Plasma-activated water’s potential contribution to ‘One Health’
Bjoern H. Kolbe A , Bryan Coad B C and Katharina Richter A D *A
B
C
D
![]() Bjoern Hendrik Kolbe is a PhD student at The University of Adelaide in the research group of Dr Katharina Richter. His background is in applied material science and dental technologies. Based on his previous experience in plasma technology, he currently researches plasma-activated water to combat foodborne pathogens. |
![]() Dr Bryan Coad is a Senior Research Fellow at The University of Adelaide. His background is in physical chemistry. His areas of research interest include biomaterials and plasma processes. |
![]() Dr Katharina Richter is a biomedical researcher with global work stints in Germany, New Zealand, Switzerland, Denmark, Belgium, Netherlands and Australia. A trained pharmacist she completed her PhD in medicine and applied microbiology in 2017 and started her own research group in 2019 at The University of Adelaide. Her group has two priorities: (i) developing new treatments against infections with antibiotic-resistant bacteria and (ii) improving health literacy of the society by effective science communication through public speaking, science outreach and STEM workshops at schools. |
Abstract
Foodborne pathogens cause a major burden to public health and the economy, costing A$2.44 billion, and causing 48,000 hospitalisations annually in Australia. With an increasing global impact of foodborne illnesses and the emergence of antibiotic-resistant pathogens, new decontamination technologies should consider the One Health approach to human, animal and environmental health. This review explores the application of plasma-activated water (PAW) as a novel sanitisation method. We discuss the implications of adopting PAW as an environmentally friendly and cost-effective sanitiser through a multidisciplinary One Health perspective. The findings underscore the promising role of PAW in mitigating foodborne pathogens, offering a holistic solution that aligns with the principles of One Health for enhanced food safety and public health.
Keywords: antimicrobial resistance, cold plasma, decontamination, food safety, foodborne pathogens, plasma-activated liquids, sanitiser.
Introduction
Foodborne pathogens cost A$2.44 billion annually in Australia due to increased mortality, healthcare costs or lost workforce.1 The World Health Organisation reports 600 million foodborne illnesses worldwide, leading to 420,000 deaths annually.2 The main pathogens responsible for these diseases include Listeria, Salmonella, Campylobacter and Escherichia coli.3
Overuse or misuse of antibiotics and chemical sanitisers in the food supply chain can cause antimicrobial resistance (AMR), which is a significant threat to public health. These resistances reduce the effectiveness of antimicrobial agents in different areas, such as commonly applied decontamination strategies and even ‘last resort’ antibiotics.4
The One Health approach addresses the complex interconnectedness of human, animal and environmental health, especially in the context of food safety, to reduce the risk of emerging resistance (Fig. 1). The concept encourages multidisciplinary solutions through the targeted use of antibiotics, pesticides and sanitisers, as well as general guidelines for health practitioners, veterinarians and the food industry.5
The One Health concept for addressing the interconnectedness of human, animal and environmental health, to reduce the risk of emerging microbial resistance.
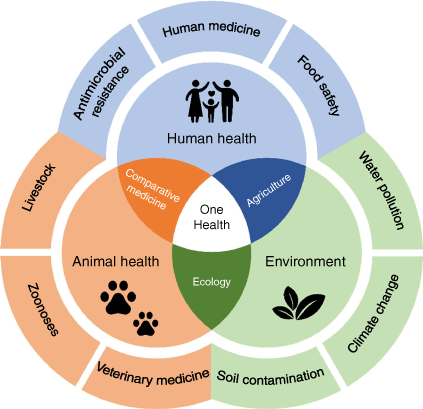
Sanitisers are typically chemical-based and can lead to adverse effects, such as chemical residues in food and environmental pollution.6 Eco-friendly methods are heat or UV treatment, however, these methods are often unsuitable for low processed food as they can induce changes to sensory attributes like colour, smell or texture, leading to a demand for novel eco-friendly sanitisers. Cold plasma technology is a non-thermal solution that shows promise in addressing these challenges. Recent approaches even facilitate cold plasma to generate sanitisers based on liquids like water or aqueous solutions.
