Probabilistic forecasts of extreme wind gusts
Paul Fox-Hughes
A
B
Handling Editor: Josephine Brown
Abstract
We document two damaging to destructive wind gust events in Tasmania, Australia, both of which resulted from synoptic-scale weather features and had widespread impacts. In documenting the events, we present a forecast diagnostic using ACCESS-CE (Australian Community Climate and Earth-System Simulator – City Ensemble), a high-resolution ensemble convection-allowing numerical weather prediction model introduced into the Bureau of Meteorology in recent years. In each case, the deterministic high-resolution operational forecast model, ACCESS-C, and especially its ensemble counterpart ACCESS-CE, provided very useful guidance of the potential for damaging to destructive wind gusts, both in respect of timing and of geographical range. The gust probability diagnostic summarised model guidance in a way that permitted rapid assessment by operational meteorologists, and communication to emergency managers of the hazard.
Keywords: ACCESS-C, diagnostics, downslope winds, ensemble numerical weather prediction, extreme winds, forecasting, severe weather, synoptic weather, visualisation.
1.Introduction
Damaging and especially destructive wind gusts are a hazard in many parts of Australia (Grace and Holton 1988; Turner et al. 2001; Allen et al. 2021; Bell et al. 2022; Webb and Park 2023) and globally (e.g. Brönnimann et al. 2012; Cao and Wang 2013; Clark and Gray 2018; Letson et al. 2021 among many others). Here, we follow the definitions of ‘damaging’ and ‘destructive’ used in the issue of public weather warnings in Tasmania by the Bureau of Meteorology. For the purposes of public weather warnings, ‘damaging’ wind gusts are those with speeds 100 km h−1 or more and destructive gusts are those of at least 125 km h−1. The Tasmanian damaging wind threshold is higher than for other, less climatologically windy, parts of Australia, which have a threshold of 90 km h−1. The ‘destructive’ threshold remains the same, however, as emergency management authorities agree that, regardless of the general windiness of a region, they respond to more frequent and more serious damage when wind gusts exceed this value. Such wind gusts can endanger life and affect a wide range of activities and industries (e.g. Fujita and Caracena 1977; Schüepp et al. 1994; Imai et al. 2002; Gibson 2007; Negrón-Juárez et al. 2010; Mitchell 2013; Konôpka et al. 2016; Repetto et al. 2017; Campbell et al. 2019; Zovko-Rajak et al. 2023). Forecasting the likelihood of their occurrence and that of other hazards is an important part of the work of operational meteorologists at the Australian Bureau of Meteorology. In this paper, we will generally refer to damaging and destructive wind gusts as ‘extreme’ for brevity.
A variety of phenomena give rise to extreme wind gusts (Golden and Snow 1991). Additionally, extreme wind gusts can occur in straight-line or rotating forms. Generally in the midlatitudes, including Tasmania, they occur as a mesoscale manifestation of the interaction of synoptic weather systems focussed by some mechanism on the mesoscale. For example, extreme wind gusts may occur as a result of a cold frontal passage focussed by the vertical circulation at the head of the front or other mechanisms (e.g. Schumacher et al. 2023), or in severe thunderstorms including those developing in association with cold frontal passages, or associated with low pressure troughs (Fox-Hughes et al. 1996, 2018 and numerous references therein; Louis 2018; Chernokulsky et al. 2022).
Recurrent and sometimes extreme downslope winds can occur in many regions, focussed by the coincidence of suitable topography and atmospheric stability conditions (Durran 1990). The downward flow induced by suitable topography, and synoptic or mesoscale conditions is often turbulent and gusty. Globally, particular regions are frequently subject to downslope winds, often in midlatitudes subject to prevailing winds across significant mountain ranges, such as indicated in Abatzoglou et al. (2021, see their fig. 5). Downslope winds in general have been occasionally documented in Australia as separate phenomena (e.g. Grace and Holton 1988; Pitts and Lyons 1989) or, increasingly, as compounding the impact of already potentially dangerous fires (e.g. Kepert et al. 2013; Peace et al. 2022; Fox-Hughes 2023). Abatzoglou et al. (2021), noted above, highlight the Great Dividing Range in south-eastern Australia and much of Tasmania as subject to quite frequent conditions conducive to downslope winds. Despite the high proportion of the landscape in Tasmania having substantially elevated topography and the regular occurrence of strong winds, extreme wind events have been infrequently documented in Tasmania, and mostly in association with severe thunderstorm events (Jones 1990 and several references therein; Fox-Hughes et al. 1996, 2018). Here, we document two cases of downslope winds in Tasmania associated with broad synoptic troughs.
High-resolution convective-allowing versions of numerical weather prediction model ensembles have been implemented in research and more recently operationally in the last two decades (e.g. Baldauf et al. 2011; Seity et al. 2011; Hagelin et al. 2017; Jirak et al. 2018). In recent years, the Australian Bureau of Meteorology has implemented operationally a suite of high-resolution deterministic (ACCESS-C, 1.5-km horizontal) and ensemble (ACCESS-CE, 2.2-km horizontal) models over priority subdomains of Australia (Cooper et al., Bureau Research Report APS3 ACCESS City Ensemble, in prep.). The Australian Community Climate and Earth-System Simulator – City Ensemble (ACCESS–CE) models are nested in the Bureau global ensemble model, ACCESS-GE. ACCESS-CE includes 1 control instance and 11 perturbed ensemble members. The estimation of wind gusts derived from ACCESS-C and ACCESS-CE is described in Ma et al. (2018) as the sum of modelled 10-m average wind speed and the maximum fluctuation of horizontal turbulence wind.
An important problem in the application of ensemble numerical weather prediction models is the representation of probabilistic data. It has received research attention as such models have become more widely used in operational meteorology (e.g. Evans et al. 2014; Roberts et al. 2019; Demuth et al. 2020; Ono 2023; Radford et al. 2023; Young and Grahame 2024). The use of high-resolution convective-allowing models in ensemble mode generates large amounts of data requiring sophistication in extracting key information in a timely fashion for operational use. Timely assimilation by meteorologists of numerical weather prediction and other guidance is particularly critical in the preparation and issue of severe weather warnings.
The representation of extreme wind gusts here offers a compact and easily intelligible means of identifying key features of forecast events, as well as forecast uncertainties. We display model output as viewed by operational meteorologists. This is for several reasons: it highlights the value and flexibility of not only the operational visualisation system used by Bureau operational staff (Visual Weather) but permits inspection of the operational and development tools for displaying high-resolution ensemble and other important data sources (e.g. satellite imagery). As such, the displays generally present data in formats other than SI units. In particular, operational meteorologists routinely contend with a mixture of wind speed units. Most displays of model and observational data present wind speed in knots, given the universal application of this measurement in aviation and marine services, important and long-standing users for Bureau and other national meteorological services. However, emergency management agencies and the broader community expect to see forecasts and warnings for wind presented in kilometres per hour (or miles per hour in some nations). Thus, depending on the focus, some wind speeds in this article are presented in knots (generally data displays) whereas impacts and warning thresholds are noted in kilometers per hour. Finally, times are indicated in Universal Co-ordinated Time (UTC), 10 h behind local Australian Eastern Standard Time (AEST) for the first (June 2022) case and 11 h behind Australian Eastern Daylight (Saving) Time (AEDT), in use for the second case (October 2023) study event.
