Ox-eye daisy (Leucanthemum vulgare Lam.) invasion has only a small effect on the diversity of a subalpine grassland
Keith L. McDougall
A
B Independent Consultant.
C Present address:
Abstract
Ox-eye daisy (OED; Leucanthemum vulgare) has invaded subalpine communities in Australia.
We evaluated the effect of OED on subalpine grassland diversity in Kosciuszko National Park through addition and removal experiments.
In 2023 and 2024, we conducted two experiments on grassland that had been partly invaded by OED: (1) addition of OED seed to uninvaded plots, hypothesised to reduce α– and β-diversity; and (2) removal of OED plants by herbicide treatment and hand-pulling, hypothesised to temporarily reduce diversity. After 3 years, half the plots were slashed to mimic fire disturbance. We used generalised additive models to assess the treatment effects on diversity.
α-diversity increased and β-diversity decreased over time, regardless of the treatment. In disturbed plots where OED was added, OED had a negative effect on plot richness and diversity of rosette forbs. The effect on β-diversity was positive. When OED plants were removed, there was a temporary reduction in α-diversity following herbicide application with most measures returning to pre-treatment levels after 11 years. However, Xerochrysum subundulatum (a native forb) was severely affected by herbicide and did not recover fully.
OED affected grassland diversity, possibly through an allelopathic effect on recruitment. Despite OED abundance, the grassland community appeared resilient to invasion when undisturbed. Herbicides had a lasting effect on few native species. However, repeated applications will be required to prevent OED re-invasion.
The slow decline in α-diversity following OED invasion means that managers of subalpine grassland have time to address negative impacts on threatened species. Prevention and rapid response remain the most critical tools for OED management.
Keywords: α-diversity, allelopathy, β-diversity, functional diversity, herbicide effects, invasive species, species richness, turnover.
Introduction
Invasive non-native plants are recognised as a significant threat to biodiversity in a range of global assessments and Australian legislation (e.g. Environment Protection and Biodiversity Conservation Act 1999, IPBES 2023). The effects of invasive non-native plant species in natural ecosystems are extensive (e.g. reviews of Walker and Smith 1997 and Pyšek et al. 2012). The reported detrimental effects of invasive plants on resident native plant species are often associated with competition, allelopathy, and alterations to nutrient cycling, hydrology or fire regimes (Levine et al. 2003). Non-native plants may also have positive ecosystem effects (e.g. Clark et al. 2024).
Many of the reported impacts of non-native plants have been on diversity at different scales: alpha (α) diversity (the richness or abundance of groups of plants within a defined area), beta (β) diversity (community heterogeneity – the dissimilarity between samples in space or time), and gamma (γ) diversity (total species diversity at a broader scale, such as an ecosystem or region), though the impacts are variable. For instance, Flory and Clay (2009) found an increase in α-diversity measures after removal of the invasive grass Microstegium vimineum (Trin.) A. Camus but this was dependent on method of removal. Guido and Pillar (2017) similarly identified an increase in α-diversity after removal of Eragrostis plana Nees but removal did not return communities to a non-invaded state. Hejda et al. (2009) found that reductions in α-diversity were common after invasion by 13 non-native plant species in the Czech Republic but effects were dependent on the stature of invasive species, with tall invaders causing most impact. In contrast to many other studies, Meffin et al. (2010), in a field seed addition experiment over 6 years in a range of New Zealand montane habitats, found that the presence of Hieracium lepidulum (Stenstr.) Omang resulted in an increase or no change in a range of α-diversity measures; i.e. the already species-rich communities were able to accommodate another species. The effects of non-native species on β-diversity of plant communities have been far less studied than α-diversity effects but are again inconsistent. For example, Dyderski and Jagodziński (2021) found that the effect of non-native species on β-diversity in forests in western Poland depended on the species of invader and the habitat invaded. Kortz and Magurran (2019) identified an increase in α-diversity proportionate to the cover of four non-native species in the Brazilian Cerrado but found that this was offset by a reduction in β-diversity.
The effects of non-native species on diversity tend to be scale-dependent (Powell et al. 2013). For this reason, local effects on α- and β-diversity do not necessarily mean that impacts will occur at larger spatial scales (e.g. γ-diversity). However, anything that reduces diversity at local scales or interferes with diversity processes will potentially affect diversity more broadly. It is for this reason that many rare and threatened species are regarded as threatened by invasive plants (Kearney et al. 2019).
Despite the many documented effects of non-native plant invasions on diversity of natural areas, there have been relatively few experimental studies, with most effects being inferred by the abundance of the invader. Indeed, Stricker et al. (2015) found that more than half of the findings of papers describing invasive plant impacts were based on observation alone. They also found that most studies lasted for less than 1 year. Short-term observational studies risk mixing cause and effect, and identifying invaders that are ephemeral. In contrast, the need to quickly respond to new invaders, especially if eradication seems possible, precludes long-term experimental studies of impact. Ideally, both would occur; a precautionary rapid management response might be followed by studies of habitat suitability, likely range and identification of native species affected.
Experimental field studies of invasive species effects on diversity often involve removing the non-native species and recording the change in species composition. The effect is then implied. However, removal may create a disturbance, which may affect recovery or otherwise influence change. Field addition experiments have been few because of ethical considerations; i.e. the risk of introducing invasive species to uninvaded communities. Experiments on the competitive effects of invasive species are therefore often done in a glasshouse by comparing productivity of native species with and without the invader (e.g. see Vilà and Weiner (2004) for a review of experimental studies on invasive species competition).
Periodic disturbances such as fire and drought may also affect α-diversity and β-diversity and exacerbate or mitigate the effects of invasion. Fire often leads to temporary increases in α-diversity following colonisation of post-fire responders (e.g. Joner et al. 2021) while drought may have the opposite effect (e.g. Myers and Harms 2011). β-diversity has been found to decrease after a severe fire if disturbance-tolerant species become dominant (i.e. increasing homogeneity), but to increase in fire mosaics, which create complex diversity patterns (e.g. Perry et al. 2011). However, fire may have alternative effects on diversity where the vegetation is not fire-adapted (e.g. Pastro et al. 2011; Mahood and Balch 2019) or site productivity is low (Burkle et al. 2015).
Leucanthemum vulgare Lam. (ox-eye daisy, hereafter OED) is a shortly-rhizomatous perennial forb with a European origin. Its flowers, on stems to 0.8 m high, produce copious amounts of seed annually (Clements et al. 2004). The seed are long-lived and highly germinable, although some seed may be dormant or acquire dormancy if conditions are unfavourable (Clements et al. 2004). Seeds do not have appendages for long-distance dispersal by wind but readily attach to fur and so are likely to be dispersed by animals (McDougall et al. 2018b). The fecundity, competitiveness, and enduring seed-bank of OED have made it a target for control in many countries (e.g. Kashmir: Khuroo et al. 2010, Canada, and the United States: Cole et al. 2007; Stutz et al. 2018) and the subject of efforts to identify biological control agents (Stutz et al. 2021). The reasons for the success of OED in native vegetation and pastures outside its native range are poorly understood but may be related in part to its unpalatability (Clements et al. 2004) and evolution of morphological traits in its introduced range (Stutz et al. 2018). OED is thought to be favoured by disturbance and especially fire, based on observations in Kosciuszko National Park (KNP; McDougall et al. 2018b), Australia where seedlings were found to be suppressed by dense native vegetation but apparently released when the cover of native plants was reduced following fire. Based on space for time sampling, McDougall et al. (2018b) found that heavily-infested plots in Kosciuszko National Park had lower species richness, especially of rosette forbs, than lightly infested plots.
