The effect of grazing management and legumes on soil organic carbon stocks in pastoral systems relevant to the Australian Carbon Credit Unit Scheme: a critical review
Alice Debney A and Lisa Lobry de Bruyn
A
Abstract
Soil carbon dynamics and the potential of management to influence its sequestration and storage is receiving global recognition. The Australian federal government’s emissions trading scheme includes a list of eligible activities whereby a landholder can earn Australian Carbon Credit Units through documenting soil carbon stocks over time. A systematic literature review was undertaken to establish the nature and geographical spread of evidence on the impact of grazing management and legume inclusion in pastoral systems (two of the eligible management activities) on soil carbon stocks. A small number of eligible research articles were identified in Australia (n = 14) from the global literature. Published studies were mainly in New South Wales and Queensland (from 2010 to 2020) on a variety of soil types across all climatic zones. In the majority of studies, there was limited ability to determine the effect of the eligible activities on soil carbon stock due to experimental design and one-off sampling. The legume studies (n = 3) that did show accumulation of soil organic carbon (SOC) had sampled SOC stocks before and after treatment imposed, accounted for equivalent soil mass, and were all conducted on a research station. Interpreting the impacts of management was hindered by insufficient documentation of grazing management history that was often confounded by the biophysical drivers of soil carbon dynamics, and reporting SOC stocks for a fixed soil depth (50% of studies). An investment in resampling those sites sampled more than 10 years ago in close proximity to original sampling location could provide greater insight into the expected levels of SOC accumulation.
Keywords: agriculture, carbon sequestration, Clean Energy Regulator, climate change mitigation, Emissions Reduction Fund, farmers, grazing management, legumes, soil carbon.
Introduction
Soil carbon dynamics and the potential to influence its sequestration and storage through management is receiving global recognition (Lal et al. 2015, 2021a). The Synthesis Report of the Intergovernmental Panel on Climate Change Fifth Assessment Report has found that human-induced climate change is real, with recent changes having had widespread impacts on humans and natural systems that are unprecedented, with the biggest impacts being diminishing amounts of snow and ice and rising sea levels (Pachauri and Meyer 2014). As a result, research is now focused both nationally and internationally on strategies to reduce net greenhouse gas (GHG, including carbon dioxide (CO2), methane (CH4), nitrous oxide (N2O), sulfur hexafluoride (SF6), and specified kinds of hydrofluorocarbons and perfluorocarbons) emissions. Agriculture in Australia produces an estimated 16% of GHG emissions (Young et al. 2009) and contributes globally between 20 and 25% of GHG emissions (Verschuuren 2017). However, as agriculture is a major land-use sector in Australia, occupying 54% of Australia’s land mass, it is also well placed to offset GHG emissions through sequestration of carbon in trees and soil.
Australia’s federal government set up an emission-trading scheme called the Clean Energy Act 2011, which took effect on 1 July 2012 (Federal Register of Legislation 2012). This legislation covered roughly 50% of Australia’s emissions in the energy and major industrial activities sector, but did not include GHG emissions from agriculture, forestry, land use, and landfills. Other GHG emissions are measured as kilotonnes of carbon dioxide equivalence (CO2-e). The quantity of other GHG can be expressed as CO2-e by multiplying the amount of the GHG by its global warming potential (GWP). For example, if 1 kg of methane is emitted, this can be expressed as 29.8 kg of CO2-e (1 kg CH4 × 29.8 (GWP) = 29.8 kg CO2-e) (IPPC 2021). Due to increased complexities and political influence on these sectors (Macintosh and Waugh 2012), a separate system was designed and implemented through the Carbon Credits (Carbon Farming Initiative) Act 2011 (Federal Register of Legislation 2012), leading to the issuance of Australian Carbon Credit Units (ACCUs), which are equal to 1 tonne of carbon dioxide equivalent (Verschuuren 2017). The latest method that enables increases in soil organic carbon (SOC) stocks in agricultural systems to be traded as ACCUs is the Estimating Soil Organic Carbon Sequestration Using Measurement and Models method, released in December 2021 (Federal Register of Legislation 2021). In early 2022, Professor Macintosh, a director of research at the Australian National University Law School, controversially suggested ACCUs issued for vegetation-based projects of being ‘fraudulent’ because up to 80% of those already issued were flawed (Greber 2022). These claims were largely directed at ACCUs issued for vegetation-based projects, not soil carbon projects. Nevertheless, reservations do exist on the contribution of soil carbon projects for climate change mitigation, despite the rigour of the method used to estimate SOC accumulation, and may well influence future policy on carbon sequestration in agriculture (Minasny et al. 2023; Simmons et al. 2023).
Soil organic carbon sequestration, in those areas where it has the greatest potential, can provide Australian farmers with an additional revenue stream or be used to inset their own emissions while also improving the capacity of soil for plant growth and therefore productivity (Fitch et al. 2022). Aside from the potential additional revenue stream that farmers and landowners can access through the Carbon Farming Initiative, SOC provides numerous benefits to agricultural production systems. The importance of soil in the functioning of the planet has been made, and the role it performs in climate mitigation, food production, soil health, carbon sequestration, and other ecosystem services (Lal et al. 2021b; Kopittke et al. 2022, 2023). These functions relate to the soil as an ecosystem able to support a diverse set of biota, with the ability to take in water, cycle nutrients and organic matter, buffer against toxins, and protect it from disruptive forces such as erosion and compaction. Biogeochemical cycling results in enhanced nutrient availability to plants that are released as a result of microbial activity and enhances soil structure as these microbes produce resins and gums that assist with the binding of soil particles into stable soil aggregates. Improved soil structure increases plant available water capacity and allows air, water, and plant roots to move more easily through the soil, and protects the soil from erosion by wind or water, which is a major source of SOC loss. Soil organic matter, and by association SOC, improves soil biological activity and diversity by providing a food source for soil biota. The soil biota recycle nutrients, improve soil structure, and can suppress crop diseases. Finally, SOC can lessen the effect of harmful toxins and heavy metals as well as assisting degradation of pesticides, thereby providing buffering capacity (Petersen and Hoyle 2016).
In the latest release (as at May 2022) of the Agricultural Commodities, Australia, report for the 2019–20 financial year, 325 million of the 377 million hectares (86%) of agricultural land were used for grazing (Australian Bureau of Statistics 2021). Grazing is undertaken in a variety of climatic zones and soil and land types, from rangelands to higher rainfall zones (Fig. 1). Rangeland grazing systems are low input, extensively managed enterprises. Higher input grazing systems tend to be in the higher rainfall, more fertile soil, and higher pasture production regions closer to the coast.
Approximate study locations of articles reviewed and climate zones in Australia (source: Bureau of Meteorology 2023, using GDA94 geographic coordinate system).