2.Data and methods
2.1. Numerical weather prediction model data
The atmospheric model data used in these cases are from the ACCESS-C and ACCESS-CE high-resolution convective-allowing model, part of the ACCESS suite of numerical weather prediction models run routinely by the Bureau of Meteorology. ACCESS-C is the deterministic and ACCESS-CE the high-resolution ensemble instance of the third generation of ACCESS models implemented by the Bureau (APS3). The global model ACCESS-G and ACCESS-GE member of the suite is documented in Bureau of Meteorology (2020). The previous implementation of ACCESS-C, ACCESS-C2, is documented in Bureau of Meteorology (2018) and Roff et al. (2022). Currently available documentation for ACCESS-C3 and ACCESS-CE3 resides at: http://www.bom.gov.au/australia/charts/about/about_access.shtml with further description in Rennie et al. (2020). Briefly, ACCESS-C3 has horizontal resolution of ~1.5 km, 80 vertical levels to 38.5-km height and runs four cycles per day. It uses the UK Met Office Unified Model version 10.6+, with RA1-M physics configuration in mid-latitude domains. ACCESS-CE3 runs at 2.2-km horizontal resolution, again with 80 vertical levels, and the same model version and physics configuration as ACCESS-C3. The ensemble contains 12 members, run four times per day to 42 h.
ACCESS-CE runs over a number of domains around Australia, including a combined Victoria and Tasmania domain; all further reference to ACCESS-C and ACCESS-CE refers to the model run over this domain.
In the following case studies, ACCESS-CE is used to diagnose the probability of damaging wind gusts. This is done by calculating the percentage of ensemble members exceeding the damaging wind gust threshold. The resulting ‘ensemble probability’ is not a true representation of the real-world risk; this is because the ensemble probability has not been calibrated, and the ensemble system is subject to various biases (like under-dispersion). Regardless, the ensemble probability is a powerful way of distilling complex ensemble data into a usable field, to communicate hazard likelihood, intensity, timing, extent and uncertainties.
The ensemble probabilities represent the percentage of ensemble members that exceed the gust thresholds at any of the 10-min model timesteps during the 3 h prior to the time stated. To determine this, the maximum wind gust for each ensemble member is first processed for the 3-h period prior to the forecast time; then, the percentage of these ensemble member maxima that exceed the gust threshold yields the probability.
Broad-scale displays of synoptic patterns are derived from European Centre for Medium Range Weather Forecasts (ECMWF) data.
2.2. Observations
Several sets of observational data are used to validate ACCESS guidance in this study. The Tasmanian Bureau of Meteorology Automatic Weather Station (AWS) network was an important data source. AWS data are available at 1-min temporal resolution. The 10-min mean wind and 3-s wind gust strength and direction are reported routinely from 42 stations located around the state (see e.g. http://www.bom.gov.au/tas/observations/map.shtml). The locations of AWS from which data were used in this study are displayed in Fig. 1, which also displays Tasmanian topography, important in understanding the occurrence of downslope wind events in Tasmania. Other specific features and locations mentioned in this study are also identified in Fig. 1.
Topographic map of Tasmania, showing locations mentioned in the article and indicative position of Great Western Tiers, at the north of the central Tasmanian highlands. Regions in brown are above ~1000-m elevation. Map courtesy ListMap Tasmania.
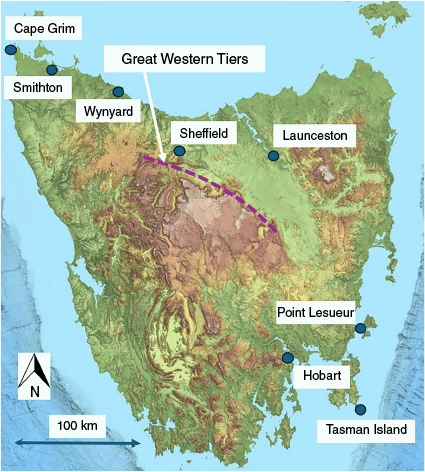
Mid-level water vapour satellite imagery (6.9-μm wavelength) can indicate the presence of very dry air at fairly low levels in the troposphere (e.g. Mills 2008a, 2008b; Fox-Hughes 2015). Himawari-9 imagery from the Japanese Meteorological Agency (Bessho et al. 2016) is available every 10 min over Tasmania and was useful to identify the occurrence of atmospheric wave activity. In particular, the deep descent of air in the lee of significant Tasmanian topography during both case studies is clear from satellite imagery.
Finally, Tasmanian emergency service authorities provided lists of incident callouts and requests for help during the events, at the resolution of individual addresses, to allow precise geo-location of wind gust damage. The electricity supply utility TasNetworks provided locality-level details of power outages during the events.
3.Events
In this section, we document two events, 11–12 June 2022 and 25 October 2023, that caused significant damage and community impacts in different areas of Tasmania.
3.1. 11–12 June 2022
On 11 June 2022, a broad low pressure trough lay over the Tasman Sea with a meridionally oriented high pressure system developing to its west over the Great Australian Bight (Fig. 2). In the vigorous south-westerly stream between these synoptic systems, an embedded low pressure centre passed Tasmania, further increasing the pressure gradient and associated winds over the state. The substantial pressure gradient between the high and trough, together with its unusually meridional orientation, resulted in strong south-south-westerly winds over Tasmania overnight on 11 June into the early morning of 12 June.
Bureau of Meteorology operational display of Mean Sea Level Pressure (MSLP), together with temperature (colour scale, °C) and wind barbs (knots, 1 knot = 1.852 km h–1) at 1500 m above ground level. Analysis from ECMWF, valid 12:00 hours UTC 11 June 2022.
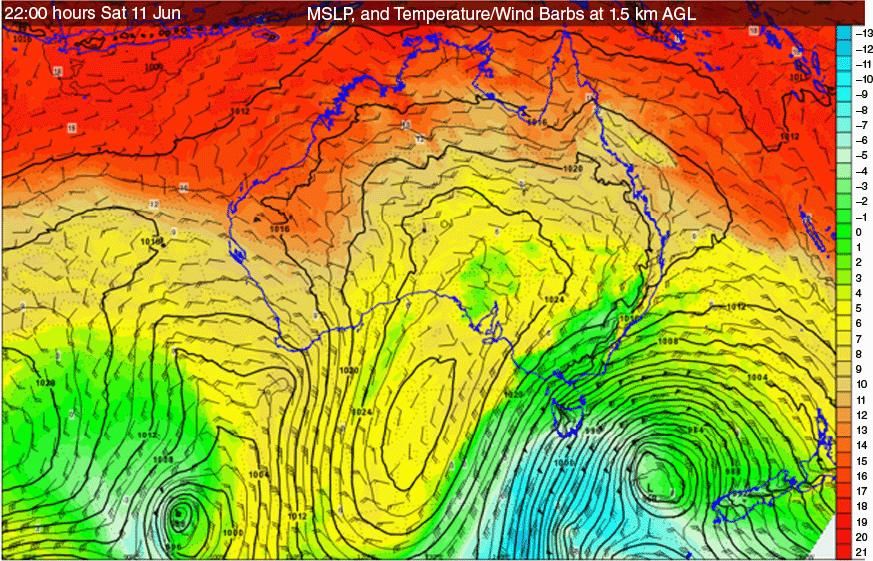
While the high remained some distance west of Tasmania, a developing subsidence inversion was evident in the mid-troposphere from ~700 hPa over western Tasmania at 12:00 hours UTC (22:00 hours AEDT) 11 June (Fig. 3). The blocking and diversion of the south-south-west airflow over the Tasmanian Central Plateau caused increase of windspeed on the descent of the air in its lee. A series of mountain bluffs forming the northern edge of the Central Plateau are known as the Great Western Tiers and range between 1200 and 1400 m in elevation. The Tiers thus provide a pronounced topographic feature over which downslope winds can occur.
ACCESS-G forecast of conditions over Tasmania and surrounds, valid 12:00 hours UTC 11 June 2022 with base time 00:00 hours UTC 11 June. (a) Wind barbs at 850 hPa (knots, 1 knot = 1.852 km h–1) and precipitation (shaded). The region of the Great Western Tiers is indicated by a blue dashed line. (b) Model aerological diagram at the location indicated by an orange dot in (a). The blue line in the aerological diagram denotes dewpoint temperature and the red line is the drybulb temperature.