OED has been widely grown as an ornamental in Australia. The first Australian herbarium collections were made in Victoria and Tasmania in the 1880s. OED is now widespread in native vegetation in south-eastern Australia, growing from the coast to the Australian Alps, and is increasingly regarded as a threat to conservation values (McConnachie et al. 2015). Since its first record in Kosciuszko National Park in 1961, OED has spread throughout the northern part of the park where it is now locally dominant in grassland and woodland understoreys (McDougall et al. 2018b). The NSW National Parks and Wildlife Service has undertaken an annual program focused on containment since 2012, using herbicides to prevent spread from a containment zone and to protect threatened species (McConnachie et al. 2015). Within the containment zone, a mosaic of heavily, lightly and uninfested vegetation, OED is regarded as being ineradicable and no management is attempted. We were therefore permitted to use the containment zone to conduct removal and addition experiments in subalpine grassland, as any additional harm would be negligible. The experiments sought to assess: (1) the effects of OED on plant diversity at different spatial scales; (2) the effect of disturbance on OED cover; and (3) whether herbicide used to remove OED had a lasting effect on diversity. For the OED addition experiment, we expected:
a reduction in α-diversity measures because the tall stature of OED (compared with native grassland species), and its apparent rapid spread in sub-alpine vegetation are suggestive of a highly competitive species – the reduction in α-diversity might be especially severe for species that fill a similar niche to OED;
a reduction in spatial β-diversity because plots will become dominated by the few species that tolerate OED competition, hence driving greater similarity (and lower β-diversity) between invaded plots; and
a reduction in temporal β-diversity because, with the expected reduction in α-diversity, species losses should exceed gains at the plot level and invaded plots should then become more similar over time (in comparison with uninvaded plots).
We expected that the removal of OED would lead to temporary reductions in diversity measures because of herbicide impacts; however, with time, diversity would increase in plots without OED relative to those with OED, thus reversing the effects of the invader. In both addition and removal experiments, we expected disturbance to result in greater cover of OED, exacerbating the changes in diversity measures.
Materials and methods
Study area
The experimental site was located in Kellys Plain, a treeless valley at about 1260 m above sea level, in Kosciuszko National Park, New South Wales (35.813°S, 148.647°E; Fig. 1). The plain has low relief and is surrounded at higher elevations by woodland of Eucalyptus pauciflora Sieber ex Spreng. and Eucalyptus stellulata Sieber ex DC. Snow is common in winter months but is rarely persistent. The experimental plots were placed in grassland on a west-facing, gentle slope dominated by Poa clivicola Vickery, with sparse emergent shrubs of Hakea microcarpa R.Br. to about 1.5 m tall. When in flower, ox-eye daisy (OED; Leucanthemum vulgare Lam.) is by far the tallest herbaceous species.
Part of Kellys Plain in January 2015. The approximate position of the experimental area is indicated by an arrow. Trees dominate in the higher parts of the landscape. The white areas are dominated by Leucanthemum vulgare (ox-eye daisy).
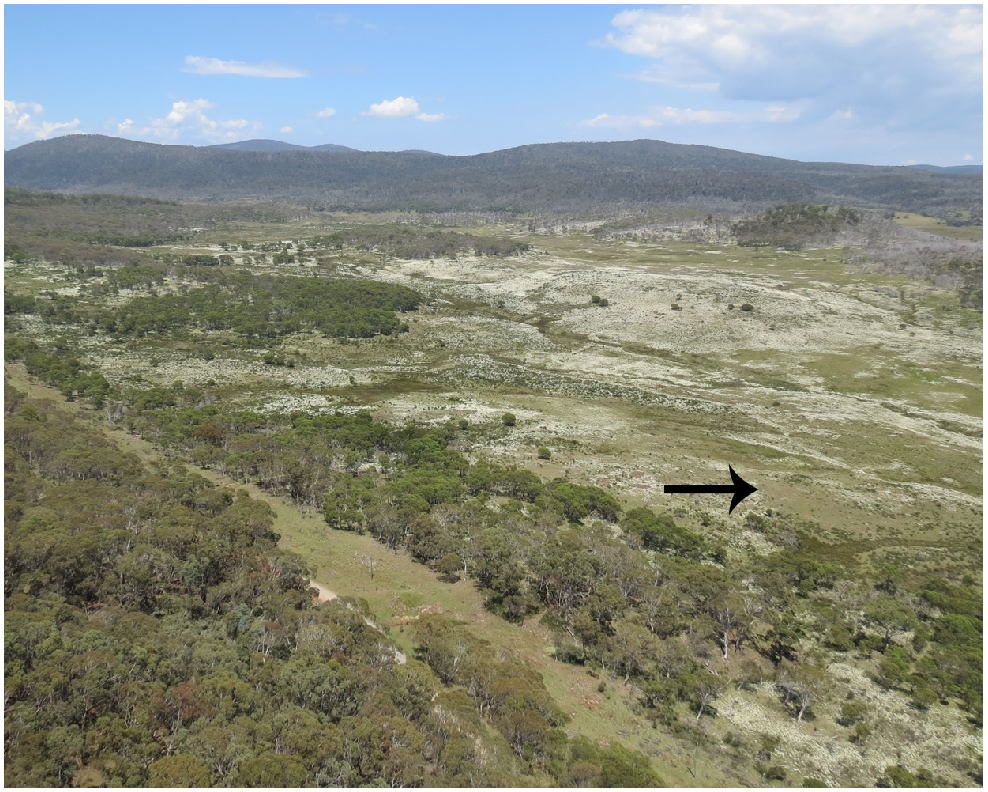
Feral horses are common in Kellys Plain; less common large herbivores include deer, kangaroos, and wallabies; none of these herbivores appear to eat OED. A wildfire burned the area in February 2007 but the severity of the fire was not assessed at that time. OED was first noticed in Kellys Plain in 2011. The site was burnt again in a wildfire in January 2020. The extent of burning from that fire was assessed in April 2020 where tall shrubs had been burnt but there was little evidence of burning in the ground layer. The experimental site was fenced in 2017 to exclude horses, which were damaging plot markers; the fenced area is approximately 10 ha. Based on records since 1887 at the town of Adaminaby, 25 km from Kellys Plain and 200 m lower, rainfall in the 12 months prior to sampling in January was mostly close to average (686 mm) for the duration of the experiments, except in 2019 and 2023 when there were large rainfall deficits (>200 mm).
Experimental design
In January 2013, 80 plots were randomly located by tossing a 1 m2 plastic frame within an area of grassland of approximately 10 ha. Opposite corners of the frame were permanently marked with metal pins if they contained no tall Hakea microcarpa shrubs and either no OED (40 plots) or at least 5% OED cover (40 plots). Once the 80 plots had been chosen, the plastic frame was placed over the pins for each plot and all species within the frame were recorded with an estimate of cover closest to the following ordinal scale: 1 = 5 × 5 cm (0.25%); 2 = 10 × 10 cm (1%); 3 = 20 × 20 cm (4%); 4 = 30 × 30 cm (9%); 5 = 50 × 50 cm (25%); 6 = 100 × 50 cm (50%); 7 = 100 × 75 cm (75%); and 8 = 100 × 100 cm (100%). The following aggregates of taxa were created because identification to species level was difficult or impossible when infertile: Asperula spp. (Asperula gunnii Hook.f., Asperula scoparia Hook.f.); Carex spp. (Carex breviculmis R.Br., Carex hebes Nelmes); other grasses (Anthosachne scabra (R.Br.) Nevski; Deyeuxia monticola (Roem. & Schult.) Vickery; Dichelachne micrantha (Cav.) Domin; Dichelachne rara (R.Br.) Vickery); other rosettes (Ajuga australis R.Br.; Pappochroma bellidioides (Hook.f.) G.L.Nesom; Solenogyne spp.; Velleia montana Hook.f.); Prasophyllum spp.; Pterostylis spp.; and Senecio spp. (Senecio gunnii (Hook.f.) Belcher, Senecio longipilus I.Thomps.). The recognition of species aggregates representing multiple species means that richness could be under-estimated at the plot level. However, when such species were identifiable it was clear that different species within an aggregate rarely occurred together; any effect of this on richness will be small.
After these baseline measurements, the plots were randomly assigned to two experiments such that mean species/per plot was approximately the same in treatments and controls. The two experimental set-ups are described below.
OED seed was applied to 20 plots at the rate of 7 g (about 20,000 seeds)/plot in April 2013. OED seed heads were collected from the study area in February 2013 and air-dried at room temperature for a month. Seeds were then removed from the heads, weighed, and placed in a separate envelope for each plot. The seeds were spread evenly through each addition plot and the surface soil of inter-tussock gaps scarified to aid burial. For the control treatment in this experiment, similar soil scarification was applied to inter-tussock spaces of 20 plots.
Herbicide (Grazon Extra: Picloram 100 g/L; Triclopyr 300 g/L; and Aminopyralid 8 g/L) was applied with a knapsack sprayer to 20 plots in February 2013; the entire plot and a 0.5 m buffer around each plot were sprayed. This herbicide targets dicotyledonous species, causing plant death and preventing seed germination for up to 2 years; it should not affect grasses. For the control treatment in this experiment, no herbicide was applied to 20 plots.