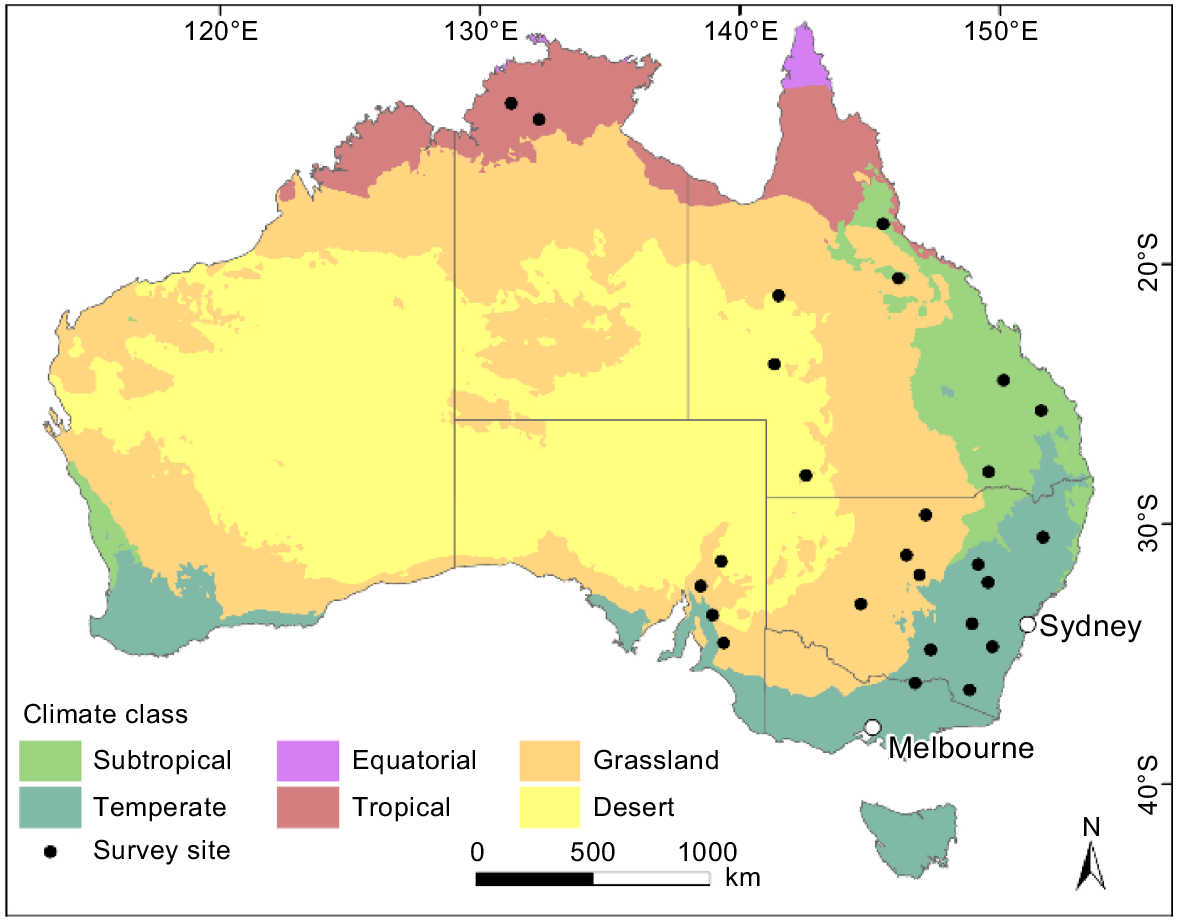
Two of the eligible management activities under the 2021 Estimating Soil Organic Carbon Sequestration Using Measurement and Models method are ‘altering the stocking rate, duration or intensity of grazing (or any combination of such activities) to promote soil vegetation cover or improve soil health, or both’ and ‘using legume species in cropping or pasture systems’. The aims of this literature critique were: to assess the quality of currently available evidence on the effect of these two management activities on SOC stocks in exclusively grazing systems (i.e. excluding mixed farming systems); to critique how the effects of the management activities were measured; consider the implications of the evidence base for these management activities; and how it may affect those landholders considering embarking on a soil carbon project under current guidelines.
Methodology
Search terms and article selection approach
The search terms ‘rotational grazing’, ‘grazing management’, and ‘legumes’ were used in combination with other search terms to find research articles on the impact of grazing management and legume inclusion in Australian pastoral systems. The terms were selected because they are both eligible management activities under the 2021 Estimating Soil Organic Carbon Sequestration Using Measurement and Models method, and to establish the level of evidence available, especially evidence that was conducted in the field and established SOC stocks according to current method guidelines (Federal Register of Legislation 2021).
The literature critique was undertaken in two stages. The first stage used the Scopus database to search for relevant literature. The first search string query entered into the ‘Search documents’ bar was ‘soil organic carbon’ AND ‘rotational grazing’ OR ‘grazing management’. The second search string query was ‘soil organic carbon’ AND ‘legumes’ AND NOT ‘crop’. The scope of the literature search included no date or country restrictions and was limited to published journal articles. These two searches returned 27 and 30 articles, respectively. The second stage of the review was to undertake a manual review of the 57 articles, and apply inclusion or exclusion criteria to decide on the final set of eligible articles. The inclusion criteria for the review were: the study was conducted in Australia; under a pastoral land use with a comparison of soil carbon stocks to at least 0.3 m; between set stocking and at least one other type of grazing management; or comparing pasture management with and without legumes. The exclusion criteria were: the study was conducted outside Australia; using pot trials (in Australia); soil carbon stocks not calculated; soil sampled at shallow depth (<0.1 m); and in cropping or mixed livestock and cropping systems. The inclusion and exclusion criteria for studies were directly related to their relevance to the eligible management activities, and calculation of SOC stocks in the 2021 Estimating Soil Organic Carbon Sequestration Using Measurement and Models method (Federal Register of Legislation 2021). The method is specific to Australia, and ACCUs can be issued using measured and modelled changes in soil carbon stocks. Eligible management activities must meet the ‘newness requirement’, hence the comparisons between the eligible management activities selected for this review, rather than articles that explore other eligible management activities that appeared in the search strings, for example switching from cropping to pasture or including a legume in a cropping rotation.
Following the manual review, 10 articles on grazing management and four articles on legumes (Table 1), one of which had already appeared in the rotational grazing search query, were selected for closer examination. In addition, if articles were identified outside the search terms, then their eligibility was examined using the stated inclusion/exclusion criteria.