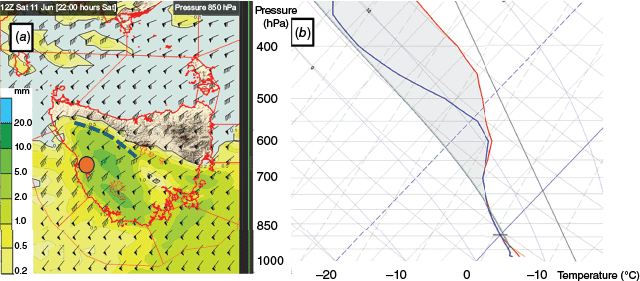
Fig. 3 displays ACCESS-G model output from the 00:00 hours UTC run on 11 June 2022, valid at 12:00 hours UTC 11 June. Fig. 3a displays a field of 850-hPa wind barbs (in knots), highlighting a region of enhanced windspeed over and close to the Tiers. Also displayed in Fig. 3a is the forecast 3-h accumulated precipitation field for that time. The vertical atmospheric profile displayed in Fig. 3b is located upstream of the Great Western Tiers, at a location denoted by an orange dot in Fig. 3a. The sounding indicates a layer of greater stability above 700 hPa, conducive to the development of downslope winds in the lee of the Tiers.
Strong descent in the lee of the Tiers can be seen in the vertical cross-section of winds and potential temperature from the ACCESS-C deterministic model 18:00 hours UTC run, valid 11:00 hours UTC 10 June (Fig. 4). The cross-section line cuts across the Tiers, from offshore on the west coast of Tasmania through to waters just off the central north coast (see Inset, Fig. 4). A vertical wave structure is evident downstream as the elevation of the airmass oscillates as it moves into Bass Strait.
ACCESS-C vertical cross-section through the region indicated by the arrow in the figure inset. The model base time is 18:00 hours UTC 10 June 2022, with validity time 11:00 hours UTC 11 June. Topography is indicated by grey shading. Wind barbs are horizontal winds through the depth of the cross-section and shading represents wind speed (knots, 1 knot = 1.852 km h–1), with stronger winds darker red through black. Potential temperature is indicated by yellow contours.
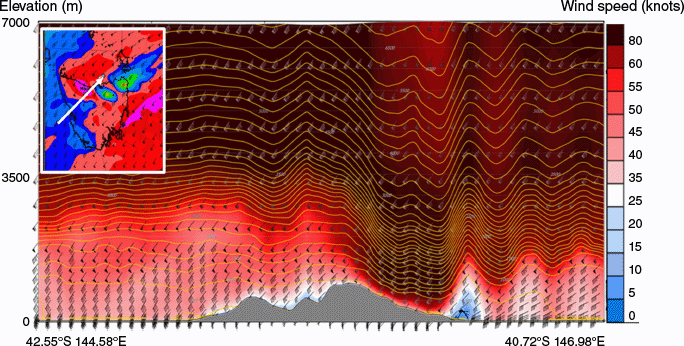
Deterministic ACCESS-C guidance suggested a maximum gust value of 186 km h−1 in the immediate lee of the Tasmanian Central Plateau (Fig. 5), at 14:00 hours UTC 11 June, with a broad area of potentially destructive gusts (yellow in Fig. 5) extending from the lee of the central Great Western Tiers through to the Midlands between Hobart and Launceston. Isolated pockets of destructive winds were also modelled on or in the immediate lee of elevated peaks in the north-east. Both areas are very significant in their potential for community impact. More widespread areas of damaging wind gusts (red in Fig. 5) can be seen across northern Tasmania and associated with elevated terrain in the south and west of the island.
ACCESS-C gust guidance for 14:00 hours UTC 11 June 2022, from the 18:00 hours UTC 10 June model run.
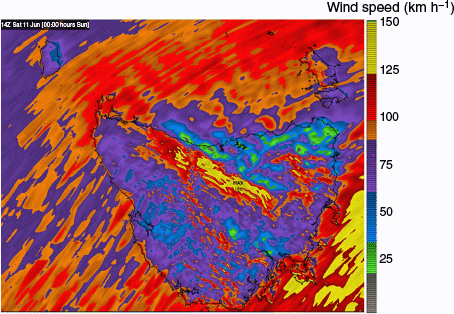
Assessment of the deterministic ACCESS-C model guidance performance is available from multiple sources. Himawari-9 water vapour imagery at 14:00 hours UTC 11 June is displayed in Fig. 6. Water vapour content is shaded grey through orange, the latter indicating very dry air through the depth of the troposphere. A band of very dry air is evident in the lee (i.e north-north-east) of the Tiers, supporting the model guidance of steep and sustained descent in this region. The alternating dark grey to orange and lighter grey bands over north-eastern Tasmania and offshore are consistent with the wave motion indicated in the ACCESS-C cross-section in Fig. 4. Outlined in red are regions modelled as likely to experience destructive wind gusts. Notable AWS wind gust observations at this time are listed in Table 1 in kilometres per hour. Gusts across northern Tasmania of 60–90 km h−1 were widespread, with 126 km h−1 observed at Sheffield in the immediate lee of the Great Western Tiers. Highly exposed Kennaook and Cape Grim observed 122 km h−1 and Tasman Island, also very exposed to south-westerly winds, recorded 150 km h−1. Although these two sites are not broadly representative owing to their exposure, the recorded wind gusts still usefully indicate of a very windy and potentially dangerous weather event. Fig. 7 displays observed and modelled wind gusts at Sheffield, the latter from the 12:00 hours UTC 10 June run of ACCESS-CE for the period 18:00 hours UTC 10 June through to 12:00 hours UTC 12 June. There is generally good correspondence between modelled and observed values, especially the timing of onset and decay of the strongest winds. There is a period during the peak of the event where the observed winds drop significantly. It is possible that the location of strongest downslope winds varied with broader atmospheric conditions (such as wind direction and stability) during this time.
Himawari-9 water vapour imagery at 14:00 hours UTC 11 June showing deep descent in the immediate lee (north-east) of the Great Western Tiers (orange shades). Area within the red outline indicates high likelihood of wind gusts greater or equal to 125 km h−1 at the same time, from the 00:00 hours UTC 11 June ACCESS-CE run.
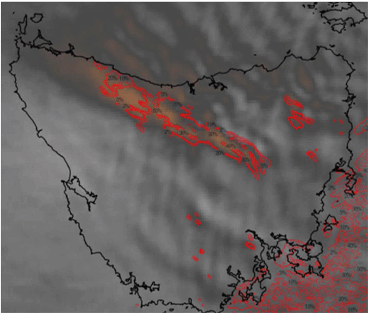
Location | Gust speed (km h−1) | Time (UTC), date | |
---|---|---|---|
Tasman Island | 150 | 12:32 hours, 11 June | |
Sheffield | 126 | 15:15 hours, 11 June | |
Cape Grim | 122 | 15:22 hours, 11 June | |
Wynyard | 94 | 16:51 hours, 11 June | |
Smithton | 93 | 11:58 hours, 11 June |
Meteogram of observed (black) wind gust strength (km h−1) at Sheffield AWS. Modelled wind gusts are also displayed from the ACCESS-CE 12:00 hours UTC 10 June run. The blue shaded area represents the interquartile range of ensemble values, with the blue line representing the 25th percentile, green the average and red the 75th percentile values.
