syndrome, with a distribution that overlaps with Z. granulata (PlantNET 2022), found that sites where A. mellifera was dominant had few or no native bees compared with sites with few A. mellifera (Hawkeswood 1990). A recent review on competition between honeybees and Australian native bees found that, generally, A. mellifera posits more negative than positive associations with Australian native bees, but this outcome was variable (see Prendergast et al. 2023). A. mellifera is known to have a larger foraging distance (frequently 6, but up to 9.5 km; Beekman and Ratnieks 2001) than do Australian native bees (the foraging distance of which typically does not exceed 0.5 km; Heard 2016; Smith et al. 2017). Given that Z. granulata habitat is highly fragmented (DPIE 2018) but some populations are located <9 km apart (e.g. Jerrara, Kiama and Kiama South), there is a chance that A. mellifera is visiting several populations where native species cannot. This could benefit Z. granulata by promoting pollen transfer between populations, potentially helping maintain gene flow and prevent inbreeding depression. Investigations into viable-seed production in the presence and absence of A. mellifera would provide a useful insight into the impacts of the invasive pollinator on Z. granulata.
Dipterans were the most species-rich taxon found in our study and were frequently detected foraging on Z. granulata flowers by all survey methods. Dipteran species are known to be significant pollinators in rainforest communities, both in Australia (Williams and Adam 1994) and globally (Vázquez and Simberloff 2002; Smith-Ramírez et al. 2005). This is likely to be a consequence of their morphological traits, including their numerous setae, allowing for a large pollen-carrying capacity (Stavert et al. 2016; Cook et al. 2020). Dipterans are frequently found to play a key role in Australian subtropical plant–pollinator networks, along with other Hymenopterans, Coleopterans, and Lepidopterans (Williams and Adams 1998; Williams 2021). Our findings that both flies and bees were common floral visitors and likely pollinators align with those of other studies on both eastern Australian Rutaceae species (Williams 2021) and Australian rainforest plants (Williams and Adams 1998).
Wind pollination
Given that no pollen was transported in the wind-pollination experiment, it can be concluded that wind is not a likely pollination vector for Z. granulata. More so, field observations showed pollen of this species to be generally sticky, and SEM observations of the pollen found the exine wall to contain heterobrochate structures, traits that are not typical of species relying on an abiotic pollination vector (Walker 1976; Dafni 1992). This result supports inferences of Armstrong (1979) and Auld (2001) that Zieria species and, more broadly, Rutaceae species (Auld 2001; Wilson 2013) are zoophilous.
Breeding system
Z. granulata produced fruit from both self- and outcross-pollination events, which is indicative of a mixed mating system. However, <10% of flowers from the selfing treatments (autonomous or manipulative selfing) produced fruit, and no viable seed was produced from these treatments, indicating that Z. granulata is likely to be an obligate outcrossing species. In his study of 31 Zieria species in NSW (not including Z. granulata), Armstrong (2002) found that 19 species (61%) were self-incompatible, whereas only 12 species (39%) had a mixed mating system. The present study adds an additional species to this dataset and confirms that self-incompatibility is more common than self-compatibility in the Zieria genus.
Fruit production did not differ significantly between the hand-pollinated inflorescences (when pollen was sourced from a different shrub) and inflorescences exposed to natural pollinators (open control). This indicated that the Z. granulata populations studied were not pollen limited in the 2020 flowering season. However, fruit production varied significantly between sites for the open control treatment, indicating that spatial variation affected fruit production throughout the 2020 flowering season. Because of a small sample size, as outlined in the methods, detailed conclusions as to which factors caused variable fruit set within and between sites are not possible; however, we discuss what may have contributed to this spatial variation. Flowers that were exposed to natural pollinators produced twice as many fruits at Austinmer as at Jerrara. This spatial variation in fruit production may be related to the effectiveness of the dominant pollinator at each site, or variation in the size of the populations. A study investigating the effects of population size on fruit set in a Ranunculaceae species over three flowering seasons found population size and fruit set to be significantly and positively related (Molano-Flores and Hendrix 1999). However, Austinmer population contained two mature shrubs, whereas the population at Jerrara contained over 20 shrubs; the result obtained in this study therefore does not support the positive relationship between population size and fruit set found in some other studies (Sih and Baltus 1987; Molano-Flores and Hendrix 1999). Molano-Flores and Hendrix (1999) discussed the possibility of this positive relationship representing a range of factors that are not always statistically or biologically significant. Repeat studies investigating the proportion of flowers that fruit across several populations of varying sizes would aid in understanding whether population size is a factor affecting fruit production, and thus seed set, for Z. granulata.