Source | Location | Experimental design and sampling regime, Length of time (years) activity applied | Strength of Experimental design based on Reinhart et al. (2022) | Climate zone | Soil type | Type of grass pastures | Type of activity | Soil carbon accumulation outcome | |
---|---|---|---|---|---|---|---|---|---|
Allen et al. (2013) | 18 grazing properties in Qld | One point in time all sites sampled. No baseline measures of SOC. Some paired sites with grazing regime and exclosure. 10-year paddock history taken. Grazing treatment not stratified according to climate. | Silver | Subtropical, Tropical grasslands and Desert | Chromosol, Hydrosol, Dermosol, Kandosol, Vertosol, Tenosol, Rudosol, Sodosol | Range of grass types | Continuous, Rotational, Cell, Exclosure (absence of grazing) | Cell grazing (34 Mg/ha) was associated with higher SOC stocks than Continuous (18 Mg/ha) grazing and Exclosure (16 Mg/ha), but not Rotational grazing (29 Mg/ha). However, once the location bias for sites was accounted for SOC stocks was slightly lower in Cell grazing than Continuously grazed plots, due to climate differences. | |
Badgery et al. (2014) | 75 locations in Central west of NSW | One point in time all sites sampled. No baseline measures of SOC. Historical management information recorded. Average length of pasture phase 4.7 over 10-year period. | Bronze | Cool temperate and warm temperate | Red and Brown Luvisols and Lixisols (WRB), Red and Brown Chromosols and Dermosols (Isbell 2002) | Native perennial pasture in rotation, permanent pasture, and pasture crop in rotation | Continuous grazing vs intensive rotational grazing | No significant influence of pasture management on total SOC. Generally, there were larger differences in the POC due to land use and management than the other SOC fractions. | |
Chan et al. (2010) | South-eastern Australia (23 sites – Gulgong in the north to south of Albury) | One point in time all sites sampled. No baseline measures of SOC. At least 10 years under the same management. | Silver | Cool temperate and warm temperate | All paired sites had the same soil type (site soil types not specified) | Various annual and perennial pastures, summer and winter active | Continuous vs rotational grazing | No significant change in soil carbon stocks with the grazing management treatment. | |
Conrad et al. (2017) | Gayndah, Qld | Tropical forage tree established in 1969, and at various time periods after. 40, 34, 22, and 9 years established. Space for time substitution. Baseline at time zero, and different aged stands sampled in 2009. | Gold | Subtropical | Vertisols (four of the sites) and Alfisols (one site) | Leucaena plant and dominant grass species were green panic, sabi, blue grass, buffel grass, black spear grass | Subtropical leucaena plant and grass pasture | 0.28 Mg/ha/year. | |
Conrad et al. (2018) | Gayndah, Qld | Tropical forage tree established in 1969, and at various time periods after. 40, 34, 22, and 9 years established. Space for time substitution. Baseline at time zero, and different aged stands sampled in 2009. Paired sites to compare grass pastures to Leucaena plant and grass pastures. | Gold | Subtropical | Vertosol, clay 55–63%, alkaline soil | Leucaena plant and dominant grass species were green panic, sabi, blue grass, buffel grass, black spear grass | Subtropical leucaena plant and grass pasture | Only a comparison between pastures. Leucaena rows displayed significantly higher SOC stock compared to grass pasture. | |
Cowie et al. (2013) | Northern Tablelands, NSW | One point in time all sites sampled. No baseline measures of SOC. Historical management information recorded. At least 5 years of the management practice for Rotational Grazing and 10 years for Continuous grazing. | Bronze | Cool temperate | Chromosol | Not specified | Grazing | Not significant, although SOC stocks were higher in Rotational grazing compared with Continuous grazing. | |
Myers (1976) | Katherine, NT | One point in time sampled. Paired sites. Townsville styo pasture established for 3–15 years and a total of 10 sites. No baseline measures of SOC. Space for time substitution. | Bronze | Tropical | Tindall clay loam, also referred to as a Tippera clay loam | Perennial grass under low open eucalypt forest | Grazed Townsville stylo (Stylosanthes humilis) grass | No differences with grazing management categories. | |
Orgill et al. (2018) | Berridale, NSW | Compared organic carbon and nitrogen stocks at the conclusion of a 5-year grazing trial on a fertilised native pasture in south-eastern Australia. One point in time all sites sampled. No baseline measures of SOC. Comparative. On farms. | Silver | Cool temperate | Lixosol (WRB), Brown Chromosol ASC, 10–20% clay in the top 32 cm (sandy clay loam/sandy loam) | Native grassland dominated by native perennial grass species, including wallaby grasses, spear grasses, and snowgrass. Naturalised annual grasses, legumes, and weeds present | Ungrazed, tactically grazed, cell grazed (sheep). Tactically grazed plots were rested for 4–6 weeks over late spring/summer and late summer/autumn to allow seed set and recruitment. Cell grazed for 1 day every 80–120 days at 300–400 DSE/ha. Several destocking events occurred | Assuming the plots had similar initial SOC stocks then a difference of 1.46 Mg C/ha/year under cell grazed treatment and 0.78 Mg C/ha/year in tactically grazed treatments compared to no grazing. | |
Pringle et al. (2011) | Wambiana Station, southwest of Charters Towers, Qld | One point in time all sites sampled. No baseline measures of SOC. Comparative. Two paddocks: one with a historically moderate cattle-stocking rate, and the other with a historically heavy cattle-stocking rate for 12 years. | Bronze | Subtropical | Dominant soil map unit is a soil complex, comprising brown Sodosol-yellow Kandosol, grey Vertosol, red Kandosol | C4 tropical grasses | Grazing management. Two treatments with the greatest contrast in grazing pressure were selected. These were the ‘heavy’ and ‘moderate’ stocking-rate | Significant effect of soil type on soil carbon but no effect of grazing pressure. | |
Pringle et al. (2014) | Toorak, Qld | The experiment was established in 1984, consisting of five unreplicated nominal rates of pasture utilisation by sheep (10%, 20%, 30%, 50%, and 80%) and an exclosure (ungrazed by sheep, 0%). At time of sampling, it was 26 years. One point in time SOC measured, and no baseline measures. 10 samples per treatment. | Bronze | Tropical grassland | Vertosol; clay content 55–60% | Tropical Mitchell grass (Astrebla spp.) | Pasture utlilization rate | No significant effect of pasture utilisation rate on SOC stocks. Ultimately the sampling scheme lacked the statistical power to adequately elucidate the full range of effects that we expected to observe. | |
Radrizzani et al. (2011) | Gayndah, Qld; Banana, Qld | Leucaena forage tree and grass pastures that had been established for 20, 31, and 38 years. Paired transects. Two sampling point in 2006 and 2007. Baseline of SOC as on established Research Station for part of study. | Bronze | Subtropical | Vertosol | Leucaena plant and grass pastures compared with native grass pastures | Leucaena forage plant | For leucaena-grass pastures that had been established for 20, 31, and 38 years, OC accumulated at rates that exceeded those of the adjacent native grass pasture by 267, 140, and 79 kg/ha.year, respectively. | |
Sanderman et al. (2015) | South Australia | One point in time soil sampling in 2013 when not being actively grazed with landowners identified as having adopted rotational grazing practices over the past 5–15 years. 12 paired sites that were rotationally grazed and continuously grazed and on same soil type and landscape position. No baseline measures of SOC. Space for time substitution. | Silver | Desert, Grassland and warm temperate | Rocky, shallower soils. Red Chromosols and some sites there were some Calcarosols | Native perennial grasses (spear grass, wallaby grass) annual grasses (e.g. wild oats, silver grass, brome grass) | Rotational grazing | Mean SOC stocks (0–30 cm) were 48.3 Mg C ha−1 with a range of 20–80 Mg C ha−1 across the study area with no differences between grazing management categories. SOC stocks were well correlated with rainfall and temperature (multiple linear regression R2 = 0.61). After removing the influence of climate on SOC stocks, the management variables, rest periods, stocking rate, and grazing days were found to be significantly correlated with SOC, explaining 22% of the variance in SOC, but there were still no clear differences in SOC stocks at paired sites. | |
Schatz et al. (2020) | Douglas Daly, NT | Nine-year study, with SOC measured twice each year for 5 years (2009–14). Repeated soil sampling on research station with two grazing management types. Baseline measure of SOC in 2009. | Silver | Tropical | Blain soil type ‘sandy red earth’ | Buffel grass (79%), some other annual grasses and weeds | Continuous grazing vs IRG (intensive rotational grazing) | No differences with grazing management categories. 45% of SOC stocks was located in top 10 cm. | |
Waters et al. (2017) | Semi-arid rangelands of southern Australia | Three locations and paired comparison with 5 rotational grazing sites, 1 Continuous grazing site, and 5 no stock but native animal grazing present. 20 random sampling points in each site at one point in time. No baseline measure of SOC. 7 years of stocking rate history from landholders gathered. | Bronze | Grassland | L1 nd L2 were Lixisols (WRB). L1 flood plain Vertisols | Variable | Rotational grazing – managers aimed to rotate livestock from one paddock to the next with a goal of achieving approx. 30% pasture utlisation rate in each rotation | Grazing management had no effect on SOC or TN on grey soils (Vertisols); but for red soils (Lixisols), significantly higher levels of SOC were found for both the 0–5- and 5–10-cm soil depths (0.3% and 0.27%, respectively) and associated with increased TN. |
Analysis
The 14 articles that met the inclusion criteria were examined to extract data on the following parameters. The information from each article included: SOC accumulation (tonnes of carbon per hectare per year); soil sampling depth (m); measured or modelled; experimental design and sampling regime; length of time over which the study was conducted (years) and eligible activity applied; type of management activity (grazing management or legumes); type of grazing or type of legume; location, climate, and average annual rainfall (AAR); and soil type. In addition, for each article the strength of evidence was assessed based on Reinhart et al. (2022) who developed three standards: before–after randomised controlled trial (Gold standard); randomised controlled trial (Silver standard); and control-impact trial (Bronze standard). Some articles conformed to higher levels, but also may have lacked other qualities, such as calculation of SOC stocks based on equivalent soil mass, which placed them in the lower category.