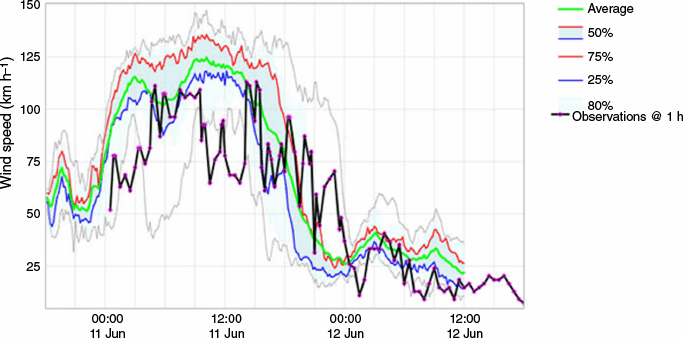
Impacts of the event were widespread. Fig. 8a shows the location of the 75 State Emergency Service (SES) requests for assistance, with a background of the percentage of ACCESS-CE ensemble members exceeding 100 km h−1 over Tasmania, valid at 14:00 hours UTC 11 June. In addition, FireComm (the Tasmania Fire Service communications centre) received 107 calls, mostly in the north-west. Electricity provider TasNetworks experienced 64 known outages, affecting some 20,266 customers (Fig. 8b).
Plots of wind impacts on 11–12 June 2022. (a) State Emergency callout locations shown as red dots; background is percentage of ACCESS-CE members with gusts exceeding 100 km h−1. Model validity time is 14:00 hours UTC 11 June, from the 00:00 hours UTC model run. (b) Locations and regions affected by electricity outages. Numbers denote outages within the same region, red shading denotes electricity network areas and subareas affected by outages. Images courtesy TasNetworks and Google Maps.
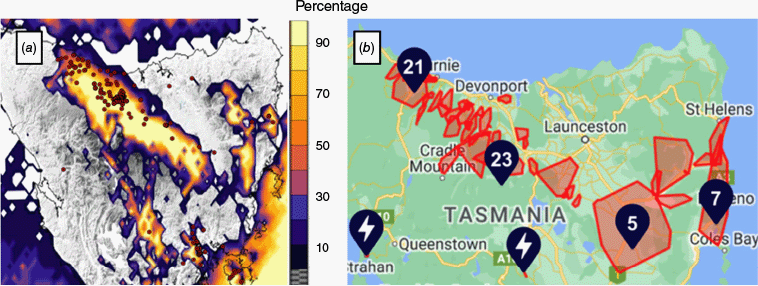
The deterministic model in this instance provided very useful guidance for the timing and extent of the extreme wind gusts. It is still valuable to examine the ensemble guidance, however.
The ACCESS-CE ensemble output for 13:00 hours UTC 11 June, from the 18:00 hours UTC 10 June run, showing wind gust strength is displayed in Fig. 9. Individual ensemble members are presented as ‘postage stamps’, allowing an overview of the variability of individual ensemble members. This can be useful but does not permit a quantitative assessment of, for example, the likelihood of severe weather thresholds being exceeded in particular areas or at specific locations.
Postage stamp display of wind gust output from ACCESS-CE, valid 13:00 hours UTC 11 June 2022, from the 18:00 hours UTC 10 June model run.
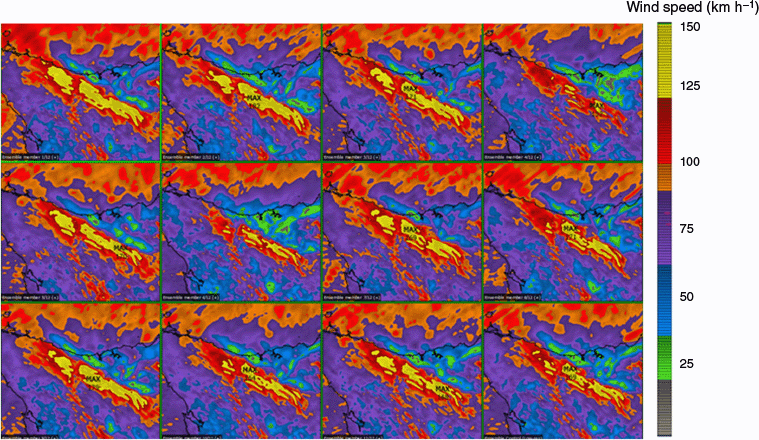
Each ensemble member provides generally similar guidance. Quantifying differences and similarities is difficult however, especially over time and when also examining related weather parameters (such as mean wind strength). This problem is magnified in time-constrained situations when meteorologists need to make consequential warning decisions.
By contrast, displaying the proportion of ensemble members that exceed a specific wind strength threshold at each available time step provides a more easily interpretable summary of the previous information. This results in the ability for operational meteorologists and emergency managers to take more rapid and comprehensive warning decisions. Such a summary is displayed in Fig. 8a, where the colour overlay highlights the proportion of ensemble members where wind gust strength exceeds 100 km h−1, again at 14:00 hours UTC 11 June.
The latter display, although easier to interpret than the larger amount of information contained in the previous postage stamp display, is also easier to assess against available observational evidence. The region of 50% or greater of ensemble members exceeding the 100 km h−1 gust threshold, displayed in orange and lighter colours, corresponds closely with the region of particularly dry air and by implication strong descent and potentially enhanced gustiness shown in the Himawari-9 water vapour (WV) imagery in Fig. 6.
Finally, we display contours of probability of potential destructive (≥125 km h−1) gusts, again at 14:00 hours UTC 11 June, in Fig. 6. This field is overlaid on the WV imagery for the same time, highlighting the good correspondence between the largest area of potentially destructive gustiness and strongest descent inferred from the corresponding Himawari-9 WV image.
3.2. Case study 2: 25 October 2023
This event is similar to the previous case but involved a different wind direction and affected a different part of Tasmania. We include it to demonstrate the variety of conditions resulting in the same hazard, and to highlight that the diagnostic approach used here is applicable to multiple situations. This situation is of particular interest as it occurs regularly, although mostly in lighter wind conditions. In addition, it affected Hobart, the capital city of Tasmania. With a population of ~250,000, Greater Hobart was thus highly exposed to the wind hazard in this (and similar) events.
A deep low pressure system moved south of Tasmania and a trough passed over the state during the early morning of 25 October (Fig. 10a). With the passage of the trough, strong westerly winds backed south-south-westerly. Ahead of this, strong winds descended from the central Tasmanian highlands into south-eastern Tasmania. A vertical profile over the Tasmanian central highlands at 15:00 hours UTC 24 October indicated the presence of a near-isothermal layer between ~850 and 700 hPa (Fig. 10b). This provided favourable conditions for wind speed enhancement in the lee of the highlands.
(a) Bureau of Meteorology operational display of mean sea level pressure (MSLP), together with temperature (colour scale, °C) and wind barbs (knots, 1 knot = 1.852 km h–1) at 1500 m above ground level. Analysis from ECMWF, valid 15:00 hours UTC 24 October 2023. (b) Model vertical sounding at 42.22°S 146.73°E, the location indicated by a white cross in (a). The blue line in the aerological diagram denotes dewpoint temperature and the red line is the drybulb temperature.
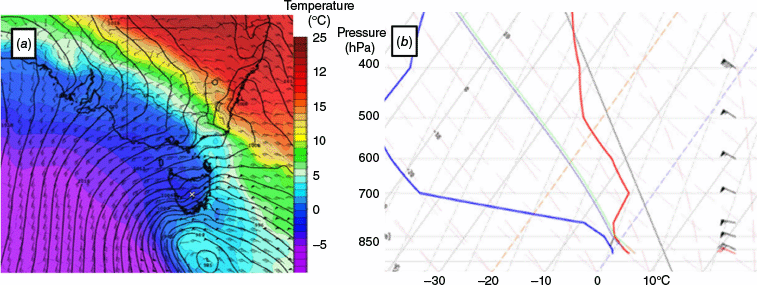
A cross-section of the modelled wind from the 00:00 hours UTC 24 October run of ACCESS-C is displayed in Fig. 11, valid at 14:00 hours UTC 24 October.
(a) ACCESS-C cross-section of horizontal winds between ~42.52°S 146.25°E and 43.35°S 148.33°E. Topography is displayed in black, and wind strength is represented by a blue (low)–red (high) colour scale, measured in knots. The location of Hobart is indicated by a blue star. Potential temperature is indicated by yellow contours. (b) Plan map of Tasmania showing the location of the cross-section in (a), with winds at 1500-m elevation (knots, 1 knot = 1.852 km h–1), coloured grey (low)–green–blue–purple (high) with winds barbs overlain. The orange dot indicates the position of Maria Island, referred to in Fig. 12.