Approximately half the treatment and control plots in each experiment were randomly selected for a disturbance treatment after the 2015 measurement. Selected plots (and a 0.5-m buffer around each) were slashed with a brush cutter to within about 1 cm of the soil level in March 2015 to mimic the effect of fire as a disturbance; cut plant material was raked out of the plots.
While there were unplanned environmental changes in the study area during the trial (i.e. changes in herbivory after fencing and the fire of 2019/20), these affected all plots; we assume that the effects were uniform amongst treatments.
Measurements were made in early January in all years between 2013 and 2024 except 2020 when the study area was inaccessible because of a wildfire. Measurements were made by the same people each year (KM and JM) and the plots measured by each recorder were assigned at random prior to measurements to minimise differences between recorders in cover estimation. From 2014 onwards, OED seedlings were removed by hand at the time of measurement from plots where they should not occur (i.e. control plots for the addition experiment and herbicide-treated plots for the removal experiment). Measurements were also partly blinded, with the recorder knowing only which plots required the removal of OED seedlings. Six plots were lost because of trampling of markers by feral horses after the first measurement; data from these plots were not used and metal pins for the remaining 74 plots were then replaced with sturdier markers.
Data analysis
With explanatory variables added at different times and fire and drought potentially affecting response variables later in the time series, we chose generalised additive models (GAMs) for exploring relationships to ensure that non-linearity would be accounted for. GAMS were fitted using the package mgcv ver. 1.9 (Wood 2011) in R ver. 4.2.3 (R Core Team 2023), with model checking using gam.check. Means for the baseline measurements of each response variable of plots in 2013 were standardised and subsequent values for each plot within the same experiment adjusted by the same amount. The baseline plot data were then used only for calculation of temporal β-diversity (between 2013 and 2014). OED was excluded from all diversity analyses.
The primary research questions, which related to the effects of adding or removing OED on diversity, were investigated using GAMs of the form y ~ s(a, bs = ‘tp’) + b × c, where y = response variable, a = year of measurement, b is treatment, c is disturbance, s is the smoothing term for year, and bs = ‘tp’ refers to the thin plate regression splines applied (Wood 2003). Where necessary, a first order autocorrelation structure was included in a mixed GAM. The requirement for an autocorrelation structure was determined by examining the autocorrelation and partial autocorrelation functions for a lag of one. Higher order autocorrelation structures appeared to overfit the smooth term, making models potentially uninterpretable. Unbiased effect sizes of model variables (partial epsilon squared, εp2) were calculated using effectsize in R (Ben-Shachar et al. 2020). Rather than categorise effect size (e.g. εp2 < 0.02 = very small, εp2 ≥ 0.26 = large), we attempt where possible to provide context to the effect size by reporting differences between means.
The effect of disturbance alone was not germane to our expectations outlined in the Introduction and so model outputs for disturbance are reported only in Supplementary Material S1. However, a strong interaction between treatment and disturbance might indicate that the treatment had different effects under different disturbance regimes. Where there was evidence of a treatment effect and an interaction between treatment and disturbance, data were partitioned so that contrasting effects of treatment with and without disturbance could be explored. We arbitrarily chose a threshold of P < 0.01 for these post hoc tests.
Models for the following response variables were fitted:
α-Diversity
Plot species richness (the number of species in each plot); and
Functional diversity (based on life-form traits): Hill diversity (for q = 1) of erect forbs (with most biomass above the ground; e.g. Senecio spp.), rosette forbs (with most biomass in basal rosette leaves, e.g. Brachyscome decipiens Hook.f), spreading forbs (with most biomass close to the ground but not forming rosettes, e.g. Asperula spp.), shrubs and graminoids (grasses, sedges and rushes).
β-Diversity
Temporal (Sørensen dissimilarity between successive years for the same plots); and
Spatial (Sørensen dissimilarity between plots in the same year).
Functional diversity measures were calculated using the package hillR in R ver. 4.2.3 (R Core Team 2023). Hill diversity (for q = 1, the exponential of Shannon diversity) was chosen because it is linear (Chao et al. 2014) and could be calculated even if response variables were nil in some plots. Total functional diversity (Chao et al. 2014) and total forb diversity were not included in models because of their high correlation with plot species richness (R2 = 0.90 and 0.74, respectively). Sørensen dissimilarities were calculated using the package betapart in R ver. 4.2.3 (R Core Team 2023). The effects of disturbance on OED cover in each experiment where OED was present were similarly investigated using GAMs.
In line with recent criticisms of the use of P values in statistical inference (e.g. Wasserstein et al. 2019), we report probabilities as continuous and do not assign an α threshold (which has typically been 0.05).
Results
The total number of species recorded in plots in 2024 was greater than in 2013 for both experiments (Fig. 2); across all plots, there were an additional eight species recorded in 2022 (compared with 2013, a 13% increase in site (γ) diversity). The total species recorded in the addition experiment was greater in controls than in seeded plots for nine out of 10 measurements after 2013 and by 2024, there were seven more species recorded in control plots than in seeded plots. In the removal experiment, there was a large decrease in the total species recorded in herbicide-treated plots in the year after herbicide application in 2013 but the number of species had returned to the pre-treatment level by 2015 and to the level of control plots (with OED) by 2018 (Fig. 2). Thereafter, the difference in species number between control and treated plots was never greater than three.
The total number of species recorded in each experiment (addition and removal) for treatments and controls between 2013 and 2024.
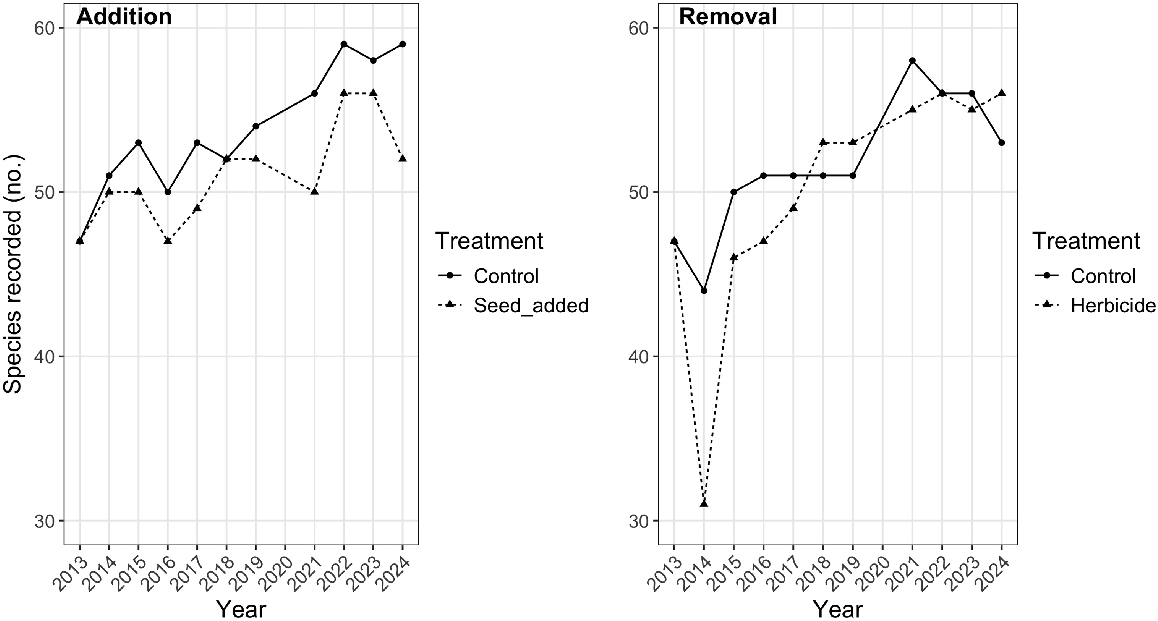
Most species were recorded more frequently in 2024 than in 2013 regardless of treatment and disturbance; 74% of species increased in % frequency (number of occurrences divided by number of plots × 100) with 26 species (32%) increasing by more than 10% and nine species increasing by more than 25% (Asperula spp. from 54 to 80%, Brachyscome decipiens from 15 to 42%, Festuca asperula Vickery from 1 to 30%, Geranium antrorsum Carolin from 11 to 64%, Hakea macrocarpa from 0 to 51%, Ranunculus graniticola Melville from 5 to 32%, and Rytidosperma penicillatum (Labill.) Connor and Edgar from 46 to 91%). In contrast, only 11% of species decreased in frequency over the course of the experiment, the largest decrease being 9% (Glycine latrobeana (Meisn.) Benth.).