Plasma and plasma-activated water
Plasma can be generated by the application of energy to gases that then form a new state of matter. The matter is composed of myriad reactive species (ions, electrons and free radicals) including reactive oxygen and nitrogen species (RONS) that have antimicrobial properties.7 Thus, water treated with plasma, so-called plasma-activated water (PAW), contains short and long-lived reaction products like superoxide (), nitric oxide (NO*), peroxynitrite (ONOO−), hydrogen peroxide (H2O2), ozone (O3), nitrite (NO2) and nitrate (NO3).4 These dissolved antimicrobial species are active against foodborne pathogens like Escherichia coli, Listeria, Campylobacter and Salmonella.3,4 This makes PAW a promising candidate for investigation in food sanitising and food safety applications. Additionally, PAW could be used as fertiliser in agriculture due to the presence of various nitrogen compounds (Fig. 2).
Combination of water and plasma. Reactions of the chemical species form reactive oxygen and nitrogen species (RONS), leading to antimicrobial and fertilising properties in plasma-activated water.
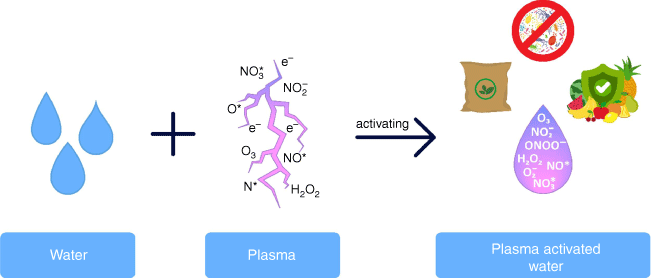
The properties of PAW are affected by the type of generator and configurations employed for plasma generation. Most important properties include the pH, oxidation–reduction potential and conductivity, which can be altered by selecting appropriate feeding gases and discharge parameters. The physicochemically diverse composition enables PAW to target multiple microbial components, including microbial membranes, enzymes and DNA.8 These simultaneous reactions result in rapid microbial inactivation and reduced possibility of emerging AMR.8
Further tailoring of the physicochemical properties allows for various applications in the food industry, including decontamination of surface contact areas and equipment, biofilm degradation and disinfection of food.3,4 The adjustable properties of PAW additionally allow for applications in agriculture, water purification and pesticide degradation (Fig. 3).9–11
Applications of PAW in the food and agricultural industry
In the food industry, the antimicrobial properties of PAW have been demonstrated for foodborne pathogens like Escherichia coli, Salmonella, Listeria and Campylobacter, where log10 reductions of colony forming units (CFU) for planktonic bacteria are reported as 5.4, 3.9, 5.4 and 4.2 respectively.12–15
Bacteria survive harsh environments by forming biofilms, made from extracellular polymeric substances. The biofilm provides shelter against antibiotics and decontamination strategies and reduces the effectiveness of sanitisers and decontamination treatments drastically. Even for biofilm-forming bacteria, PAW achieves efficient decontamination, as reports show log10CFU reductions of up to 5.4, 3.9 and 5.4 for E. coli, Salmonella and Listeria respectively.15–17 Previous studies demonstrated the food sanitising capacity of PAW for fresh fruits and vegetables, nuts, meat, fish, poultry and eggs (Table 1).
Microorganism | Food | Treatment | log10 CFU reduction | References | |
---|---|---|---|---|---|
E. coli | Chicken muscle | Specimens were immersed in PAW for 30, 45 and 60 min at 4, 25 and 40°C | 0.46 CFU mL–1 | 18 | |
Iceberg lettuce | Specimens were immersed in PAW for 1, 3 and 5 min | 1.8–6.1 CFU mL–1 | 19 | ||
Mung bean sprouts | Specimens were immersed in PAW for 1, 3 and 5 min | 2.5–3.5 CFU mL–1 | 19 | ||
Cucamelon | Specimens were immersed in PAW for 2 min | 3 CFU g–1 | 8 | ||
Fresh beef | Spray treatment with PAW, and stored at 4°C for up to 24 days | 2 CFU g–1 over 13 days of storage, >5 CFU g–1 over 24 days of storage | 20 | ||
Potatoes | Specimens were immersed in PAW for 10 min | 3.78 CFU mL–1 | 21 | ||
Listeria | Beef jerky | Beef slices were cured in PAL for 18–20 h at 4°C | 0.85 CFU g–1 in jerky | 22 | |
Lettuce | Specimens were immersed in water and plasma treated for 5 min | 2.4 CFU g–1 | 23 | ||
Cucamelon | Specimens were immersed in PAW for 2 min | 3 CFU g–1 | 8 | ||
Mackerel | Specimens were placed on PAW ice with 1.0% lactic acid for 120 min | 4.53 CFU mL–1 | 24 | ||
Salmonella | Egg shells | Eggs were immersed in PAW for 60 s | >4 log CFU egg–1 | 25 | |
Cucamelon | Specimens were immersed in PAW for 2 min | 3 CFU g–1 | 8 | ||
Fresh beef | Specimens were immersed in PAW with 0.20% lactic acid for 20 min | 3.45 CFU mL–1 | 26 | ||