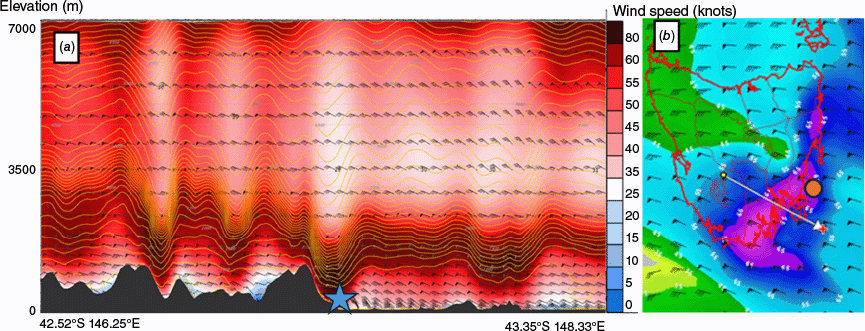
Strong winds (dark red) are evident trapped under the inversion (tightly grouped yellow isentropes). A strong downslope wave is present over Hobart, bringing 80-knot wind down to ~500 m above sea level (ASL). A large reduction in wind speed above the wave trough is a known downslope wind signature (Fovell et al. 2022). This is evident above Hobart with lighter winds (pale colour shading) above 2500-m elevation.
Several diagnostics proved valuable to visualise ensemble modelling for this event. The 06:00 hours UTC 24 October run of ACCESS-CE captured the timing and extent of elevated wind gusts. Fig. 12 displays a time series of modelled wind gusts for the location of the Point Lesueur (Maria Island) AWS, on Tasmania’s central east coast, between 06:10 hours UTC 24 October and 00:20 hours UTC 25 October. The full range of ensemble gusts (in knots) lies between the bounds of the light blue shading, with interquartile range between blue (25% of ensemble members) and red (75%) lines. The ensemble average gust during the period is shown in green. Wind gust observations from Point Lesueur are overlaid in black on the plot. Although model and observed wind gust strengths do not match exactly, there is good agreement between the timing of onset and decay of the strongest winds and in peak values.
Time series plot of Point Lesueur (Maria Island) AWS wind gust observations (black), together with envelope of ACCESS-CE ensemble forecast values, from the 06:00 hours UTC 24 October model run. The location of Maria Island is shown in Fig. 11b.
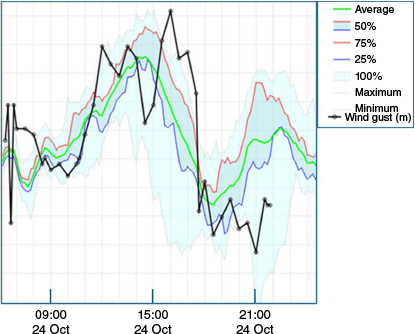
Another display of ACCESS-CE ensemble output from the same run (06:00 hours UTC 24 October) provides useful guidance on the likely extent of the wind event, in this case at a single time (although successive times can be looped within the display). Fig. 13 displays the probability in increasingly ‘hot’ colours of wind gusts exceeding 100 km h−1 at 16:00 hours UTC 24 October, based on the proportion of ensemble members with gusts exceeding the threshold value at each gridpoint. The guidance suggests a high probability of damaging (100+ km h−1) gusts, particularly in south-east Tasmania, including around the densely populated environs of Hobart, indicated by a blue star in Fig. 13. In addition, damaging gusts are predicted along the east coast, as observed by the Point Lesueur AWS observations in Fig. 12. Other AWSs in south-east Tasmania, however, did not record gusts as strong as this, including the Hobart City AWS. This is perhaps unsurprising given the sparsity of the AWS network and the localised nature of the regions of higher gust likelihood, as evidenced in Fig. 13.
Percentage of ACCESS-CE members with gusts exceeding 100 km h−1. Model validity time is 15:00 hours UTC 24 October, from the 06:00 hours UTC 24 October model run. Location of Hobart is indicated by a blue star.
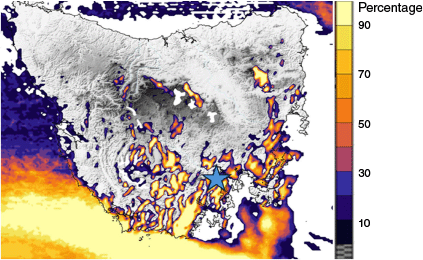
Evidence of the regions affected by damaging gusts is available from several sources, including Himawari-9 mid-level water vapour imagery. Fig 14a displays a water vapour image, valid at 15:00 hours UTC 24 October. As with the previous case, the colour enhancement highlights areas of dry air through a considerable depth of the atmosphere as grey-orange. These areas represent the descending part of atmospheric waves generated by the passage of the vigorous airflow over the topography of eastern and southern Tasmania. Alternating wave peaks and troughs are evident over eastern and especially south-eastern Tasmania, in locations corresponding well with the guidance from ACCESS-CE indicating a high probability of damaging gusts, associated with the descending arms of the wave train.
(a) Himawari-9 water vapour image, valid 14:00 hours UTC 24 October 2023. Purple-blue-green regions denote areas of high water vapour content, grey-orange areas denote areas of relatively low water vapour content. (b) As for (a), zoomed to focus on south-eastern Tasmania with the superposition of percentage of ACCESS-CE members with gusts exceeding 125 km h−1 (destructive wind gusts), valid for the same time, based on ACCESS-CE model run 12:00 hours UTC 24 October. (c) Locations and regions in south-eastern Tasmania affected by electricity outages overnight 24–25 October 2023. Image courtesy TasNetworks and Google Maps. (d) As per (b), with the addition of locations of State Emergency requests for assistance received overnight 24–25 October, indicated by red crosses.
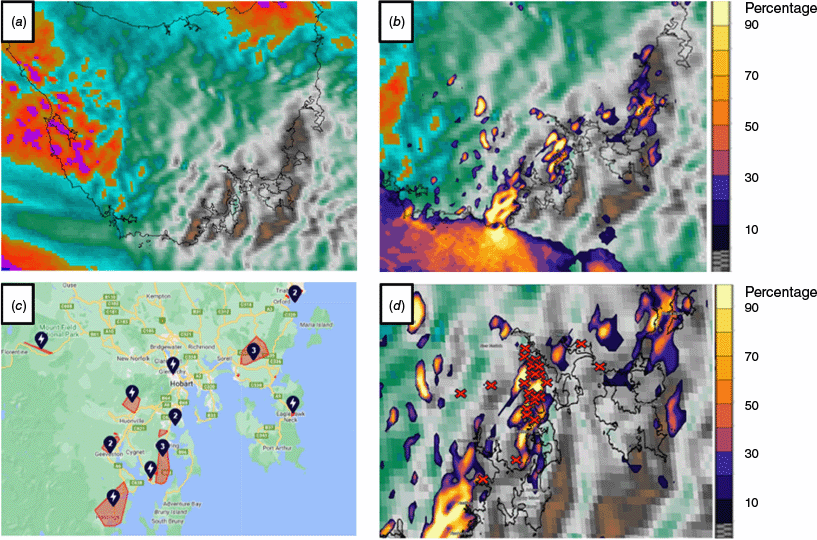
The extent of the correspondence can be seen by overlaying probability of destructive wind gusts (those in excess of 125 km h−1) from the ACCESS-CE guidance, in this case from the 12:00 hours UTC 24 October model run (Fig. 14b). Again, ‘hot’ (purple–orange–yellow) denotes increasingly high probability of, in this case, destructive wind gusts.