Eleven non-native species other than OED were recorded during the experiment but they remained a minor component. In 2013, there was on average, 0.9 non-native species/plot. This increased to 1.7 non-native species/plot in 2024. The median cover of all non-native species other than OED in plots was less than 1% in all years, and 99% of non-native species records had a cover value of 3 or less (i.e. <about 5%).
Cover of OED
In control plots of the removal experiment, the median cover value of OED with and without disturbance was 4 (i.e. 10% cover) at the beginning (2013) and end (2024) of the experiment (Fig. 3). Seeded plots of the addition experiment (with no OED in 2013) reached a median cover value of 4 by 2018 but still had a median cover value of 4 in 2024. Our expectation that OED cover would increase with disturbance was not supported: in the removal experiment the modelled effect was negligible (P = 0.21, εp2 < 0.01); in the addition experiment there was some support in the model for a difference in means (P = 0.02, εp2 = 0.03) but the effect was negative (i.e. greater in undisturbed plots, Fig. 3).
Effect of adding OED seed
The smooth term of the GAM models (time) had a positive slope for plot richness and most functional diversity variables (P < 0.01) while the smooth term for modelled β-diversity variables had a negative slope (P < 0.01, Table 1); i.e. most α-diversity variables increased over time while β-diversity variables decreased over time. The changes were similar in both experiments (Tables 1 and 2).
Variable | Time | P | Effect size (εp2) | Int. (P) | |
---|---|---|---|---|---|
α-diversity | |||||
Plot richness (no.) | ↑ | <0.001 | −0.08 | 0.005 | |
Erect forbs | 0.005 | −0.02 | 0.89 | ||
Rosette forbs | ↑ | <0.001# | −0.16 | 0.001 | |
Spreading forbs | 0.06 | <+0.01 | 0.21 | ||
Shrubs | ↑ | 0.02 | −0.01 | 0.14 | |
Graminoids | ↑ | 0.06 | <−0.01 | 0.06 | |
β-diversity | |||||
Temporal | ↓ | <0.001 | +0.02 | 0.76 | |
Spatial | ↓ | <0.001# | +0.01 | <0.001 |
The slope of the smooth term (time) is indicated for P < 0.01: ↑ = positive (increasing); ↓ = negative (decreasing); # indicates that a first order auto-correlation structure was included in the model. Int. (P) indicates the model probability of the interaction term for treatment × disturbance interaction.
Variable | Time | P | Effect size (εp2) | Int. (P) | |
---|---|---|---|---|---|
α-diversity | |||||
Plot richness (no.) | ↑ | 0.08 | <+0.01 | 0.001 | |
Erect forbs | ↑ | 0.001 | +0.03 | <0.001 | |
Rosette forbs | ↑ | 0.50 | <+0.01 | 0.005 | |
Spreading forbs | ↑ | 0.10# | <+0.01 | < 0.001 | |
Shrubs | ↑ | 0.13 | <+0.01 | < 0.001 | |
Graminoids | ↑ | 0.03 | +0.01 | < 0.001 | |
β-diversity | |||||
Temporal | ↓ | 0.002 | −0.03 | 0.001 | |
Spatial | ↓ | 0.30# | <−0.01 | 0.21 |
The slope of the smooth term (time) is indicated for P < 0.01: ↑ = positive (increasing); ↓ = negative (decreasing); # indicates that a first order auto-correlation structure was included in the model. Int. (P) indicates the model probability of the interaction term for treatment × disturbance interaction.
There was support for a negative effect of seed addition on plot richness (P < 0.001, εp2 = 0.08), erect forb diversity (P = 0.005, εp2 = 0.02), rosette forb diversity (P < 0.001, εp2 = 0.16), and support for a positive effect of seed addition on spatial and temporal β-diversity (P < 0.001, Table 1). To give context to the effect sizes, the maximum difference between means for control and treatment in plot richness was 2.8 species in 2024 (equivalent to a 15% reduction), in erect forb diversity (the effective number of species) was 0.8 species in 2019, and in rosette forb diversity was 2.1 species in 2024 (a 34% reduction). While mean temporal β-diversity was consistently higher in control plots than in seeded plots throughout the experiment, the difference fluctuated, with peaks in control plots 8% above seeded plots in 2016 and 2023 but only 1% above in 2017. With high variance among plots, the effect size was unsurprisingly small (εp2 = 0.02). The difference in mean spatial β-diversity was highly variable, higher by about 3% in control plots in 2016 but lower also by about 3% in control plots in 2023. This variability is reflected in the very low effect size for spatial β-diversity (εp2 = 0.01). There was no support in the models for an effect of seed addition on diversity of spreading forbs, shrubs or graminoids (Table 1).
For plot richness and rosette forb diversity, the differences in means in the seed addition experiment were greater in disturbed plots than in undisturbed plots; the effect of seed addition was negative in disturbed plots, with effect sizes (εp2) of 0.18 and 0.20, respectively, and negligible for plot richness in undisturbed plots (Fig. 4). In 2022, when the effect was greatest, the mean reduction in plot richness for disturbed seeded plots was about four species and the reduction in rosette forb diversity was about three effective species (compared with control seeded plots). The effect of seed addition on spatial β-diversity was positive in disturbed plots (εp2 = 0.05) and negative in undisturbed plots (εp2 = 0.06, Fig. 4).
Generalised additive models, showing contrasts between disturbed and undisturbed plots, for variables with model support for an effect of addition of OED seed and a strong interaction between treatment and disturbance: (a) plot richness (number of species/plot); (b) rosette forb α-diversity; and (c) dissimilarity (%) = spatial β-diversity; 95% confidence bands are shown. The disturbance involved slashing of vegetation in 2015.
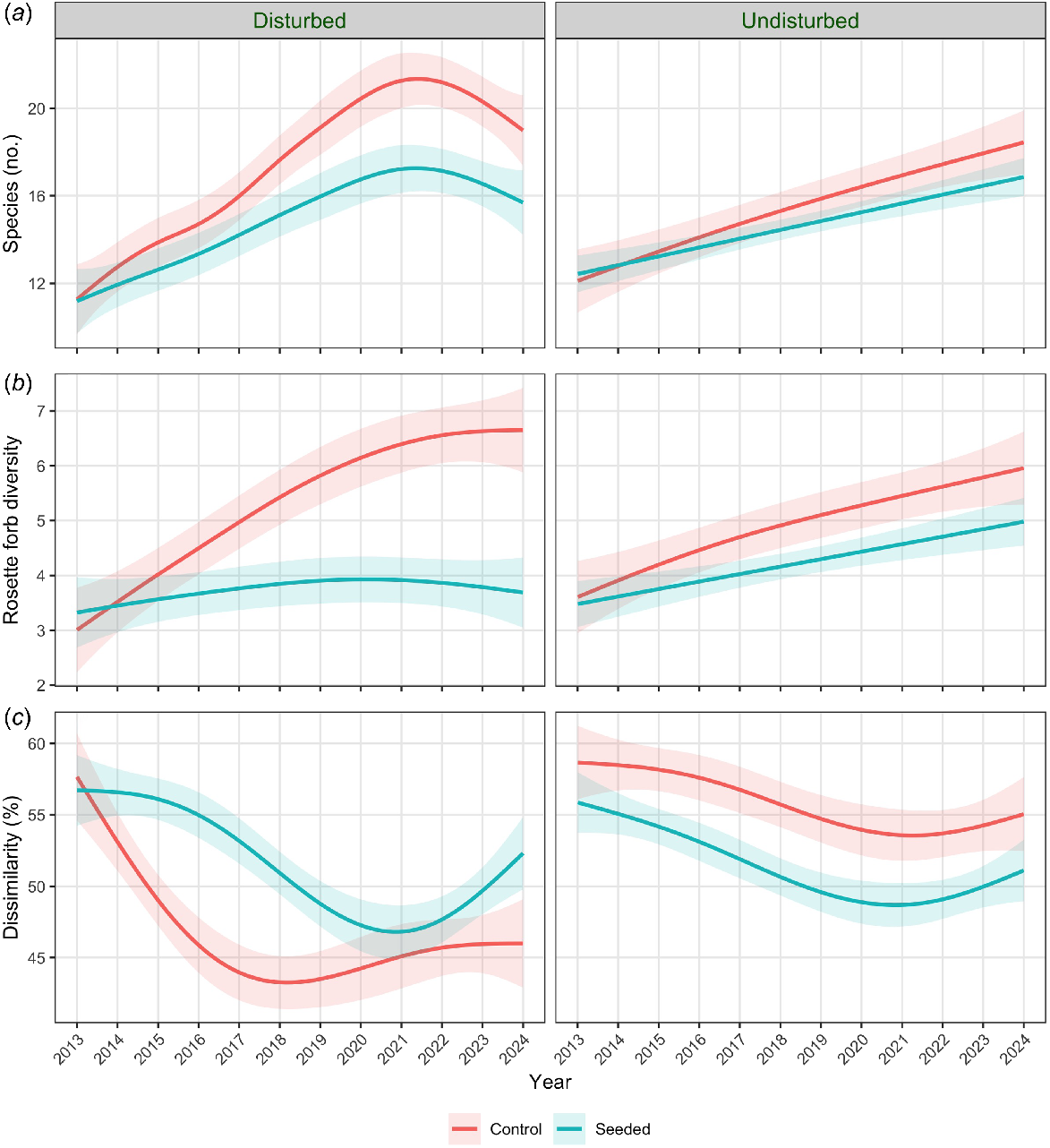
Effect of removing OED
There was support from the GAMs for a positive effect of removing OED on erect forb diversity (P = 0.001, εp2 = 0.03) and a negative effect of removing OED on temporal β-diversity (P = 0.002, εp2 = 0.03). Both variables had strong interactions between treatment and disturbance. P-values for other variables tested were high and effect sizes very small, indicating little support for an effect of removing OED (Table 2).