Results and discussion
Distribution of the literature
The legume research was based in Queensland and the Northern Territory, which both have a strongly summer-dominant rainfall pattern, with study sites’ AAR ranging from 667 to 900 mm and all soils clay or clay loam. Additionally, three out of four articles on legumes were using a subtropical leucaena plant and grass pasture, with only one article on a different type of legume plant. The grazing management articles were based in Victoria, South Australia, Queensland, New South Wales, and the Northern Territory. No published research on either management activity was recorded for Tasmania or Western Australia. The lack of research in Tasmania is particularly significant because it has mostly high rainfall and colder temperatures. Fig. 1 shows approximate study locations of the articles included for analysis, as some studies did not give exact locations, and covered an area of hundreds of kilometres. For studies with multiple locations, the furthermost east, west, north, and south locations were approximated. In terms of soil types, a limitation of comparing the studies is that there were inconsistencies between the soil classification systems. The most common soil classification was the Australia Soil Classification system (Isbell 2002), and the next most common was the World Reference Base soil classification system. The Northcote Factual Key was used, and for the two sites in the Northern Territory where the soils were classified based on historical descriptions from other research papers conducted by the territory government. Most soils were classified to the soil order level and rarely comprehensively described to the soil type level (Table 1). It therefore would be more consistent to focus on soil texture type. Of note, all research sites with lighter textured soil experience either no change or no significant change in SOC stocks, whereas some (but not all) of the sites with heavier textured or more clay-dominant soils, such as Vertosol, recorded increases in SOC stocks with management changes (Radrizzani et al. 2011; Conrad et al. 2017, 2018) (Table 1). From the information available, it appears that most major Australian soil types were covered in the research.
All of the eligible articles were published between 2010 and 2020, except for one outlier that was published in 1976. The Kyoto Protocol was adopted on 11 December 1997. It operationalises the United Nations Framework Convention on Climate Change, and since its release has increased public awareness of the significance of carbon dioxide sinks as a form of carbon offset. Soils, and agricultural soils in particular, form a significant portion of the opportunity to achieve emissions reduction targets. Australia is one of the 192 parties to the Kyoto Protocol (United Nations Climate Change 2022). Adoption of the Protocol has likely contributed to increased research in recent times on the capacity of agricultural soils in Australia to sequester carbon. The Clean Energy Act took effect on 1 July 2012, which likely triggered some of the more recent research. Just over a third of the published research (36%) was part of Australia’s National Soil Carbon Research Programme (SCaRP) (2009–2012), and National Soil Carbon Programme (NSCP) (2012–2015), for each state and territory in Australia, while another third was funded by Meat Livestock Australia in collaboration with state government agencies, and the remainder were funded from bespoke programmes or funding was not disclosed.
Experimental sites and duration of study
Each climate zone (Fig. 1) was represented on at least one experimental site of the dataset studied, with some articles having multiple experimental sites across up to three different climate zones (Fig. 2).
Climate zones (according to Koppen Climate classes) in which the article’s study site(s) is/are located. Coloured cells indicate presence in a climate zone and white cells indicate there is no study in that climate zone (source: Table 1).
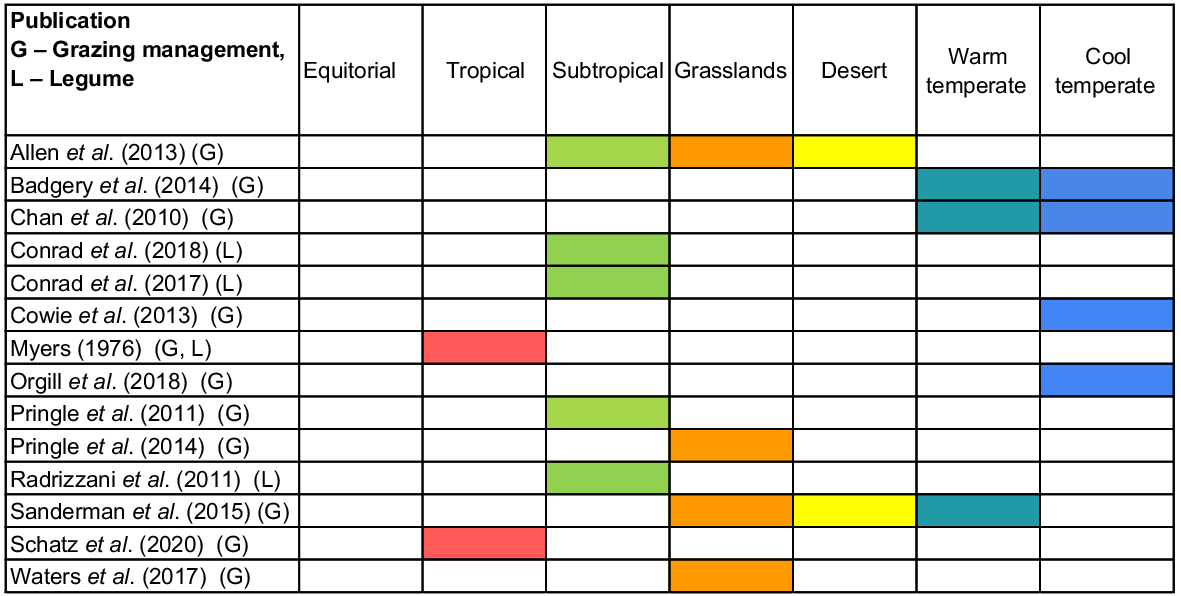
The majority of the articles (72%) were replicated paired sites, but with no baseline measure of SOC stocks. Therefore, SOC stocks were a relative comparison between treatments at one point in time, and were not a reflection of changes in SOC stocks before imposition of management activity. These comparative observational studies did not have a baseline measurement or a subsequent measurement of SOC at a second point in time. This presents significant limitations to showing whether the management activities result in accumulation of soil carbon stocks because it assumes each sampling area had a similar starting point (Table 1).
For the remaining 28% of studies, which were also replicated, there was a baseline of SOC stocks, with at least one subsequent set of measurements. The length of time the studies were active for was highly variable, ranging from the shortest, at 5 years, and the longest, at 40 years (Table 1). All these studies were on a research station where conditions can be monitored consistently, but still consisted of one sampling period as using a space for time substitution experimental design. The length of study is important due to the other factors that influence SOC stocks, particularly drought, so that climate variability and atypical years can be accounted for in a longer period of study.