Importantly, impact reports correspond well with the areas highlighted as being at greatest risk from damaging and destructive wind gusts. Fig. 14c displays regions subject to power disruptions during the event. Most were south of Hobart, and all were in the south-east and around the lower east coast. At the peak of the event, more than 8000 power outages occurred. The Tasmanian SES registered ~40 incidents in south-eastern Tasmania overnight on 24 October, mostly around the western and southern suburbs of Hobart, as well as the Kingston and Blackmans Bay area south of Hobart. These included requests for assistance and 000 (emergency assistance) calls. Fig. 14d displays the locations of the SES incidents (red crosses) on top of the percentage of ACCESS-CE members with destructive gusts exceeding 125 km h−1 and the mid-level WV imagery at the time of peak gustiness (15:00 to 16:00 hours UTC 24 October). The bulk of the known impacts line up with areas of higher population density and wind gust risk. There are some impacts around Hobart that are on the periphery of the modelled area of destructive gust risk but are within the area of damaging gust risk.
4.Discussion
The cases of extreme wind gusts documented here were both driven by broad-scale synoptic troughs rather than convective-scale processes, and so in principle, given modern weather prediction tools, it is not difficult to forecast that damaging to potentially destructive wind gusts were possible. Greater difficulty arises in specifying the locations and timings of these downslope wind-generated gust events. Both case studies documented here were well forecast in respect of wind gust strength and general location, using guidance developed to display probability of damaging or destructive wind gusts in addition to a suite of other forecast guidance. These diagnostic guidance tools are available on operational meteorologist displays throughout Australia.
Initial warnings of damaging winds in both events were issued ~24 h in advance of damaging wind gust onset. In the case of 11–12 June 2022, a Severe Weather Warning was issued at 02:20 hours UTC Friday, warning of damaging winds over much of Tasmania from the morning of Saturday 11 June extending through until the morning of Sunday 12 June (see Fig. 15a for warning area). Note that for consistency with the remainder of this article warning times are listed in UTC but were issued indicating local time in the warning header and text. The following warning issued, at 06:59 hours UTC Friday, indicated potential for wind gusts up to 120 km h−1 north of the Central Plateau from late Saturday morning. The warning was further upgraded at 07:52 hours UTC Saturday, indicating the potential for destructive wind gusts in excess of 125 km h−1 to the north of the Central Plateau until early Sunday morning. On 23 October 2023, a Severe Weather Warning was issued at 02:12 hours UTC for much of Tasmania warning of damaging winds to 100 km h−1 from late morning on 24 June, easing early the following morning (see Fig. 15b for warning area). This warning was upgraded at 05:59 hours UTC 24 June, indicating that wind gusts could locally exceed 120 km h−1 on the morning of 25 June. For both events, warnings were issued many hours in advance of warning thresholds being met, covered the area of Tasmania affected by warning conditions and captured the severity of the conditions well.
Severe Weather warning maps issued (a) 12:20 hours AEST (02:20 hours UTC) 10 June 2022 and (b) 13:12 hours AEDT (02:12 hours UTC) 23 October 2023. Yellow indicates the area subject to the Severe Weather warning.
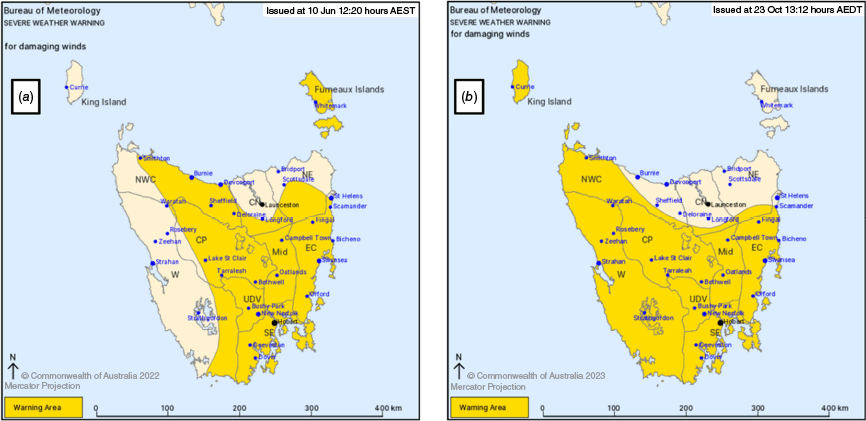
In both cases, the broad regions of strongest gusts were identified. This is pleasing but may be at least partly a consequence of the extreme nature of the events. The type of event is not uncommon, occurring several times per year, but the severity of the documented events was such that they would be expected only every few years, based on operational experience and feedback from emergency services. The rarity of such events (and other extremes) does challenge the calibration of numerical weather prediction models and highlights the value of training operational meteorologists and other users to understand the limitations and strengths of new model systems and generations. In more marginal cases, ACCESS-C and ACCESS-CE guidance will often be less definitive, and it will be more difficult to identify the occurrence and locations of damaging wind gusts.
Confirmation of the location and impacts of the gusts was based largely on surface reports. Water vapour imagery was very useful to identify regions of strong descent, from which the extent of potential damage could be inferred. WV imagery, however, does not permit an assessment of actual surface winds. That relies on measurement, damage reports or callout requests. Although Tasmania is well-served by AWS, the complexity of Tasmanian topography is such that the AWS network cannot be expected to cover all areas subject to any single phenomenon, including winds. There are likely localities that were subject to damaging or destructive gusts that were not recorded. It would be useful to examine private and organisational networks of wind measurements; however, there is no comprehensive catalogue of these, and no current means of ensuring the accuracy of the equipment. Damage reports are only called in when structures are impacted by wind gusts. Many structures will be resistant to damage up to quite extreme gusts. Some areas identified by ACCESS model guidance are in remote areas where no human structures are at risk, and therefore no damage reports will be received from those areas. During the early hours of 25 October 2023, for example, the far south-east coast of Tasmania likely experienced damaging and possibly destructive gusts. Very little built infrastructure is present there, however, and no damage was reported. Such areas are, nonetheless, still important to forecast and warn for. Tasmania is a popular hiking destination, with many walkers traversing remote and rugged regions, and is host to a range of rare and endemic vegetation communities, e.g. Huon pine stands, that may be vulnerable to downslope windstorms on occasion. Also, commercially valuable forest assets are often located in otherwise remote areas lacking infrastructure (Wood et al. 2008).
The quality of the modelling is important in these cases, as well as the ability to encapsulate the large amount of data that is available operationally. These data must be assimilated in short timeframes, ahead of and during sometimes critical weather events.
In both cases examined in this study, ACCESS-C and ACCESS-CE performed well, identifying timing and location of the major hazard. Stronger winds that occurred in smaller regions were likely not as well identified as those associated with larger-scale topographic features. Very strong winds also frequently occur in association with rapidly evolving, fast-moving and sometimes relatively small-scale weather phenomena such as mobile low pressure systems. The current generation of ACCESS high-resolution models may not resolve strong wind gusts associated with such systems. As above, the density of most surface observation networks is generally insufficient to resolve smaller-scale features, so verification of high-resolution modelling in such cases may also be difficult.
The above factors contributing to uncertainty in forecast guidance highlight the value of techniques to rapidly assess probabilistic information from ensemble models. The use of ensembles implies a significant increase in the amount of information requiring meteorologist attention. Particularly during rapidly changing, critical events, the time available to analyse such guidance and to make warning decisions is very limited. Diagnostic data displays need to succinctly and accurately summarise key information, including timing and severity of hazards. With the availability of ensemble guidance, a valuable added dimension, that of likelihood of occurrence, also requires attention. As ensemble numerical weather prediction (NWP) model output has become increasingly widely available in recent decades, several studies have investigated ways of encapsulating important information for decision making on the part of operational meteorologist and emergency management partners.
We suggest that, for the application of diagnosing wind gust strength and timing, the diagnostics presented above provide usefully succinct summaries of the important parameters of likelihood, severity and timing. As noted, they are readily deployed within the currently operational visualisation framework used within the Bureau of Meteorology, Visual Weather.