The effect of removing OED on erect forb diversity was greater in disturbed plots (εp2 = 0.05 with a positive effect) than in undisturbed plots (εp2 = 0.01 with a negative effect). There was a negative effect of removing OED on temporal β-diversity but the effect size was greater in undisturbed plots (εp2 = 0.21) than in disturbed plots (εp2 = 0.06, Fig. 5)
Generalised additive models, showing contrasts between disturbed and undisturbed plots, for variables with model support for an effect of OED removal (using herbicide followed by hand-pulling of seedlings) and a strong interaction between treatment and disturbance: (a) erect forb α-diversity; and (b) dissimilarity (%) = temporal β-diversity; 95% confidence bands are shown. The disturbance involved slashing of vegetation in 2015.
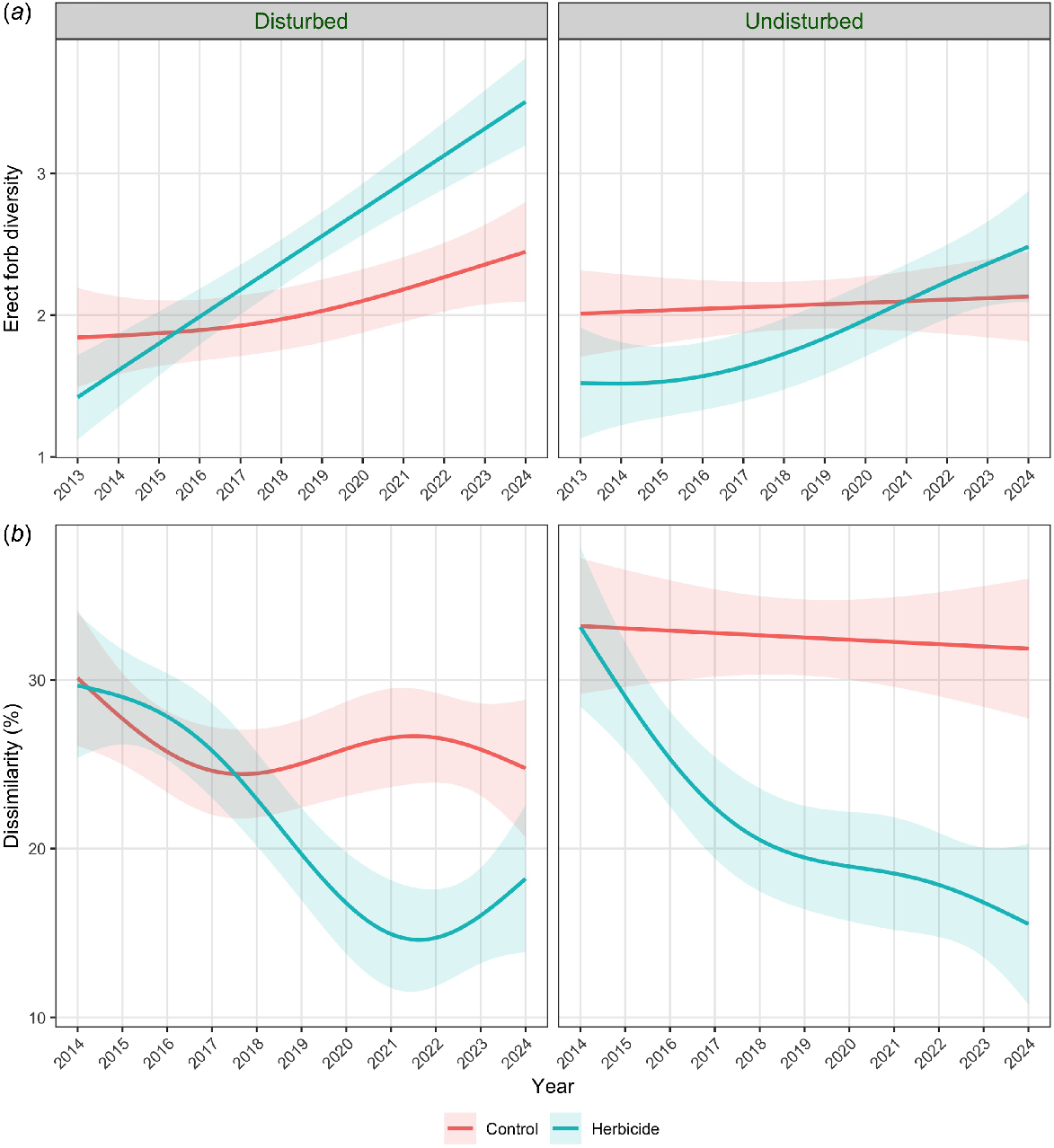
While there was little support from the GAM of plot richness for an effect of OED removal (Table 2), inspection of mean plot richness over time in disturbed and undisturbed plots suggests that in the final 3 years of the experiment, the removal plots were mimicking the addition plots (Fig. 6). For disturbed plots, there was a sharp decline in plot richness following herbicide application but mean richness in removal plots was comparable with control plots by 2017. However, after 2021, richness in removal plots exceeded that in control plots. In contrast, without disturbance, plot richness in removal plots was not comparable with control plots until 2021 but remained so thereafter. For the final 3 years of the removal experiment at least, disturbed plots without OED had greater plot richness than disturbed plots with OED, which was also the case in the addition experiment after about 2017.
Effect of herbicide
Of the 70 species or species aggregates recorded in the removal experiment, 37 (53%) had reduced frequency (relative to controls) one year after herbicide application. Of the five species or species aggregates with the largest reduction in frequency 1 year after herbicide application, all except Xerochrysum subundulatum (Sch.Bip.) R.J.Bayer had returned to pre-treatment frequency before the end of the experiment: Carex spp. (29% lower in 2014, returned to pre-treatment frequency in 2017); other rosettes (28% lower in 2014, returned to pre-treatment frequency in 2021); Rumex acetosella L. (29% lower in 2014, returned to pre-treatment frequency in 2022); and Senecio spp. (44% lower in 2014, returned to pre-treatment frequency in 2018). The frequency of Xerochrysum subundulatum was reduced by 75% in 2014 (when it was only recorded in one herbicide-treated plot that year); its frequency in treated plots was still 26% below its 2013 frequency (relative to its control) when the experiment was terminated in 2024. Herbicide application reduced the cover of the threatened species Calotis pubescens (F.Muell. ex Benth.) N.G.Walsh & K.L.McDougall from a value of 5 (c. 25% cover) in 2013 to 1 (<1% cover) in the one herbicide-treated plot, but it had returned to its pre-spray cover in 2019. The other threatened species, Glycine latrobeana, which tends to be cryptic, appeared to be unaffected by herbicide.