The majority of study sites were situated on commercial farms (72% of articles), while the remaining study sites were situated on research stations. Most of the study sites that were on research stations were those that had measurements over at least two points in time to allow for temporal comparison. There are pros and cons of commercial farms over a research station that relate to greater research relevance and credibility with on-farm research. Overall, the proportion of studies that were classified as Gold standard in terms of experimental design and explanatory power were low (14%), followed by 36% of studies at Silver standard, and 50% of studies at Bronze standard (Table 1). Reinhart et al. (2022) in their global review on grazing management and SOC stocks reported a lower proportion of studies at Gold Standard (4%), and 68% of studies at Silver standard, and the remainder of studies at Bronze standard. Cowie et al. (2013) explained some of the experimental problems that arise from comparative studies, including the limitations of the survey approach that was used as it had limited capacity to detect differences in SOC stocks that were attributable to specific management activities because of the wide variation in management histories and strong influence of environmental factors.
Soil measurement depth
Depths to which soil measurements were taken were from 0.15 to 1 m (Fig. 3), typically divided into smaller intervals closer to the surface soil, which were 0–0.1 m, 0.1–0.2 m and 0.2–0.3 m. Approximately half of the papers measure SOC stocks to 0.3 m (n = 7). There was a lack of measurement of SOC at depths greater than 0.3 m (n = 5), despite anecdotal evidence that influencing SOC at depth presents an opportunity for increased ACCUs, which can be beyond 0.3–0.5 m into the subsoil. However, soil carbon sequestration beyond 0.3 m is not reported to the United Nations as part of the national GHG inventory, even though soil carbon sequestration could be occurring at deeper depths (Simmons et al. 2023). In addition, only 50% of the studies examined SOC stocks between the grazing treatments or baseline measurement using an equivalent soil mass (Fig. 3).
Maximum sampling depth of soil cores (n = 14 articles), G - Grazing management, L - Legume, ESM - Equivalent Soil Mass (source: Table 1).
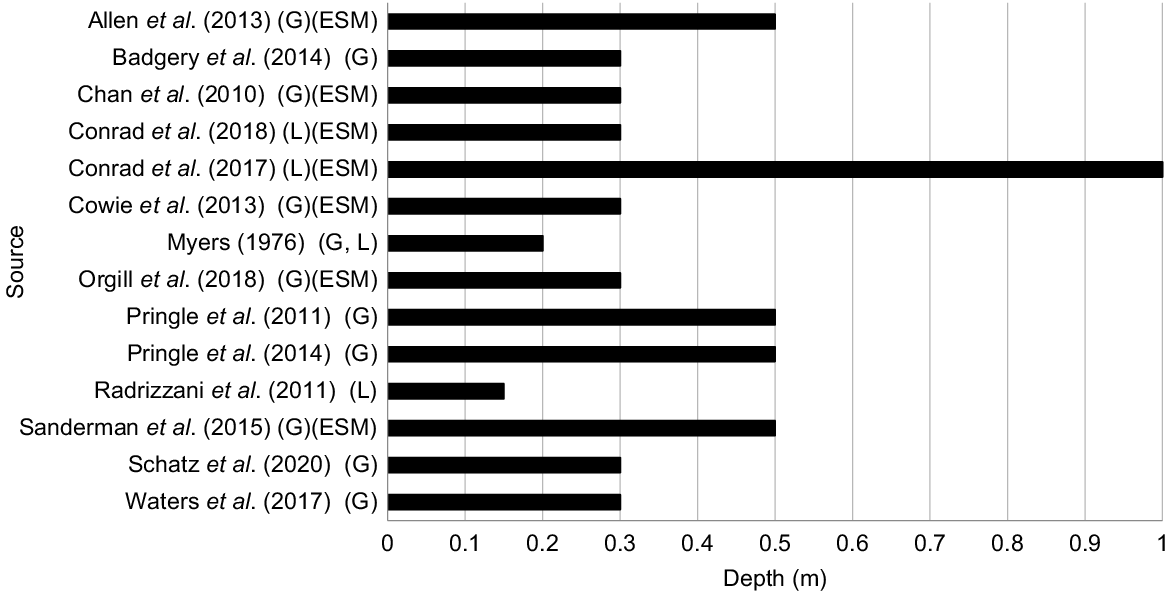
Effect of grazing management and legumes on SOC stock
The articles included in this review were from a peak period of publicly funded research and were published from 2010 to 2020. Since the end of the project funding, there has not been any follow-up research on those same experimental study sites. The scale of the research often included other explanatory variables of soil carbon sequestration, such as soil type, climate, and land use type, which confounded the effect of the management practice (Amin et al. 2020). Most articles that appeared in both search strings were ultimately excluded, due to not conforming to all inclusion criteria, and others examining the conversion of crop to pasture, which reflects a potential research bias. Another recent review on grazing management for soil carbon in Australia identified 30 published articles, but 17 of these articles were excluded from this literature critique (McDonald et al. 2023). There were 12 articles common to both reviews.
The variation in experimental design, and in the measurement of SOC stocks under the management activities, made it difficult to compare findings between studies. The variation in how SOC stocks were reported also made it difficult to compare the various studies. The reported impact of the grazing treatments were tabulated and were generally not significant (Table 1). One grazing management study suggested that 1.46 Mg C ha−1 year−1 to a depth of 0.3 m over 5 years could accrue, but this was also based on an assumption that SOC levels were similar at the start for all treatments (Orgill et al. 2018) (Table 1). Less than a third of the articles (28%) found that SOC stocks were positively influenced by either grazing management (n = 1) or legume inclusion (n = 3); and for the legume studies, there was baseline measurement of SOC stocks and the work was conducted on a research station under long-term trials (Table 1). The lack of significance in findings derived from observational studies means a level of caution needs to be applied to eligible activities for soil carbon projects, when the only significant change is recorded on a research station. Often the geographical spread of sites on commercial farms studied led to a highly variable dataset where only a relative comparison is useful. Dean et al. (2012) noted that substituting space for time can be inferior to long-term experimental sites, since the former approach needs to take into account spatial heterogeneity, appropriate site-pairing, and interconnections for SOC in time.
Additionally, apart from one article, the results were not also presented in SOC%, which would aid in communication of the research findings to landowners, who are more likely to measure SOC in percentage than stocks (Ingram et al. 2016). The separate sources of funding for the identified studies meant they have operated largely independently of each other, without coordination of influential factors, climate, soil, land use practice, or grass type. In addition, competitive funding has restricted the type of studies that have taken place and the length of study and ability to undertake long-term work, which measures temporal effects over time (Lobry de Bruyn et al. 2022). Soil bulk density used to measure SOC stocks would also change over time with grazing treatment, and hence sampling depth would need to be adjusted or soil mass kept constant between treatments for equivalent soil mass calculations, which only occurred in 50% of studies. In a global review of grazing and its effects of SOC, most studies (n = 71) had reported SOC stocks for a specific depth interval (0–30 cm), and grazing is known to alter bulk density (Reinhart et al. 2022). Also, this meta-analysis reported that only three of the 71 studies (<4%) had tested effects of grazing treatments on changes in SOC variables (Reinhart et al. 2022). Furthermore, the ambiguity of the impact of eligible activities on SOC stocks means that for carbon project developers it could result in a lack of legitimacy or uncertainty in soil carbon project deliverables. For example, when determining the profitability of a soil carbon project by predicting sequestration rate over a project crediting period (25 years for soil carbon projects registered under the ACCU Scheme), a project developer may observe a dataset that indicates carbon sequestration at a rate of 0.5 t CO2e ha−1 year−1 but it is difficult to interpret in that dataset how long that sequestration rate could be maintained for, and the developer cannot assume that this sequestration rate is possible for the entirety of the crediting period.