Alternatively, the spatial variation in fruit production may indicate that environmental factors have influenced pollination and fruit production in these populations. Environmental factors, such as poor nutrient availability, have been shown to directly reduce fruit production and, thus, reproductive success (reviewed by Stephenson (1981)). Following heavy rainfall in the summer of 2021/2022, the production of fruit at Jerrara seemed much improved and fruiting branches that were bagged produced seed with a fill rate of more than 60% (G. Liyanaga, Australian PlantBank, pers. comm.). A laboratory-based experiment comparing fruit and viable-seed production after plants are exposed to environmental stress, such as low carbon, water and nutrient availability, would aid in understanding whether resource limitation is influencing reproductive success in this species.
Pre-dispersal seed predators prevented viable-seed production in 46% of seed examined and some seeds from all study sites had signs of insect predation. In most cases in this study, the predating insects were no longer present when the seeds were inspected, but a single specimen of a Megastigmus species was reared from one seed collected at Jerrara. Phytophagous Megastigmus species have been recorded from several angiosperm families including the Anacardiaceae (Grissell and Prinsloo 2001), Rosaceae (Hernández 2009) and Myrtaceae (Juniper and Britton 2010). However, there are no published records of this genus predating Rutaceae seed. This result suggests that bagging branches with developing fruit to exclude predators may help improve seed yield for conservation or restoration purposes. This simple action appeared to greatly improve the proportion of viable seeds in 2021 (see above); however, no comparison was made to the viability of seeds from unbagged branches, so it is difficult to say whether the improved viability was due solely to bagging, to the increased rainfall, or a combination of both.
Conclusions
This study has shown that Z. granulata is self-incompatible and entomophilous. It is pollinated by a range of insect species, predominantly from the Diptera and Hymenoptera orders. Seed predation was shown to inhibit viable-seed production for all populations examined, but this was not the sole limitation. Spatial variation in fruit production indicates that additional factors such as population size, abiotic conditions or poor gene flow among populations are also contributing to non-viable-seed production in Z. granulata. Ex situ restoration for plant species depends on sourcing viable seed, which has proven to be challenging for this endangered species. The results from our study suggest that the production of viable seed is not pollinator-limited, but may be limited by resource availability, poor gene flow and predation. Seed production for conservation may be improved by bagging developing fruit to exclude predators. Genetic research combined with experimentation to further determine the factors that enhance seed production and survivorship are necessary to protect this endangered species.
Data availability
The data that support this study will be shared on request to the corresponding author.
Acknowledgements
We thank Dr David Britton, Dr Katarina Mikac, Dr Nikolas Johnston, Dr Blake Dawson, Matthew Connors and Rei Asai for assisting with identification of insect specimens; Dr Kevin Mills, Jedda Lemmon and Dr Emma Rooksby for assistance in locating the plant populations used in this study; Dr Rhondda Jones for her invaluable contribution to the statistical analysis; Professor Kristine French, Dr Emily Howells, Dr Mark Ooi, Dr James Camac, and two anonymous reviewers for comments on the manuscript; and Olivia Gobran for help with data collection throughout the flowering season.
References
Armstrong JA (1979) Biotic pollination mechanisms in the Australian flora: a review. New Zealand Journal of Botany 17(4), 467-508.
| Crossref | Google Scholar |
Armstrong JA (2002) The genus Zieria (Rutaceae): a systematic and evolutionary study. Australian Systematic Botany 15, 277-463.
| Crossref | Google Scholar |
Auld T (2001) The ecology of the Rutaceae in the Sydney region of south-eastern Australia: poorly known ecology of a neglected family. Cunninghamia 7, 213-239.
| Google Scholar |
Baskin JM, Baskin CC (2015) Inbreeding depression and the cost of inbreeding on seed germination. Seed Science Research 25, 355-385.
| Crossref | Google Scholar |
Beekman M, Ratnieks FLW (2000) Long-range foraging by the honey-bee, Apis mellifera L. Functional Ecology 14, 490-496.
| Crossref | Google Scholar |
Bennett JM, Thompson A, Goia I, Feldmann R, Ştefan V, Bogdan A, Rakosy D, Beloiu M, Biro I-B, Bluemel S, Filip M, Madaj A-M, Martin A, Passonneau S, Kalisch DP, Scherer G, Knight TM (2018) A review of European studies on pollination networks and pollen limitation, and a case study designed to fill in a gap. AoB Plants 10(6), ply068.
| Crossref | Google Scholar |
Bertin RI, Newman CM (1993) Dichogamy in angiosperms. The Botanical Review 59, 112-152.