Discussion
While some of our expectations were met, the changes occurring naturally in the grassland were greater than those associated with our treatments; thus, it is important to consider treatment effects in the context of the strong background signal of change. Between 2013 and 2024, regardless of treatment, there were large increases in α-diversity measures at the plot scale, large increases in the frequency of occurrence of many species (including the appearance of shrub (Hakea macrocarpa) juveniles in more than half of the plots), and a 13% increase in species richness at the study area scale (γ-diversity). Both β-diversity measures in plots decreased. The reduction in spatial β-diversity implies that, despite new species colonising the plots, the plots were becoming more similar to each other. This would be consistent with the total species pool (γ-diversity) remaining stable but individual species becoming more abundant across the study area. While γ-diversity did apparently increase, this must have been more than offset by local increases in abundance. The decrease in temporal β-diversity was possibly driven by the same process of increasing species abundance, with each plot increasing in its likelihood of encompassing species present in the study area over time. The changes were evident before the area was fenced and before the fire of 2020; therefore, a reduction in grazing pressure or fire disturbance cannot explain them. The OED addition and removal experiments were placed into a highly dynamic landscape.
As expected, the addition of OED to the grassland resulted in a decrease in plot species richness. However, that decrease was mostly evident in disturbed plots. Higher plot richness in disturbed plots without OED was also evident in the final 3 years of the removal experiment. Given that OED did not have greater cover in disturbed plots than in undisturbed plots and that plot richness was increasing over time regardless of the presence of OED, lower plot richness when OED is present suggests that OED exerts an inhibitory pressure on recruitment, but only where there is a disturbance that removes substantial biomass. How might OED exert this pressure? Direct competition for resources seems an unlikely explanation because the effect was mainly detected in disturbed plots, which had more abundant light and space for colonisation than undisturbed plots. In addition, the cover of OED was less than 25% in most plots for the duration of the experiment; i.e. it was not especially dominant.
One possible explanation is allelopathy, the production of chemicals from plant parts such as flowers, pollen, stems, leaves, and roots that can affect many life stages of co-occurring species, including germination (see review of Zhang et al. 2021). For instance, Perera et al. (2023) found that plant parts of invasive Solidago species (especially leaves and flowers) inhibited germination and seedling survival of a range of native European species, and extracts from Conyza sumatrensis (Retz.) E.Walker shoots were found to inhibit the germination of Bidens pilosa L. (Ferreira et al. 2020). OED stems have been found to have herbicidal properties Magharri et al. (2015) and Ahmad et al. (2019) found that OED modified soils, potentially creating growing conditions more favourable to itself than to resident species. An allelopathic effect of OED following the decay of its stems and flowers may explain why Rowland (2012) could not detect allelopathic effects of drainage water from live OED plants on the growth of two native forbs in a glasshouse experiment. OED probably exerts competitive pressure in grassland because of its abundance and tall stature but its success as an invader may also be due to allelopathic effects on recruitment and survival of co-occurring species. In disturbed sites such as our slashed plots, the fallen stems would have been in close contact with the soil and potentially able to exert an allelopathic effect directly on emerging plants; in undisturbed sites, stems would have fallen on Poa tussocks and not be in contact with soil or germinants.
Of the life forms present in plots, the evidence for a negative effect of OED on α-diversity in the addition experiment was greatest for rosette forbs; however, this was more so in disturbed plots than in undisturbed plots. In a comparison of subalpine vegetation lightly and heavily infested with OED, McDougall et al. (2018b) similarly found that rosette forbs were amongst the species most affected by OED cover. While these species occupy the same inter-tussock niche as OED, it is not clear why they would be more affected by OED than other inter-tussock species, especially if the effect is largely allelopathic. In addition, rosette forbs of subalpine grasslands spend much of their life cycle in deep shade unlike erect or spreading forbs, which protrude above the grass cover; they should be the most able life form to withstand competition for light. Further research is required into the mode of OED effects. However, if rosette forbs are broadly most affected by OED, rare or locally endemic native rosette forbs may be at most risk from OED invasion, and these might be identified to assist with planning for a management response to invasion.
There was some evidence for an increase in spatial and temporal β-diversity following the addition of OED seed though the effect sizes were very small. With β-diversity declining naturally in the grassland, this means that OED slowed the decline. If, as we propose above, the reduction in β-diversity is because of the increasing abundance of resident species, the inhibition of recruitment by OED will reduce the abundance of some species and lead to greater β-diversity (dissimilarity) in seeded plots. That is, the small changes in β-diversity can be explained by the changes in α-diversity.
While the addition of OED resulted in a reduction in some α-diversity measures, the effects were not profound and at the study area level, species richness still increased in seeded plots over the course of the experiment, though not quite as much as in unseeded plots. Meffin et al. (2010) also found little effect on α-diversity over a 6-year period after the addition of Hieracium lepidulum to montane communities in New Zealand (and indeed, identified increases in diversity in some communities). The finding was suggested as support for the biotic acceptance theory of Stohlgren et al. (2006); i.e. that species-rich native communities will tend to support additional species and especially non-native species. Conversely, biotic resistance (e.g. Levine and D’Antonio 1999) applies when a community is full (i.e. saturated) and effectively resists the invasion of new species. In practice, these and other theories about invasion processes could plausibly co-occur in the same place at different stages of community development or following disturbance (Daly et al. 2023). Indeed, the Kellys Plain grassland was very accepting of additional species (including OED) at the plot scale over the course of the experiment. However, the presence of OED, with its apparent inhibiting effect on recruitment may ultimately make the grassland more resistant to colonisation of both native and non-native species.
We expected that removal of OED by herbicide application and subsequent hand pulling would reverse any adverse effects of OED presence after a brief negative effect from herbicide application. The GAMs for plot richness and functional diversity do not indicate a negative effect of removal and so the vegetation clearly recovered after herbicide application. However, apart from erect forbs, there was no clear positive effect either and for erect forbs, where the effect of OED seed addition was small, the experimental outcomes are not especially consistent. The closer examination of plot richness over time for the removal experiment (Fig. 6) does suggest that the effects of the two experiments were becoming comparable after 2021; with more time, an effect of removal may have become evident in the GAMs. For temporal β-diversity, the outcomes were somewhat similar for both experiments, with plot dissimilarity over time greater in plots containing OED. However, the death of many species after herbicide application is likely to have had an immediate negative effect on temporal β-diversity, which may have outweighed the small negative effect that OED presence had in the addition experiment. In any case, the large variance between plots meant that the effect size of both removal and seed addition on temporal β-diversity was small.
Curiously, OED cover did not change over the course of the experiment in plots that were already invaded and plateaued at a cover mostly between ~10–30%, 5 years after seed was introduced to uninvaded plots, regardless of disturbance. This suggests that OED can readily become an enduring part of the grassland community but resources and competition perhaps limit its ultimate abundance. Meffin et al. (2010) similarly found that the invader Hieracium lepidulum failed to reach dominance 6 years after addition (as seed) to a range of communities, reaching <5% cover in most plots to a maximum of about 50% cover. MacDougall et al. (2009) proposed that invasive species reach dominance when they either have a fitness advantage or fill a niche not currently filled by resident native species. In subalpine grasslands of Kosciuszko National Park, OED occupies inter-tussock spaces. That niche is already crowded with other, mostly native species, but it can clearly accommodate more species. OED does not appear to have an innate fitness advantage, as it co-exists with other inter-tussock species and does not readily reach the dominance of the tussock grasses of the community. However, disturbances such as fire and insect herbivory of the dominant grasses (Parida et al. 2015; McDougall et al. 2018a) may allow OED to push beyond its apparent ceiling of cover in grassland (i.e. cover value 4), as it has done in neighbouring woodland communities where it reaches a cover close to 100% (McDougall et al. 2018b).
The Kellys Plain grassland was undergoing substantial change in diversity between 2013 and 2024 regardless of the presence of OED. Recovery from the 2007 fires seems an unlikely explanation because McDougall et al. (2015) found that plot richness in subalpine grasslands such as Kellys Plain returned to pre-fire levels only 1 year after fire and species composition (species presence and abundance) had returned 10 years after fire. Alternatively, the increased diversity may be due to more favourable growing conditions associated with climate warming (e.g. higher temperatures, fewer frosts, less snow, elevated CO2). The increase in frequency of the tall shrub Hakea microcarpa is perhaps support for that, as shrubification is currently a feature of many mountains experiencing the effects of climate change (Myers-Smith et al. 2011). If the Hakea juveniles reach maturity, the study area may no longer support grassland in the coming decades. Indeed, the conversion of grassland to shrubland could have a greater effect on diversity than OED, as shrublands tend to be less species-rich than grasslands in this environment (McDougall and Walsh 2007).