Interaction between the two management activities
The interaction between the inclusion of legumes and different grazing management activities and their combined impact on SOC stocks was not addressed in any of the articles. The best potential for SOC sequestration is likely a combination of these management activities, rather than one or the other. However, this is only relevant to a point, that is soils can only sequester up to as much carbon as they can hold, which is dependent on inherent soil characteristics such as clay percentage, referred to as the ‘soil carbon saturation concept’ (Hassink 1997; Six et al. 2002). Orgill et al. (2018) was the only article in which the saturation capacity was mentioned at the end of the discussion. A recent review questioned the level of carbon saturation, and that soils under pasture are probably already close to the ceiling of SOC saturation with little potential for more soil carbon sequestration (Moinet et al. 2023). This presents a limitation of the data available, because if some of the soils were close to their saturation point while others were not, it is less likely a meaningful comparison can be made.
Exploration of other facets of SOC accumulation
Many of the articles were also investigating total nitrogen and presenting the different pools of carbon (particulate, humic, and recalcitrant pools) under different scenarios. Co-benefits such as increased livestock carrying capacity, above and below ground biomass, plant available water (except for Radrizzani et al. 2011), drought resilience, and on-farm profitability due to increased soil carbon stocks were not explored.
Legume inclusion in pastoral systems has been found to have several co-benefits due to their biological nitrogen fixation capacities, as well as additional ecological services such as improved soil structure, erosion protection, and increased biodiversity. However, there has been a decline in the inclusion of leguminous plants in farming systems with readily available synthetic nitrogen fertiliser (Jensen and Hauggaard-Nielsen 2003). Legume-induced soil acidification has been observed (Haynes 1983), and a long-term fertility trial in the USA found that ammoniacal nitrogen fertiliser was accompanied by increased soil acidity, and decreased cation exchange capacity, exchangeable calcium, and magnesium ions (Barak et al. 1997). It has also been argued that legume-derived nitrogen has similarly negative environmental impacts (Crews and Peoples 2004). Further research is required to establish the net GHG emissions of legume inclusion versus the use of synthetic nitrogen fertiliser, as well as the other environmental impacts of each of these practices. It could be argued that sourcing nitrogen for plant growth from either legumes or fertiliser is practical for Australian pastoral systems, noting that in some systems, particularly intensively farmed dairies, legume establishment and persistence is rare.
There are different ways to manage grazing in farming systems, and there is a broad spectrum of systems and practices that can be described by the term ‘rotational grazing’ with more common terms including deferred grazing, rotational grazing (e.g. intensive, simple, and slow), time-controlled grazing, cell grazing, and set stocking. In a recent survey of Australian farmers, 62% of those surveyed were undertaking a form of rotational grazing, and most for more than 3 years (Coelli 2021). Under grazing management, finding the optimal grazing pressure can also be included. Nie et al. (2015) found that deferred grazing treatments resulted in a 200% perennial grass germinable seed pool increase and a 50% germinable seed pool increase for annual grasses. Sanjari et al. (2008) found that time-controlled grazing compared with continuous grazing reduced nitrate and extractable phosphorus concentrates due to increased grass growth under the time-controlled grazed areas, which decreased contamination potential for downstream water bodies. One of the limitations of comparing the identified articles is that different grazing management activities were imposed in the examined studies or it was an observational study collecting data from operating commercial farms, and therefore comparison is difficult as it is not a fully factorial, replicated, and controlled experimental design with baseline measures of SOC stocks (Table 1).
Grazing management and legume inclusion in Australian pastoral systems are management activities that can increase farm profitability, provided they are implemented strategically. The bio-economic analysis of grazing regimes has been studied in the south-west of Western Australia and it was demonstrated that rotational grazing and continuous grazing were both profitable but the more robust regime was rotational grazing (Sounness 2004). In both systems, perennial pastures were also superior to annual pastures. For example, the capital and labour costs of a rotational or cell grazing system must be considered prior to implementation of such a strategy, as this system requires relatively small paddock size and frequent movement of stock. Legume inclusion is likely to result in decreased fertiliser use; however, establishing legumes in some systems may be challenging and seeding multiple times may be required. Another significant challenge to legume establishment and persistence, particularly lucerne (Dear et al. 2003), is the prevalence of acid soils in Australia. This is especially prevalent in the more intensively grazed systems, of which it is thought approximately 21% have a high risk of acidification. Acid soils increase the risk of topsoil loss through erosion because they have decreased ability to support productive vegetation cover, thereby reducing potential inputs to SOC stocks (Barson et al. 2011).
Additionally, there is a social dimension to adoption of new management activities. Examination of long-term practitioners of rotational grazing in the New England Tablelands of New South Wales, Australia, showed that soil carbon management was focused more on the co-benefits of managing soil carbon for plant production, soil health, and overall farm sustainability than the opportunity to earn ACCUs (Amin et al. 2023a). While the two management activities assessed in this paper are already at reasonable levels of adoption Australia-wide, especially grazing management, ‘carbon farming’ is reported at 12% of Australian farmers in a recent survey of farm practices (Coelli 2021), with the adoption of a carbon soil project closer to 0.4% of Australian farmers (Fitch et al. 2022). This low level of adoption in a soil carbon project further highlights the need for research on the co-benefits, including on-farm profitability in a commercial farming system and soil stewardship if adoption is to scale-up (Amin et al. 2023b). Pannell and Crawford (2022) argue that paying farmers for carbon that they were going to sequester anyway, despite the policy measures around additionality that intend to prevent this from happening, makes no ‘new’ contribution to mitigating climate change.
Conclusions and recommendations
Anecdotal evidence of increases in SOC through management are commonplace in the soil carbon industry. However, scientific evidence on SOC sequestration potential associated with a change in grazing management or legumes in pastures, particularly at depth, is relatively scarce in Australia. This critique identified a number of limitations in the evidence base on the effect of the two management activities on SOC stocks. Published studies were mainly in New South Wales and Queensland (from 2010 to 2020) on a variety of soil types across all climatic zones funded under several time-bound research programmes. Publication of research from that period emanating from Tasmania and Victoria is also required where the climate and soil types have a greater potential for SOC sequestration. The explanatory power of environmental and grazing management factors in relation to SOC stocks may be strengthened by the scale of the research that has a common climate, rainfall, and soil type setting, and can consider the influence of soil management exclusively on SOC stocks (Amin et al. 2020). This was the case with the studies (n = 3) that did show accumulation of SOC that had sampled SOC stocks before and after treatment imposed, accounted for equivalent soil mass, and were all conducted on a single research station. Interpreting the impacts of management were also hindered by insufficient documentation of grazing management history that was often confounded by the biophysical drivers of soil carbon dynamics, and reporting SOC stocks for a fixed soil depth (50% of studies). It was suggested by Allen et al. (2013) that closer quantification of grazing management based on stocking density would improve data interpretation. An investment in resampling those sites sampled more than 10 years ago in close proximity to original sampling location could provide greater insight into the expected levels of SOC accumulation, and the support of long-term experimental trials as suggested by other researchers in their original work (Chan et al. 2010; Allen et al. 2013). Also, no reported studies examined the interaction between the two eligible management activities, which highlights the need for research using a whole systems approach, including the positive and negative influences on SOC stocks and how much each part of the system contributes. To study the impact of the two management activities on SOC stocks, increasing on-farm experimentation may be of value to provide increased coverage and place-based research. Considerations of scale, context, and the capacity of farmers to implement these management activities using best practice such as resources, knowledge, and skills could also be examined in future research if placed on commercial farms.