Staphylococcus aureus | Strawberries | Specimens were Immersed in PAW for 5, 10 and 15 min and stored at 20°C for 4 days | 1.6–2.3 CFU g–1 at day 0 | 27 | |
1.7–3.4 CFU g–1 at day 4 |
PAW treatment has the benefit of minor or no effect on sensory attributes of foods, as shown for chicken breast, bean sprouts, cucamelons and beef.8,19,20 Recent application of PAW as a nitrate rich process media for beef jerky highlighted these effects in comparison to conventional processing, while maintaining the desired red colour of the treated beef.22 The nitrogen compounds and their easier bioavailability, due to high reactivity, in PAW can additionally aid crop fertilisation. Current investigations of PAW revealed further benefits to agriculture applications for seed germination, plant growth and plant health.11
Other applications utilise the incorporated reactivity of PAW or the induced modifications of treated substrates. For example, the processing of crop materials like starch and proteins with PAW results in enhanced water-binding capabilities, aggregation and gelation.28
Additionally, the general reactivity of PAW allows for wastewater purification and pesticide degradation, while producing no toxic residues.10 Other applications as a thawing agent for meat and fish products to reduce bacterial contamination highlight the potential for innovative approaches for PAW besides the main use as sanitising agent.13,24
PAW and One Health
The ‘One Health’ framework recognises that diseases affecting animals can also affect human health, and emphasises the need for a unified strategy in mitigating foodborne threats.5 In this context, the application of PAW as an innovative, sanitisation technology promotes food safety through potent pathogen mitigation while considering the broader effects on animal welfare and environmental sustainability.
Common food sanitisers used against foodborne pathogens often contain chemicals like chlorine, quaternary ammonium compounds, bromine or iodine, which leave residues that are harmful to both human health and the environment. Therefore, gentler and more sustainable methods are desired.29 PAW is promising because its antimicrobial properties mainly derive from the action of RONS. This eliminates the need for harmful chemicals and reduces the burden on the environment resulting in improved eco-friendly food processing. Additionally, initial studies highlighted the interaction between RONS and inherent reactive species, resulting in enhanced antimicrobial properties compared to pure chemical-based sanitisers.8
In addition to the burden that sanitisers place on the environment, their residues and accumulation contribute to the risk of emerging resistance. Owing to shared mechanisms of action with antibiotics, this could lead to compromised effectiveness of antibiotics in human and animal health.30 The evidence of increased tolerance of agricultural microbiota against common antibiotics, like ciprofloxacin and kanamycin highlights the problem of possible development of resistance caused by residuals in the environment.30 Additionally, RONS are non-specific and target the membrane of bacteria, reducing the risk of emerging resistance.
Concerns of reduced agricultural output or possible pollution of soil through chemical fertilisers should be addressed regarding One Health. The demonstrated benefits of PAW’s fertilising properties related to RONS and contained nitrates could allow for the replacement of other chemical fertilisers; thereby placing less burden on soil, as well as ensuring agricultural output, while simultaneously reducing pollution in water and soil.5
Recent studies of PAW treatment of pesticides, like carbendazim and chlorpyrifos, have shown effective reduction by respectively 80 and 65% within 30–60 min. Furthermore, the simultaneous reduction of bacterial load and elimination of fungal spores could be proven.9,10 The incorporation of PAW in the food supply chain could thereby reduce pesticide residues, while simultaneously decreasing the risk of forming resistance.9
In comparison to soil, wastewater is similarly affected by sanitisers, pesticides and fertiliser residues. Therefore, efficient wastewater treatment is needed to accomplish the vision of One Health. Recent results of PAW application for in-line water treatment showed efficient methylene-orange degradation.10 Further scale-up of this application could contribute to clean water supply and improved waste management.