| Crossref | Google Scholar |
Bonelli M, Melotto A, Minici A, Eustacchio E, Gianfranceschi L, Gobbi M, Casartelli M, Caccianiga M (2020) Manual sampling and video observations: an integrated approach to studying flower-visiting arthropods in high-mountain environments. Insects 11(12), 881.
| Crossref | Google Scholar |
Boulter SL, Kitching RL, Howlett BG, Goodall K (2005) Any which way will do: the pollination biology of a northern Australian rainforest canopy tree (Syzygium sayeri; Myrtaceae). Botanical Journal of the Linnean Society 149, 69-84.
| Crossref | Google Scholar |
Burd M (1994) Bateman’s principle and plant reproduction: the role of pollen limitation in fruit and seed set. The Botanical Review 60, 83-139.
| Crossref | Google Scholar |
Bureau of Meterology (2020a) Wind rose: Sydney. Available at http://www.bom.gov.au/climate/averages/wind/selection_map.shtml [Verified 8 April 2020]
Bureau of Meterology (2020b) Climate data online: daily maximum temperature Bellambi AWS. Available at http://www.bom.gov.au/jsp/ncc/cdio/weatherData/av?p_nccObsCode=122&p_display_type=dailyDataFile&p_startYear=2020&p_c=-931061151&p_stn_num=068228 [Verified 10 June 2021]
Chen M, Zuo X-A (2019) Effect of pollen limitation and pollinator visitation on pollination success of Haloxylon Ammodendron (C.A.Mey.) bunge in fragmented habitats. Frontiers in Plant Science 10, 327.
| Crossref | Google Scholar |
Cook DF, Voss SC, Finch JTD, Rader RC, Cook JM, Spurr CJ (2020) The role of flies as pollinators of horticultural crops: an Australian case study with worldwide relevance. Insects 11, 341.
| Crossref | Google Scholar |
Gilpin A-M, Denham AJ, Ayre DJ (2017) The use of digital video recorders in pollination biology. Ecological Entomology 42, 383-388.
| Crossref | Google Scholar |
Grissell EE, Prinsloo GL (2001) Seed-feeding species of Megastigmus (Hymenoptera: Torymidae) associated with Anacardiaceae. Journal of Hymenoptera Research 10, 271-279.
| Google Scholar |
Hawkeswood TJ (1990) Insect pollination of Bursaria spinosa (Pittosporaceae) in the Armidale area, New South Wales, Australia. Giornale Italiano di Entomologia 5, 67–-87.
| Google Scholar |
Hermansen TD, Britton DR, Ayre DJ, Minchinton TE (2014) Identifying the real pollinators? Exotic honeybees are the dominant flower visitors and only effective pollinators of Avicennia marina in Australian temperate mangroves. Estuaries and Coasts 37, 621-635.
| Crossref | Google Scholar |
Hernández Á (2009) Field-based evidence of rose seed-infesting wasps Megastigmus aculeatus (Swederus) surviving bird gut passage. Animal Biology 59(2), 189-199.
| Crossref | Google Scholar |
Juniper PA, Britton DR (2010) Insects associated with the fruit of Syzygium paniculatum (Magenta Lillypilly) and Syzygium australe (brush cherry). Australian Journal of Entomology 49(4), 296-303.
| Crossref | Google Scholar |
Knight T, Vamosi JC, Mazer SJ, Burd M, Campbell DR, Dudash MR, Johnston MO, Mitchell RJ, Ashman T-L (2005) Pollen limitation of plant reproduction: pattern and process. Annual Review of Ecology, Evolution, and Systematics 36, 467-497.
| Crossref | Google Scholar |
Linnaeus C (1758) ‘Systema naturae per regna tria naturae, secundum classes, ordines, genera, species, cum characteribus, differentiis, synonymis, locis.’ (Laurentii Salvii: Holmiae). Vol. Tomus I, Edn 10, pp. 1–824. 10.5962/bhl.title.542
Lopresti LC, Sommerville KD, Gilpin A-M, Minchinton TE (2023) Reproductive biology of rainforest Rutaceae: floral biology, breeding systems and pollination vectors of Acronychia oblongifolia and Sarcomelicope simplicifolia subsp. simplicifolia. Cunninghamia 23, 11-26.
| Google Scholar |
Mallinger RE, Gaines-Day HR, Gratton C (2017) Do managed bees have negative effects on wild bees?: a systematic review of the literature. PLoS ONE 12(12), e0189268.