Acknowledgements
Tani Cooper, Senior Spatial Sciences Co-ordinator at the University of New England for the construction of Fig. 1.
References
Allen DE, Pringle MJ, Bray S, Hall TJ, O’Reagain PO, Phelps D, Cobon DH, Bloesch PM, Dalal RC (2013) What determines soil organic carbon stocks in the grazing lands of north-eastern Australia? Soil Research 51(8), 695-706.
| Crossref | Google Scholar |
Amin MN, Hossain MS, Lobry de Bruyn L, Wilson B (2020) A systematic review of soil carbon management in Australia and the need for a social-ecological systems framework. Science of The Total Environment 719, 135182.
| Crossref | Google Scholar | PubMed |
Amin MN, Lobry de Bruyn L, Hossain MS, Lawson A, Wilson B (2023a) The social-ecological system of farmers’ current soil carbon management in Australian grazing lands. Environmental Management 72, 294-308.
| Crossref | Google Scholar |
Amin MN, Lobry de Bruyn L, Lawson A, Wilson B, Hossain MS (2023b) Lessons learned from farmers’ experience of soil carbon management practices in grazing regimes of Australia. Agronomy for Sustainable Development 43(1), 5.
| Crossref | Google Scholar |
Australian Bureau of Statistics (2021) Agricultural commodities, Australia. (ABS, Canberra) Available at https://www.abs.gov.au/statistics/industry/agriculture/agricultural-commodities-australia/latest-release#:~:text=At%2030%20June%202020%20there%20were%3A%20377%20million,were%20used%20for%20grazing%2C%20down%202%25%20from%202018-19
Badgery WB, Simmons AT, Murphy BW, Rawson A, Andersson KO, Lonergan VE (2014) The influence of land use and management on soil carbon levels for crop-pasture systems in Central New South Wales, Australia. Agriculture, Ecosystems & Environment 196, 147-157.
| Crossref | Google Scholar |
Barak P, Jobe BO, Krueger AR, Peterson LA, Laird DA (1997) Effects of long-term soil acidification due to nitrogen fertilizer inputs in Wisconsin. Plant and Soil 197(1), 61-69.
| Crossref | Google Scholar |
Barson M, Mewett J, Paplinkska J (2011) Land management practice trends in Australia’s grazing (beef cattle/sheep) industries. (ABARES: Canberra) Available at https://www.abs.gov.au/ausstats/abs@.nsf/mf/4630.0
Bureau of Meteorology (2023) Climate classification maps, Koppen – major classes. Bureau of Meteorology, Australian Government. Available at http://www.bom.gov.au/climate/maps/averages/climate-classification/?maptype=kpngrp [Accessed 9 January 2024]
Chan KY, Oates A, Li GD, Conyers MK, Prangnell RJ, Poile G, Liu DL, Barchia IM (2010) Soil carbon stocks under different pastures and pasture management in the higher rainfall areas of south-eastern Australia. Soil Research 48(1), 7-15.
| Crossref | Google Scholar |
Coelli R (2021) Natural resource management and drought resilience – a survey of farm practices. (ABARES: Canberra, ACT) Available at https://doi.org/10.25814/99n0-7q92
Conrad KA, Dalal RC, Dalzell SA, Allen DE, Menzies NW (2017) The sequestration and turnover of soil organic carbon in subtropical leucaena-grass pastures. Agriculture, Ecosystems & Environment 248, 38-47.
| Crossref | Google Scholar |
Conrad K, Dalal RC, Fujinuma R, Menzies NW (2018) Soil organic carbon and nitrogen sequestration and turnover in aggregates under subtropical leucaena–grass pastures. Soil Research 56(6), 632-647.
| Crossref | Google Scholar |
Cowie AL, Lonergan VE, Rabbi SMF, Fornasier F, Macdonald C, Harden S, Kawasaki A, Singh BK (2013) Impact of carbon farming practices on soil carbon in northern New South Wales. Soil Research 51(8), 707-718.
| Crossref | Google Scholar |
Crews TE, Peoples MB (2004) Legume versus fertilizer sources of nitrogen: ecological tradeoffs and human needs. Agriculture, Ecosystems & Environment 102(3), 279-297.
| Crossref | Google Scholar |
Dean C, Roxburgh SH, Harper RJ, Eldridge DJ, Watson IW, Wardell-Johnson GW (2012) Accounting for space and time in soil carbon dynamics in timbered rangelands. Ecological Engineering 38(1), 51-64.
| Crossref | Google Scholar |
Dear BS, Moore GA, Hughes SJ (2003) Adaptation and potential contribution of temperate perennial legumes to the southern Australian wheatbelt: a review. Australian Journal of Experimental Agriculture 43(1), 1-18.
| Crossref | Google Scholar |
Federal Register of Legislation (2012) Clean energy legislation amendment Act 2012. Available at https://www.legislation.gov.au/C2012A00084/latest/text
Federal Register of Legislation (2021) Carbon credits (carbon farming initiative—estimation of soil organic carbon sequestration using measurement and models) methodology determination 2021. Available at https://www.legislation.gov.au/Details/F2021L01696
Fitch P, Battaglia M, Lenton A, Feron P, Gao L, Mei Y, Hortle A, Macdonald L, Pearce M, Occhipinti S, Roxburgh S, Steven A (2022) Australia’s sequestration potential. (CSIRO) Available at https://www.csiro.au/-/media/Missions/TNZ/CCA-report/CCA-Report-Australias-Potential-Sequestration-Final-28-November-2022.pdf
Hassink J (1997) The capacity of soils to preserve organic C and N by their association with clay and silt particles. Plant and Soil 191(1), 77-87.
| Crossref | Google Scholar |
Haynes RJ (1983) Soil acidification induced by leguminous crops. Grass and Forage Science 38(1), 1-11.
| Crossref | Google Scholar |
Ingram J, Mills J, Dibari C, Ferrise R, Ghaley BB, Hansen JG, Iglesias A, Karaczun Z, McVittie A, Merante P, Molnar A, Sánchez B (2016) Communicating soil carbon science to farmers: Incorporating credibility, salience and legitimacy. Journal of Rural Studies 48, 115-128.
| Crossref | Google Scholar |
IPPC (2021) IPCC sixth assessment report: climate change 2021. (IPPC: Cambridge) Available at https://www.ipcc.ch/assessment-report/ar6/
Jensen ES, Hauggaard-Nielsen H (2003) How can increased use of biological N2 fixation in agriculture benefit the environment? Plant and Soil 252(1), 177-186.
| Crossref | Google Scholar |
Kopittke PM, Berhe AA, Carrillo Y, Cavagnaro TR, Chen D, Chen Q-L, Román Dobarco M, Dijkstra FA, Field DJ, Grundy MJ, He J-Z, Hoyle FC, Kögel-Knabner I, Lam SK, Marschner P, Martinez C, McBratney AB, McDonald-Madden E, Menzies NW, Mosley LM, Mueller CW, Murphy DV, Nielsen UN, O’Donnell AG, Pendall E, Pett-Ridge J, Rumpel C, Young IM, Minasny B (2022) Ensuring planetary survival: the centrality of organic carbon in balancing the multifunctional nature of soils. Critical Reviews in Environmental Science and Technology 52(23), 4308-4324.