As various disciplines work together for the One Health approach, effective controls pre- and post-harvest are crucial to effectively reduce the risk of contamination throughout the food supply chain. Therefore, protocols for PAW application should be developed to ensure proper use of this novel sanitiser. Furthermore, regulatory criteria should be developed for the successful application of PAW, as to our knowledge PAW is not yet approved by authorities like the World Health Organization or US Food and Drug Administration. These guidelines would ensure optimal performance for different applications.5
The remaining challenges are the necessary quality attributes and limitations to batch production of PAW with volumes of up to 520 L h–1. Addressing these limitations would enable an efficient utilisation of PAW as a commercial-scale sanitiser within the food industry.13,29
Conclusion
The application of PAW as food sanitiser, fertiliser and degradation agent for environmental pollutants could positively contribute to One Health. Besides foodborne pathogen reduction, PAW could efficiently reduce the risk of AMR within food production due to the rapid and synergistic antimicrobial reactions, addressing the unmet need for novel antimicrobials. However, further research is required to study the potential interactions of PAW with pollutants and to prove its non-toxicity before regulatory approval could enable industry application of this novel sanitiser.
Data availability
Data sharing is not applicable as no new data were generated or analysed during this study.
Declaration of funding
This research was funded by The University of Adelaide, Australia. B. H. Kolbe received an Australian Government Research Training Program scholarship. K. Richter received a Future Making Fellowship by the Division of Research and Innovation, The University of Adelaide. Further support has been provided by the Australian National Health and Medical Research Council (2018/GNT1163634) and Medical Research Future Fund (2022/MRF2023153).
Acknowledgements
The authors thank Dr Adrian Abdo and Anja Zelmer for scientific feedback, and Stefania Lebedev for support in creating the professional graphics.
References
1 Glass K et al. (2023) The cost of foodborne illness and its sequelae in Australia circa 2019. Foodborne Pathog Dis 20(10), 419-426.
| Crossref | Google Scholar | PubMed |
2 The Food and Agribuisness Growth Centre (2021) The National Food Waste Strategy Feasibility Study – Final Report. FIAL. https://workdrive.zohopublic.com.au/external/06152b9ff5971843391f39fc4d32a847e56fb907c167a4a645887b0a4bc43000 (accessed 12 March 2024)
3 Abdo A et al. (2023) Efficacy of plasma-treated water against Salmonella typhimurium: antibacterial activity, inhibition of invasion, and biofilm disruption. Antibiotics 12, 1371.
| Crossref | Google Scholar | PubMed |
4 Han QY et al. (2023) Application of plasma-activated water in the food industry: a review of recent research developments. Food Chem 405, Part A, 134797.
| Crossref | Google Scholar | PubMed |
5 Garcia SN et al. (2020) One Health for food safety, food security, and sustainable food production. Front Sustain Food Syst 4, 1.
| Crossref | Google Scholar |
7 Das S et al. (2022) Role of cold atmospheric plasma in microbial inactivation and the factors affecting its efficacy. Health Sci Rev 4, 100037.
| Crossref | Google Scholar |
8 Rothwell JG et al. (2023) An effective sanitizer for fresh produce production: in situ plasma-activated water treatment inactivates pathogenic bacteria and maintains the quality of cucurbit fruit. Microbiol Spectr 11(4), e00034-23.
| Crossref | Google Scholar | PubMed |
9 Sawangrat C et al. (2022) Application of pinhole plasma jet activated water against Escherichia coli, Colletotrichum gloeosporioides, and decontamination of pesticide residues on chili (Capsicum annuum L.). Foods 11(18), 2859-2876.
| Crossref | Google Scholar | PubMed |
10 Gao Y et al. (2022) Microbubble-enhanced water activation by cold plasma. Chem Eng J 446, 137318.
| Crossref | Google Scholar |
11 Guo D et al. (2021) Plasma-activated water production and its application in agriculture. J Sci Food Agric 101(12), 4891-4899.
| Crossref | Google Scholar | PubMed |
12 Große-Peclum V et al. (2023) Using TRIS-buffered plasma-activated water to reduce pathogenic microorganisms on poultry carcasses with evaluation of physicochemical and sensory parameters. Foods 12(5), 1113.
| Crossref | Google Scholar | PubMed |
13 Xiang Q et al. (2022) A review on recent advances in plasma-activated water for food safety: current applications and future trends. Crit Rev Food Sci Nutr 62(8), 2250-2268.
| Crossref | Google Scholar | PubMed |
14 Traylor MJ et al. (2011) Long-term antibacterial efficacy of air plasma-activated water. J Phys D Appl Phys 44(47), 472001.
| Crossref | Google Scholar |
15 Fernández-Gómez P et al. (2023) Susceptibility and transcriptomic response to plasma-activated water of Listeria monocytogenes planktonic and sessile cells. Food Microbiol 113, 104252.