| Crossref | Google Scholar |
Martyn Yenson AJ, Offord CA, Meagher PF, Auld T, Bush D, Coates DJ, Commander LE, Guja LK, Norton SL, Makinson RO, Stanley R, Walsh N, Wrigley D, Broadhurst L (2021) ‘Plant Germplasm Conservation in Australia: strategies and guidelines for developing, managing and utilising ex situ collections. 3rd edition.’ (Australian Network for Plant Conservation: Canberra, ACT, Australia)
Martyn AJ, Seed LU, Ooi MK, Offord CA (2009) Seed fill, viability and germination of NSW species in the family Rutaceae. Cunninghamia 11, 203-212.
| Google Scholar |
Molano-Flores B, Hendrix SD (1999) The effects of population size and density on the reproductive output of Anemone canadensis L. (Ranunculaceae). International Journal of Plant Sciences 160(4), 759-766.
| Crossref | Google Scholar |
Morrant DS, Schumann R, Petit S (2009) Field methods for sampling and storing nectar from flowers with low nectar volumes. Annals of Botany 103, 533-542.
| Crossref | Google Scholar |
PalDat (2020) PalDat – a palynological database. Available at www.paldat.org [verified 24 November 2020]
PlantNET (2022) PlantNET (The NSW Plant Information Network System). (Royal Botanic Gardens and Domain Trust: Sydney, NSW, Australia). Available at https://plantnet.rbgsyd.nsw.gov.au [Verified 25 February 2022]
Pradhan U, Devy MS (2019) Pollinators of Sikkim Mandarin Orange Citrus reticulata (Sapindales: Rutaceae). Journal of Threatened Taxa 11, 13625-13628.
| Crossref | Google Scholar |
Prendergast KS, Dixon KW, Bateman PW (2023) The evidence for and against competition between the European honeybee and Australian native bees. Pacific Conservation Biology 29, 89-109.
| Crossref | Google Scholar |
R Core Team (2022) ‘R: a language and environment for statistical computing.’ (R Foundation for Statistical Computing: Vienna, Austria). Available at https://www.R-project.org/
Sannier J, Baker WJ, Anstett M-C, Nadot S (2009) A comparative analysis of pollinator type and pollen ornamentation in the Araceae and the Arecaceae, two unrelated families of the monocots. BMC Research Notes 2, 145-149.
| Crossref | Google Scholar |
Sgolastra F, Fisogni A, Quaranta M, Bogo G, Bortolotti L, Galloni M (2016) Temporal activity patterns in a flower visitor community of Dictamnus albus in relation to some biotic and abiotic factors. Bulletin of Insectology 69(2), 291-300.
| Google Scholar |
Sih A, Baltus MS (1987) Patch size, pollinator behavior, and pollinator limitation in catnip. Ecology 68, 1679-1690.
| Google Scholar |
Smith-Ramírez C, Martinez P, Nuñez M, González C, Armesto JJ (2005) Diversity, flower visitation frequency and generalism of pollinators in temperate rain forests of Chiloé Island, Chile. Austral Ecology 28, 53-60.
| Crossref | Google Scholar |
Smith JP, Heard TA, Beekman M, Gloag R (2017) Flight range of the Australian stingless bee Tetragonula carbonaria (Hymenoptera: Apidae). Austral Entomology 56(1), 50-53.
| Crossref | Google Scholar |
Sommerville KD, Clarke B, Keppel G, McGill C, Newby Z-J, Wyse SV, James SA, Offord CA (2017) Saving rainforests in the South Pacific: challenges in ex situ conservation. Australian Journal of Botany 65, 609-624.
| Crossref | Google Scholar |
Stavert JR, Liñán-Cembrano G, Beggs JR, Howlett BG, Pattemore DE, Bartomeus I (2016) Hairiness: the missing link between pollinators and pollination. PeerJ 4, e2779.
| Crossref | Google Scholar |
Stephenson AG (1981) Flower and fruit abortion: proximate causes and ultimate functions. Annual Review of Ecology and Systematics 12, 253-279.
| Crossref | Google Scholar |
Vázquez DP, Simberloff D (2002) Ecological specialization and susceptibility to disturbance: conjectures and refutations. American Naturalist 159, 606-623.
| Crossref | Google Scholar |
Williams G, Adam P (1994) A review of rainforest pollination and plant-pollinator interactions with particular reference to Australian subtropical rainforests. Australian Zoologist 29, 177-212.
| Crossref | Google Scholar |
Williams GA, Adam P (1998) Pollen loads collected from large insects in Australian subtropical rainforests. Proceedings- Linnean Society of New South Wales 120, 49-67.
| Google Scholar |
Williams G, Adam P (2001) The Insect Assemblage Visiting the Flowers of the Subtropical Rainforest Pioneer Tree Alphitonia Excelsa (Fenzl) Reiss. Ex Benth. (Rhamnaceae). Proceedings of the Linnean Society of New South Wales 123, 235-259.
| Google Scholar |