| Crossref | Google Scholar |
Kopittke PM, Minasny B, Pendall E, Rumpel C, McKenna BA (2023) Healthy soil for healthy humans and a healthy planet. Critical Reviews in Environmental Science and Technology 54, 210-221.
| Crossref | Google Scholar |
Lal R, Negassa W, Lorenz K (2015) Carbon sequestration in soil. Current Opinion in Environmental Sustainability 15, 79-86.
| Crossref | Google Scholar |
Lal R, Monger C, Nave L, Smith P (2021a) The role of soil in regulation of climate. Philosophical Transactions of the Royal Society B: Biological Sciences 376(1834), 20210084.
| Crossref | Google Scholar |
Lal R, Bouma J, Brevik E, Dawson L, Field DJ, Glaser B, Hatano R, Hartemink AE, Kosaki T, Lascelles B, Monger C, Muggler C, Ndzana GM, Norra S, Pan X, Paradelo R, Reyes-Sánchez LB, Sandén T, Singh BR, Spiegel H, Yanai J, Zhang J (2021b) Soils and sustainable development goals of the United Nations: an International Union of Soil Sciences perspective. Geoderma Regional 25, e00398.
| Crossref | Google Scholar |
Lobry de Bruyn LA, North S, Biggs A, Oliver IC, Wong VNL, Knox OGG (2022) Australian priorities for soil research and land manager engagement to improve sustainable soil management. Geoderma Regional 29, e00509.
| Crossref | Google Scholar |
Macintosh A, Waugh L (2012) An introduction to the carbon farming initiative: key principles and concepts. Environmental and Planning Law Journal 29, 439-461.
| Google Scholar |
McDonald SE, Badgery W, Clarendon S, Orgill S, Sinclair K, Meyer R, Butchart DB, Eckard R, Rowlings D, Grace P, Doran-Browne N, Harden S, MacDonald A, Wellington M, Pachas ANA, Eisner R, Amidy M, Harrison MT (2023) Grazing management for soil carbon in Australia: a review. Journal of Environmental Management 347, 119146.
| Crossref | Google Scholar |
Minasny B, McBratney AB, Arrouays D, Chabbi A, Field DJ, Kopittke PM, Morgan CLS, Padarian J, Rumpel C (2023) Soil carbon sequestration: much more than a climate solution. Environmental Science & Technology 57(48), 19094-19098.
| Crossref | Google Scholar | PubMed |
Moinet GYK, Hijbeek R, van Vuuren DP, Giller KE (2023) Carbon for soils, not soils for carbon. Global Change Biology 29(9), 2384-2398.
| Crossref | Google Scholar | PubMed |
Myers RJK (1976) Nitrogen accretion and other soil changes in Tindall clay loam under Townsville stylo/grass pastures. Australian Journal of Experimental Agriculture 16(78), 94-98.
| Crossref | Google Scholar |
Nie ZN, Zollinger RP, Behrendt R (2015) Impact of deferred grazing and fertilizer on herbage production, soil seed reserve and nutritive value of native pastures in steep hill country of southern Australia. Grass and Forage Science 70(3), 394-405.
| Crossref | Google Scholar |
Orgill SE, Condon JR, Conyers MK, Morris SG, Alcock DJ, Murphy BW, Greene RSB (2018) Removing grazing pressure from a native pasture decreases soil organic carbon in southern New South Wales, Australia. Land Degradation & Development 29(2), 274-283.
| Crossref | Google Scholar |
Pannell D, Crawford M (2022) Challenges in making soil-carbon sequestration a worthwhile policy. Farm Policy Journal FPJ1901, Available at https://www.farminstitute.org.au/product/fpj1901-pannell-d-crawford-m-2022-challenges-in-making-soil-carbon-sequestration-a-worthwhile-policy/.
| Google Scholar |
Petersen EH, Hoyle FC (2016) Estimating the economic value of soil organic carbon for grains cropping systems in Western Australia. Soil Research 54(4), 383-396.
| Crossref | Google Scholar |
Pringle MJ, Allen DE, Dalal RC, Payne JE, Mayer DG, O’Reagain P, Marchant BP (2011) Soil carbon stock in the tropical rangelands of Australia: effects of soil type and grazing pressure, and determination of sampling requirement. Geoderma 167-168, 261-273.
| Crossref | Google Scholar |
Pringle MJ, Allen DE, Phelps DG, Bray SG, Orton TG, Dalal RC (2014) The effect of pasture utilization rate on stocks of soil organic carbon and total nitrogen in a semi-arid tropical grassland. Agriculture, Ecosystems & Environment 195, 83-90.
| Crossref | Google Scholar |
Radrizzani A, Shelton HM, Dalzell SA, Kirchhof G (2011) Soil organic carbon and total nitrogen under Leucaena leucocephala pastures in Queensland. Crop and Pasture Science 62(4), 337-345.
| Crossref | Google Scholar |
Reinhart KO, Sanni Worogo HS, Rinella MJ (2022) Ruminating on the science of carbon ranching. Journal of Applied Ecology 59(3), 642-648.
| Crossref | Google Scholar |
Sanderman J, Reseigh J, Wurst M, Young M-A, Austin J (2015) Impacts of rotational grazing on soil carbon in native grass-based pastures in Southern Australia. PLoS ONE 10(8), e0136157.
| Crossref | Google Scholar | PubMed |
Sanjari G, Ghadiri H, Ciesiolka CAA, Yu B (2008) Comparing the effects of continuous and time-controlled grazing systems on soil characteristics in Southeast Queensland. Soil Research 46(4), 348-358.
| Crossref | Google Scholar |
Schatz T, Ffoulkes D, Shotton P, Hearnden M (2020) Effect of high-intensity rotational grazing on the growth of cattle grazing buffel pasture in the Northern Territory and on soil carbon sequestration. Animal Production Science 60(15), 1814-1821.
| Crossref | Google Scholar |
Simmons A, Cowie A, Henry BK, Wilson B, Pannell D, Rowlings D, Mitchell E, Harrison M, Grace P, Viscarra Rossel R, Eckard R, Badgery W (2023) Here’s how to fix Australia’s approach to soil carbon credits so they really count towards our climate goals. Writers in Conversation. The Conversation Media Publishing Ltd, The University of Melbourne.
Six J, Conant RT, Paul EA, Paustian K (2002) Stabilization mechanisms of soil organic matter: implications for C-saturation of soils. Plant and Soil 241(2), 155-176.
| Crossref | Google Scholar |
United Nations Climate Change (2022) What is the Kyoto Protocol? Available at https://unfccc.int/kyoto_protocol
Verschuuren J (2017) Towards a regulatory design for reducing emissions from agriculture: lessons from Australia’s carbon farming initiative. Climate Law 7(1), 1-51.
| Crossref | Google Scholar |
Waters CM, Orgill SE, Melville GJ, Toole ID, Smith WJ (2017) Management of grazing intensity in the semi-arid rangelands of Southern Australia: effects on soil and biodiversity. Land Degradation & Development 28(4), 1363-1375.
| Crossref | Google Scholar |
Young RR, Wilson B, Harden S, Bernardi A (2009) Accumulation of soil carbon under zero tillage cropping and perennial vegetation on the Liverpool Plains, eastern Australia. Soil Research 47(3), 273-285.
| Crossref | Google Scholar |