Implications for OED management
Despite the effects of OED addition on diversity, we found that the overall impact was not profound over the 11 years of the experiment. OED cover seemed to plateau at 25% or less, OED presence did not apparently lead to local extinctions and the two threatened species present at the beginning were still present at the end. The addition of OED had most effect in disturbed plots, inhibiting a natural increase in plot richness and rosette forb diversity, but even that effect may diminish as the effect of disturbance disappears. This begs the question: is OED control necessary? We believe it is.
Our findings suggest that OED exerts its competitive pressure by inhibiting recruitment of some inter-tussock species. The changes were mainly detected in disturbed plots, habitat favourable to seed germination and establishment of disturbance-loving species. However, if OED does inhibit germination of resident species in general, recruitment failure as plants senesce may ultimately result in major α-diversity declines wherever OED is present. In addition, our study focussed on species diversity and not abundance. If species are persisting but becoming less abundant where OED is present, output of seed will be reduced as may the chance of recruitment. While the effect of OED on species diversity may be small over a period of 11 years, over several generations of the resident plants, there may be a substantial reduction in α-diversity.
Removing an invasive species need not remove its effect. For instance, Grove et al. (2012) found that the allelopathic effect of Cytisus scoparius (L.) Link lingered after its removal through effects on microbial symbionts. However, in the case of OED invasion, removal using herbicide and hand-pulling seemed to reverse its effects on α-diversity. This is encouraging for long term management of OED. While the effect of herbicide on overall diversity lasted for almost a decade, only one species (Xerochrysum subundulatum) was apparently still negatively affected by 2024. Fortunately, this forb is widespread and common in subalpine communities and so a broad herbicide spray program for OED is unlikely to change its conservation status. Given that the herbicide we used is designed to kill dicotyledonous species and that more than half the species in plots were dicots, it was surprising that so few immediate effects of herbicide application were observed. Many species with low cover in plots (including the critically endangered Glycine latrobeana) grow beneath the dominant grasses and shrubs of the grassland and will have been protected from the direct effects of herbicide spray.
The effects of herbicide we observed were at the plot scale, with re-invasion of affected species possible over short distances. The effects of broad-scale repeated herbicide application are less certain and will require careful monitoring to ensure that any benefits from removing OED are not offset by significant diversity losses, especially of rare or threatened species. If herbicide side-effects are too great, it may be necessary to tolerate OED presence where it is already abundant and widespread. If translocations of threatened species are required into habitat occupied by OED, the addition of soil amendments to counter allelopathy (Bieser et al. 2022) may reduce its effects on seed germination and seedling survival.
With the uncertainty of long-term effects of OED, we suggest that land managers focus on preventing establishment of satellite populations of OED, protecting assets such as threatened species, and employing citizen science for the early detection of new infestations. The imminent release of biological control agents (Stutz et al. 2021) may reduce the competitiveness of OED and allow small areas to be rehabilitated even if the local eradication of OED proves impossible.
Declaration of funding
Funding for this project was provided by the NSW Department of Climate Change, Energy, the Environment and Water as part of the Saving Our Species program.
Author contributions
KM prepared a first draft of the manuscript and analysed the data; KM and JM set up the experiment and collected the data; GW assisted with data collection and plot maintenance; all authors contributed to the preparation of the final manuscript.
Acknowledgements
We are grateful to Elouise Peach (NSW National Parks and Wildlife Service) for arranging the herbicide spraying, slashing of plots, and maintenance of the experimental area.
References
Ahmad R, Khuroo AA, Hamid M, Rashid I (2019) Plant invasion alters the physico-chemical dynamics of soil system: insights from invasive Leucanthemum vulgare in the Indian Himalaya. Environmental Monitoring and Assessment 191(Suppl 3), 792.
| Crossref | Google Scholar |
Ben-Shachar MS, Lüdecke D, Makowski D (2020) effectsize: Estimation of effect size indices and standardized parameters. Journal of Open Source Software 5(56), 2815.
| Crossref | Google Scholar |
Bieser JMH, Al-Zayat M, Murtada J, Thomas SC (2022) Biochar mitigation of allelopathic effects in three invasive plants: evidence from seed germination trials. Canadian Journal of Soil Science 102, 213-224.
| Crossref | Google Scholar |
Burkle LA, Myers JA, Belote RT (2015) Wildfire disturbance and productivity as drivers of plant species diversity across spatial scales. Ecosphere 6, 1-14.
| Crossref | Google Scholar |
Chao A, Chiu C-H, Jost L (2014) Unifying species diversity, phylogenetic diversity, functional diversity, and related similarity and differentiation measures through Hill numbers. Annual Review of Ecology, Evolution, and Systematics 45, 297-324.
| Crossref | Google Scholar |
Clark RE, Carter WA, Ku TCW, Seewagen CL (2024) Invasive plants as a foraging resource for insectivorous birds in a Connecticut, USA forest: insights from a community-level bird-exclusion experiment. Biological Invasions 26, 2081-2093.
| Crossref | Google Scholar |
Clements DR, Cole DE, King J, McClay A (2004) The biology of Canadian weeds. 128. Leucanthemum vulgare Lam. Canadian Journal of Plant Science 84, 343-363.
| Crossref | Google Scholar |
Cole DE, King JR, Oyarzun DA, Dietzler TH, McClay AS (2007) Experiences with invasive plant management and ecology in Alberta. Canadian Journal of Plant Science 87, 1013-1022.
| Crossref | Google Scholar |
Daly EZ, Chabrerie O, Massol F, Facon B, Hess MCM, Tasiemski A, Grandjean F, Chauvat M, Viard F, Forey E, Folcher L, Buisson E, Boivin T, Baltora-Rosset S, Ulmer R, Gibert P, Thiébaut G, Pantel JH, Heger T, Richardson DM, Renault D (2023) A synthesis of biological invasion hypotheses associated with the introduction–naturalisation–invasion continuum. Oikos 2023, e09645.
| Crossref | Google Scholar |
Dyderski MK, Jagodziński AM (2021) Impacts of invasive trees on alpha and beta diversity of temperate forest understories. Biological Invasions 23, 235-252.
| Crossref | Google Scholar |
Ferreira PJ, da Costa Zonetti P, Albrecht AJP, Rosset IG, Silva AFM, Albrecht LP, Vieira AH, Paulert R (2020) Conyza sumatrensis effect on Bidens pilosa (Asteraceae) seed germination. Botanical Sciences 98, 348-354.
| Crossref | Google Scholar |
Flory SL, Clay K (2009) Invasive plant removal method determines native plant community responses. Journal of Applied Ecology 46, 434-442.
| Crossref | Google Scholar |
Grove S, Haubensak KA, Parker IM (2012) Direct and indirect effects of allelopathy in the soil legacy of an exotic plant invasion. Plant Ecology 213, 1869-1882.
| Crossref | Google Scholar |
Guido A, Pillar VD (2017) Invasive plant removal: assessing community impact and recovery from invasion. Journal of Applied Ecology 54, 1230-1237.
| Crossref | Google Scholar |
Hejda M, Pyšek P, Jarošík V (2009) Impact of invasive plants on the species richness, diversity and composition of invaded communities. Journal of Ecology 97, 393-403.
| Crossref | Google Scholar |
IPBES (2023) Summary for policymakers of the thematic assessment report on invasive alien species and their control of the intergovernmental science-policy platform on biodiversity and ecosystem services. (Eds HE Roy, A Pauchard, P Stoett, T Renard Truong, S Bacher, BS Galil, PE Hulme, T Ikeda, KV Sankaran, MA McGeoch, LA Meyerson, MA Nuñez, A Ordonez, SJ Rahlao, E Schwindt, H Seebens, AW Sheppard, V Vandvik). IPBES secretariat, Bonn, Germany. 10.5281/zenodo.7430692
Joner F, Giehl ELH, Pillar VD (2021) Functional and taxonomic alpha and beta diversity responses to burning grasslands in southern Brazil. Journal of Vegetation Science 32, e13060.
| Crossref | Google Scholar |
Kearney SG, Carwardine J, Reside AE, Fisher DO, Maron M, Doherty TS, Legge S, Silcock J, Woinarski JCZ, Garnett ST, Wintle BA, Watson JEM (2019) The threats to Australia’s imperilled species and implications for a national conservation response. Pacific Conservation Biology 25, 231-244.