| Crossref | Google Scholar | PubMed |
16 Smet C et al. (2019) Inactivation of single strains of Listeria monocytogenes and Salmonella typhimurium planktonic cells biofilms with plasma activated liquids. Front Microbiol 10, 1539.
| Crossref | Google Scholar | PubMed |
17 Xia B et al. (2023) The importance of superoxide anion for Escherichia coli biofilm removal using plasma-activated water. J Environ Chem Eng 11(3), 109977.
| Crossref | Google Scholar |
18 Royintarat T et al. (2020) Chemical-free and synergistic interaction of ultrasound combined with plasma-activated water (PAW) to enhance microbial inactivation in chicken meat and skin. Sci Rep 10(1), 1559.
| Crossref | Google Scholar | PubMed |
19 Schnabel U et al. (2015) Decontamination of fresh-cut iceberg lettuce and fresh mung bean sprouts by non-thermal atmospheric pressure plasma processed water (PPW). Mod Agric Sci Technol 1(1), 23-39.
| Crossref | Google Scholar |
20 Zhao Y et al. (2020) Plasma-activated water treatment of fresh beef: bacterial inactivation and effects on quality attributes. IEEE Trans Radiat Plasma Med Sci 4(1), 113-120.
| Crossref | Google Scholar |
21 Lim J et al. (2021) Effect of post‐discharge time of plasma‐treated water (PTW) on microbial inactivation and quality of fresh‐cut potatoes. J Food Process Preserv 45(5), e15387.
| Crossref | Google Scholar |
22 Inguglia ES et al. (2020) Plasma-activated water as an alternative nitrite source for the curing of beef jerky: influence on quality and inactivation of Listeria innocua. Innov Food Sci Emerg Technol 59, 102276.
| Crossref | Google Scholar |
23 Patange A et al. (2019) Efficacy of cold plasma functionalised water for improving microbiological safety of fresh produce and wash water recycling. Food Microbiol 84, 103226.
| Crossref | Google Scholar | PubMed |
24 Jyung S et al. (2023) Inactivation of Listeria monocytogenes through the synergistic interaction between plasma-activated water and organic acid. Food Res Int 167, 112687.
| Crossref | Google Scholar | PubMed |
25 Lin CM et al. (2019) The optimization of plasma-activated water treatments to inactivate Salmonella enteritidis (ATCC 13076) on shell eggs. Foods 8(10), 520.
| Crossref | Google Scholar | PubMed |
26 Qian J et al. (2019) Action of plasma-activated lactic acid on the inactivation of inoculated Salmonella enteritidis and quality of beef. Innov Food Sci Emerg Technol 57, 102196.
| Crossref | Google Scholar |
27 Ma R et al. (2016) Effect of non-thermal plasma-activated water on fruit decay and quality in postharvest Chinese bayberries. Food Bioprocess Technol 9(11), 1825-1834.
| Crossref | Google Scholar |
28 Oliveira M et al. (2022) Plasma-activated water: a cutting-edge technology driving innovation in the food industry. Food Res Int 156, 111368.
| Crossref | Google Scholar | PubMed |
29 Herianto S et al. (2021) Nonthermal plasma-activated water: a comprehensive review of this new tool for enhanced food safety and quality. Compr Rev Food Sci Food Saf 20(1), 583-626.
| Crossref | Google Scholar | PubMed |
30 Malagón-Rojas JN et al. (2020) From environment to clinic: the role of pesticides in antimicrobial resistance. Rev Panam Salud Publica 44, e44.
| Crossref | Google Scholar |
![]() Bjoern Hendrik Kolbe is a PhD student at The University of Adelaide in the research group of Dr Katharina Richter. His background is in applied material science and dental technologies. Based on his previous experience in plasma technology, he currently researches plasma-activated water to combat foodborne pathogens. |
![]() Dr Bryan Coad is a Senior Research Fellow at The University of Adelaide. His background is in physical chemistry. His areas of research interest include biomaterials and plasma processes. |
![]() Dr Katharina Richter is a biomedical researcher with global work stints in Germany, New Zealand, Switzerland, Denmark, Belgium, Netherlands and Australia. A trained pharmacist she completed her PhD in medicine and applied microbiology in 2017 and started her own research group in 2019 at The University of Adelaide. Her group has two priorities: (i) developing new treatments against infections with antibiotic-resistant bacteria and (ii) improving health literacy of the society by effective science communication through public speaking, science outreach and STEM workshops at schools. |