The approaches outlined above are useful for identifying potential damage from extreme wind gusts. More broadly, however, they can identify regions in which wind speed is likely to be enhanced owing to topographic or other factors. This is of use in, for example, fire weather forecasting, where forecasts may be requested by fire managers in difficult and variable terrain in remote areas where observational data are lacking. High-resolution numerical weather guidance of potentially enhanced winds is likely to be of particular value in these circumstances. Verification of such guidance can potentially be undertaken or enhanced in future with the use of satellite-based assessments of extreme wind events, such as described in Sills et al. (2020).
Historically, wind enhancement techniques were developed for individual sites or small regions to better forecast wind variability for high-priority activities such as aviation forecasting at airports. The advent of kilometre-scale numerical weather guidance, together with tools to highlight important variations and extremes within the large forecast datasets, allows those intensively developed techniques to be generalised to a broader forecast domain.
5.Conclusions
We have documented two cases studies of damaging to destructive wind gusts in Tasmania in recent years resulting from synoptic scale weather events. The type of event is not uncommon in Tasmania, occurring several times per year, but the severity of the documented events was such that they would be expected only every few years based on operational experience and feedback from emergency services. We used a recently developed diagnostic data display for the ensemble high-resolution ACCESS-CE model to summarise key aspects of the large amount of model data associated with both events, including timing, severity and extent. Although we have documented only two cases here, the diagnostic display is in routine use in the Tasmanian Office of the Bureau of Meteorology and is available for use in other forecast offices around Australia. Its simplicity of use and ability to summarise important guidance makes it useful for wind warning situations in Tasmania, and more broadly. The approach to displaying ensemble information is applicable to other high-impact weather phenomena also. We suggest that this and similar diagnostics are valuable aids to operational meteorologists faced with time-critical warning decisions in the lead-up to and during severe weather events.
Data availability
Bureau of Meteorology surface observations used in this study are available through Bureau Climate Data Service (https://reg.bom.gov.au/climate/data-services/). ACCESS-CE model data can be obtained from the Australian National Computational Infrastructure THREDDS service (https://thredds.nci.org.au/thredds/catalog/wr45/ops_aps3/access-vt/catalog.html).
Declaration of funding
P. Fox-Hughes received part-funding from the Australian Climate Service for this work.
Acknowledgements
Mika Peace and Simon Louis helpfully reviewed the manuscript of this paper. The anonymous reviewers provided helpful guidance in clarifying the content of this article.
References
Abatzoglou JT, Hatchett BJ, Fox‐Hughes P, Gershunov A, Nauslar NJ (2021) Global climatology of synoptically forced downslope winds. International Journal of Climatology 41(1), 31-50.
| Crossref | Google Scholar |
Allen JT, Allen ER, Richter H, Lepore C (2021) Australian tornadoes in 2013: implications for climatology and forecasting. Monthly Weather Review 149(5), 1211-1232.
| Crossref | Google Scholar |
Baldauf M, Seifer A, Förstner J, Majewski D, Raschendorfer M, Reinhardt T (2011) Operational convective-scale numerical weather prediction with the COSMO model: description and sensitivities. Monthly Weather Review 139(12), 3887-3905.
| Crossref | Google Scholar |
Bell SS, Dowdy AJ, Ramsay HA, et al. (2022) Using historical tropical cyclone climate datasets to examine wind speed recurrence for coastal Australia. Scientific Reports 12, 11612.
| Crossref | Google Scholar |
Bessho K, Date K, Hayashi M, Ikeda A, Imai T, Inoue H, Kumagai Y, Miyakawa T, Murata H, Ohno T, Okuyama A (2016) An introduction to Himawari-8/9 – Japan’s new-generation geostationary meteorological satellites. Journal of the Meteorological Society of Japan – Series II 94(2), 151-183.
| Crossref | Google Scholar |
Brönnimann S, Martius O, von Waldow H, Welker C, Luterbache J, Compo GP, Sardeshmukh PD, Usbeck T (2012) Extreme winds at northern mid-latitudes since 1871. Meteorologische Zeitschrift 21(1), 13-27.
| Crossref | Google Scholar |
Bureau of Meteorology (2018) NOC Operations Bulletin Number 114. APS2 Upgrade to the ACCESS-C Numerical Weather Prediction System. 22 February 2018. (Bureau of Meteorology: Melbourne, Vic., Australia) Available at http://www.bom.gov.au/australia/charts/bulletins/BNOC_Operations_Bulletin_114.pdf [Verified 29 October 2024]
Bureau of Meteorology (2020) NOC Operations Bulletin Number 125. APS3 upgrade of the ACCESS-G/GE Numerical Weather Prediction system. 20 October 2019. (Bureau of Meteorology: Melbourne, Vic., Australia) Available at http://www.bom.gov.au/australia/charts/bulletins/opsbull_G3GE3_external_v3.pdf [Verified 29 October 2024]
Campbell SL, Fox-Hughes PD, Jones PJ, Remenyi TA, Chappell K, White CJ, Johnston FH (2019) Evaluating the risk of epidemic thunderstorm asthma: lessons from Australia. International Journal of Environmental Research and Public Health 16(5), 837.
| Crossref | Google Scholar | PubMed |
Cao S, Wang J (2013) Statistical summary and case studies of strong wind damage in China. Journal of Disaster Research 8(6), 1096-1102.
| Crossref | Google Scholar |
Chernokulsky A, Shikhov A, Bykov A, Kalinin N, Kurgansky M, Sherstyukov B, Yarinich Y (2022) Diagnosis and modelling of two destructive derecho events in European Russia in the summer of 2010. Atmospheric Research 267, 105928.
| Crossref | Google Scholar |
Clark PA, Gray SL (2018) Sting jets in extratropical cyclones: a review. Quarterly Journal of the Royal Meteorological Society 144(713), 943-969.
| Crossref | Google Scholar |
Demuth JL, Morss RE, Jankov I, Alcott TI, Alexander CR, Nietfeld D, Jensen TL, Novak DR, Benjamin SG (2020) Recommendations for developing useful and usable convection-allowing model ensemble information for NWS forecasters. Weather and Forecasting 35(4), 1381-1406.
| Crossref | Google Scholar |
Evans C, Van Dyke DF, Lericos T (2014) How do forecasters utilize output from a convection-permitting ensemble forecast system? Case study of a high-impact precipitation event. Weather and Forecasting 29(2), 466-486.
| Crossref | Google Scholar |
Fovell RG, Brewer MJ, Garmong RJ (2022) The December 2021 Marshall Fire: predictability and gust forecasts from operational models. Atmosphere 13, 765.
| Crossref | Google Scholar |
Fox-Hughes P (2015) Characteristics of some days involving abrupt increases in fire danger. Journal of Applied Meteorology and Climatology 54, 2353-2363.
| Crossref | Google Scholar |
Fox-Hughes P (2023) Synoptic and mesoscale aspects of exceptional fire weather during the New Year period 2019–20 in southeastern New South Wales, Australia. Weather and Forecasting 38(11), 2237-2252.
| Crossref | Google Scholar |
Fox-Hughes P, Bally J, Hubbert G (1996) A tornadic thunderstorm in northwest Tasmania: 22 November 1992. Australian Meteorological Magazine 45, 161-75.
| Google Scholar |
Fox-Hughes P, Barnes-Keoghan I, Porter A (2018) Observations of a tornado at an Automatic Weather Station in northern Tasmania. Journal of Southern Hemisphere Earth Systems Science 68, 215-230.
| Crossref | Google Scholar |
Fujita TT, Caracena F (1977) An analysis of three weather-related aircraft accidents. Bulletin of the American Meteorological Society 58(11), 1164-1181.
| Crossref | Google Scholar |
Gibson B (2007) Examination of a density current with severe winds and extensive dust: South Australia case study 2 April 2005. Australian Meteorological Magazine 56(4), 267-283.