| Crossref | Google Scholar |
Khuroo AA, Malik AH, Reshi ZA, Dar GH (2010) From ornamental to detrimental: plant invasion of Leucanthemum vulgare Lam. (Ox-eye Daisy) in Kashmir valley, India. Current Science 98, 600-602.
| Google Scholar |
Kortz AR, Magurran AE (2019) Increases in local richness (α-diversity) following invasion are offset by biotic homogenization in a biodiversity hotspot. Biology Letters 15, 20190133.
| Crossref | Google Scholar | PubMed |
Levine JM, D’Antonio CM (1999) Elton revisited: a review of evidence linking diversity and invasibility. Oikos 87, 15-26.
| Crossref | Google Scholar |
Levine JM, Vilà M, D’Antonio CM, Dukes JS, Grigulis K, Lavorel S (2003) Mechanisms underlying the impacts of exotic plant invasions. Proceedings of the Royal Society Biological Sciences Series B 270, 775-781.
| Crossref | Google Scholar |
MacDougall AS, Gilbert G, Levine JM (2009) Plant invasions and the niche. Journal of Ecology 97, 609-615.
| Crossref | Google Scholar |
Magharri E, Razavi SM, Ghorbani E, Nahar L, Sarker SD (2015) Chemical composition, some allelopathic aspects, free-radical-scavenging property and antifungal activity of the volatile oil of the flowering tops of Leucanthemum vulgare Lam. Records of Natural Products 9, 538-545.
| Google Scholar |
Mahood AL, Balch JK (2019) Repeated fires reduce plant diversity in low-elevation Wyoming big sagebrush ecosystems (1984–2014). Ecosphere 10, e02591.
| Crossref | Google Scholar |
McConnachie AJ, Peach E, Turner PJ, Stutz S, Schaffner U, Simmons A (2015) The invasive weed ox-eye daisy, ‘Leucanthemum vulgare’ Lam. (Asteraceae): prospects for its management in New South Wales. Plant Protection Quarterly 30, 103-109.
| Google Scholar |
McDougall KL, Walsh NG (2007) Treeless vegetation of the Australian Alps. Cunninghamia 10, 1-57.
| Google Scholar |
McDougall KL, Walsh NG, Wright GT (2015) Recovery of treeless subalpine vegetation in Kosciuszko National Park after the landscape-scale fire of 2003. Australian Journal of Botany 63, 597-607.
| Crossref | Google Scholar |
McDougall KL, Wright GT, Burgess TI, Farrow R, Khaliq I, Laurence MH, Wallenius T, Liew ECY (2018a) Plant, invertebrate and pathogen interactions in Kosciuszko National Park. Proceedings of the Royal Society of New South Wales 140, 295-312.
| Google Scholar |
McDougall KL, Wright GT, Peach E (2018b) Coming to terms with Ox-eye Daisy (Leucanthemum vulgare) in Kosciuszko National Park, New South Wales. Ecological Management & Restoration 19, 4-13.
| Crossref | Google Scholar |
Meffin R, Miller AL, Hulme PE, Duncan RP (2010) BIODIVERSITY RESEARCH: Experimental introduction of the alien plant Hieracium lepidulum reveals no significant impact on montane plant communities in New Zealand. Diversity and Distributions 16, 804-815.
| Crossref | Google Scholar |
Myers JA, Harms KE (2011) Seed arrival and ecological filters interact to assemble high-diversity plant communities. Ecology 92, 676-686.
| Crossref | Google Scholar | PubMed |
Myers-Smith IH, Forbes BC, Wilmking M, Hallinger M, Lantz T, Blok D, Tape KD, Macias-Fauria M, Sass-Klaassen U, Lévesque E, Boudreau S, Ropars P, Hermanutz L, Trant A, Collier LS, Weijers S, Rozema J, Rayback SA, Schmidt NM, Schaepman-Strub G, Wipf S, Rixen C, Ménard CB, Venn S, Goetz S, Andreu-Hayles L, Elmendorf S, Ravolainen V, Welker J, Grogan P, Epstein HE, Hik DS (2011) Shrub expansion in tundra ecosystems: dynamics, impacts and research priorities. Environmental Research Letters 6, 045509.
| Crossref | Google Scholar |
Parida M, Hoffmann AA, Hill MP (2015) Climate change expected to drive habitat loss for two key herbivore species in an alpine environment. Journal of Biogeography 42, 1210-1221.
| Crossref | Google Scholar |
Pastro LA, Dickman CR, Letnic M (2011) Burning for biodiversity or burning biodiversity? Prescribed burn vs. wildfire impacts on plants, lizards, and mammals. Ecological Applications 21, 3238-3253.
| Crossref | Google Scholar |
Perera PCD, Chmielowiec C, Szymura TH, Szymura M (2023) Effects of extracts from various parts of invasive Solidago species on the germination and growth of native grassland plant species. PeerJ 11, e15676.
| Crossref | Google Scholar | PubMed |
Perry DA, Hessburg PF, Skinner CN, Spies TA, Stephens SL, Taylor AH, Franklin JF, McComb B, Riegel G (2011) The ecology of mixed severity fire regimes in Washington, Oregon, and northern California. Forest Ecology and Management 262, 703-717.
| Crossref | Google Scholar |
Powell KI, Chase JM, Knight TM (2013) Invasive plants have scale-dependent effects on diversity by altering species-area relationships. Science 339, 316-318.
| Crossref | Google Scholar | PubMed |
Pyšek P, Jarošík V, Hulme PE, Pergl J, Hejda M, Schaffner U, Vilà M (2012) A global assessment of invasive plant impacts on resident species, communities and ecosystems: the interaction of impact measures, invading species’ traits and environment. Global Change Biology 18, 1725-1737.
| Crossref | Google Scholar |
R Core Team (2023) ‘R: A language and environment for statistical computing.’ (R Foundation for Statistical Computing: Vienna, Austria) Available at https://www.R-project.org/
Stohlgren TJ, Jarnevich C, Chong GW, Evangelista PH (2006) Scale and plant invasions: a theory of biotic acceptance. Preslia 78, 405-426.
| Google Scholar |
Stricker KB, Hagan D, Flory SL (2015) Improving methods to evaluate the impacts of plant invasions: lessons from 40 years of research. AoB PLANTS 7, plv028.
| Crossref | Google Scholar |
Stutz S, Mráz P, Hinz HL, Müller-Schärer H, Schaffner U (2018) Biological invasion of oxeye daisy (Leucanthemum vulgare) in North America: pre-adaptation, post-introduction evolution, or both? PLoS ONE 13, e0190705.
| Crossref | Google Scholar | PubMed |
Stutz S, De Clerck-Floate R, Hinz HL, McClay A, McConnachie AJ, Schaffner U (2021) Host range and impact of Dichrorampha aeratana, the first potential biological control agent for Leucanthemum vulgare in North America and Australia. Insects 12, 438.
| Crossref | Google Scholar | PubMed |
Vilà M, Weiner J (2004) Are invasive plant species better competitors than native plant species? – evidence from pair-wise experiments. Oikos 105, 229-238.
| Crossref | Google Scholar |
Walker LR, Smith SD (1997) Impacts of invasive plants on community and ecosystem properties. In ‘Assessment and management of plant invasions’. Springer Series on Environmental Management. (Eds JO Luken, JW Thieret) pp. 69–86. (Springer: New York, NY, USA) 10.1007/978-1-4612-1926-2_12
Wasserstein RL, Schirm AL, Lazar NA (2019) Moving to a world beyond “p < 0.05”. The American Statistician 73(supp1), 1-19.
| Crossref | Google Scholar |
Wood SN (2003) Thin plate regression splines. Journal of the Royal Statistical Society Series B: Statistical Methodology 65, 95-114.
| Crossref | Google Scholar |
Wood SN (2011) Fast stable restricted maximum likelihood and marginal likelihood estimation of semiparametric generalized linear models. Journal of the Royal Statistical Society Series B: Statistical Methodology 73, 3-36.
| Crossref | Google Scholar |
Zhang Z, Liu Y, Yuan L, Weber E, van Kleunen M (2021) Effect of allelopathy on plant performance: a meta-analysis. Ecology Letters 24, 348-362.
| Crossref | Google Scholar | PubMed |