| Google Scholar |
Golden JH, Snow JT (1991) Mitigation against extreme windstorms. Reviews of Geophysics 29, 477-504.
| Crossref | Google Scholar |
Hagelin S, Son J, Swinbank R, McCabe A, Roberts N, Tennant W (2017) The Met Office convective‐scale ensemble, MOGREPS‐UK. Quarterly Journal of the Royal Meteorological Society 143(708), 2846-2861.
| Crossref | Google Scholar |
Imai T, Fujii T, Tanemoto K, Shimamura T, Maeda T, Ishida H, Hibino Y (2002) New train regulation method based on wind direction and velocity of natural wind against strong winds. Journal of Wind Engineering and Industrial Aerodynamics 90(12–15), 1601-1610.
| Crossref | Google Scholar |
Jirak IL, Clark AJ, Roberts B, Gallo B, Weiss SJ (2018) Exploring the optimal configuration of the high resolution ensemble forecast system. In ‘25th Conference on Numerical Weather Prediction’, 3–8 June 2018, Denver, CO, USA. Presentation 14B.6. (American Meteorological Society) Available at https://ams.confex.com/ams/29WAF25NWP/webprogram/Paper345640.html [Recorded presentation and manuscript]
Kepert JD, Fawcett RJ, Tory KJ, Thurston W (2013) Applications of very high resolution atmospheric modelling for bushfires. In ‘Extended abstracts, Annual Conference of the Australasian Fire and Emergency Services Council and the Bushfire Cooperative Research Centre’, 2–5 September 2013, Melbourne, Vic., Australia. (Bushfire Co-operative Research Centre: Melbourne, Vic., Australia) Available at https://www.bushfirecrc.com/sites/default/files/managed/resource/kepert_jeff.pdf
Konôpka B, Zach P, Kulfan J (2016) Wind – an important ecological factor and destructive agent in forests. Central European Forestry Journal 62(2), 123-130.
| Crossref | Google Scholar |
Letson FW, Barthelmie RJ, Hodges KI, Pryor SC (2021) Intense windstorms in the northeastern United States. Natural Hazards and Earth System Sciences 21(7), 2001-2020.
| Crossref | Google Scholar |
Louis SA (2018) A warm-front triggered nocturnal tornado outbreak near Kiama, NSW, Australia. Journal of Southern Hemisphere Earth Systems Science 68, 147-164.
| Crossref | Google Scholar |
Ma Y, Dietachmayer G, Lu W, Rikus L, Sgarbossa D, Steinle P (2018) Diagnose wind gusts from high resolution NWP modelling over mountainous regions. Bureau Research Report BBR029. (Bureau of Meteorology) Available at http://www.bom.gov.au/research/publications/researchreports/BRR-029.pdf
Mills GA (2008a) Abrupt surface drying and fire weather. Part 1: overview and case study of the South Australian fires of 11 January 2005. Australian Meteorological Magazine 57, 299-309.
| Google Scholar |
Mills GA (2008b) Abrupt surface drying and fire weather. Part 2: a preliminary synoptic climatology in the forested areas of southern Australia. Australian Meteorological Magazine 57, 311-328.
| Google Scholar |
Mitchell SJ (2013) Wind as a natural disturbance agent in forests: a synthesis. Forestry 86(2), 147-157.
| Crossref | Google Scholar |
Negrón‐Juárez RI, Chambers JQ, Guimaraes G, Zeng H, Raupp CF, Marra DM, Ribeiro GH, Saatchi SS, Nelson BW, Higuchi N (2010) Widespread Amazon forest tree mortality from a single cross‐basin squall line event. Geophysical Research Letters 37, L16701.
| Crossref | Google Scholar |
Ono K (2023) Clustering technique suitable for Eulerian framework to generate multiple scenarios from ensemble forecasts. Weather and Forecasting 38(6), 833-847.
| Crossref | Google Scholar |
Peace M, Greenslade J, Ye H, Kepert JD (2022) Simulations of the Waroona fire using the coupled atmosphere–fire model ACCESS-Fire. Journal of Southern Hemisphere Earth Systems Science 72(2), 126-138.
| Crossref | Google Scholar |
Pitts RO, Lyons TJ (1989) Airflow over a two‐dimensional escarpment. I: observations. Quarterly Journal of the Royal Meteorological Society 115(488), 965-981.
| Crossref | Google Scholar |
Radford JT, Lackmann GM, Goodwin J, Correia J, Jr, Harnos K (2023) An iterative approach toward development of ensemble visualization techniques for high-impact winter weather hazards: part I: product development. Bulletin of the American Meteorological Society 104(9), E1630-E1648.
| Crossref | Google Scholar |
Rennie S, Rikus L, Eizenberg N, Steinle P, Krysta M (2020) Impact of Doppler radar wind observations on Australian high-resolution numerical weather prediction. Weather and Forecasting 35(2), 309-324.
| Crossref | Google Scholar |
Repetto MP, Burlando M, Solari G, De Gaetano P, Pizzo M (2017) Integrated tools for improving the resilience of seaports under extreme wind events. Sustainable Cities and Society 32, 277-294.
| Crossref | Google Scholar |
Roberts B, Jirak IL, Clark AJ, Weiss SJ, Kain JS (2019) Postprocessing and visualization techniques for convection-allowing ensembles. Bulletin of the American Meteorological Society 100(7), 1245-1258.
| Crossref | Google Scholar |
Roff G, Bermous I, Dietachmayer G, Fernon J, Fraser J, Lu W, Rennie S, Steinle P, Xiao Y (2022) APS2-ACCESS-C2: the first Australian operational NWP convection-permitting model. Journal of Southern Hemisphere Earth Systems Science 72(1), 1-18.
| Crossref | Google Scholar |
Schüepp M, Schiesser HH, Huntrieser H, Scherrer HU, Schmidtke H (1994) The winterstorm ‘Vivian’ of 27 February 1990: about the meteorological development, wind forces and damage situation in the forests of Switzerland. Theoretical and Applied Climatology 49, 183-200.
| Crossref | Google Scholar |
Schumacher RS, Childs SJ, Adams-Selin RD (2023) Intense surface winds from gravity wave breaking in simulations of a destructive macroburst. Monthly Weather Review 151(3), 775-793.
| Crossref | Google Scholar |
Seity Y, Brousseau P, Malardel S, Hello G, Bénard P, Bouttier F, Lac C, Masson V (2011) The AROME-France convective-scale operational model. Monthly Weather Review 139(3), 976-991.
| Crossref | Google Scholar |
Sills DML, Kopp GA, Elliott L, et al. (2020) The Northern Tornadoes Project: uncovering Canada’s true tornado climatology. Bulletin of the American Meteorological Society 101, E2113-E2132.
| Crossref | Google Scholar |
Turner J, Lachlan‐Cope TA, Marshall GJ, Pendlebury S, Adams N (2001) An extreme wind event at Casey Station, Antarctica. Journal of Geophysical Research: Atmospheres 106(D7), 7291-7311.
| Crossref | Google Scholar |
Webb C, Park J (2023) Channelling flows in the Hunter Valley. Journal of Southern Hemisphere Earth Systems Science 73(2), 194-211.
| Crossref | Google Scholar |
Wood MJ, Scott R, Volker PW, Mannes DJ (2008) Windthrow in Tasmania, Australia: monitoring, prediction and management. Forestry: an International Journal of Forest Research 81(3), 415-427.
| Crossref | Google Scholar |
Young MV, Grahame NS (2024) The history of UK weather forecasting: the changing role of the central guidance forecaster. Part 7: operational forecasting in the Twenty‐first Century: graphical guidance products, risk assessment and impact‐based warnings. Weather 79(3), 72-80.
| Crossref | Google Scholar |
Zovko-Rajak D, Tory KJ, Kepert JD (2023) A case study of South Australia’s severe thunderstorm and tornado outbreak 28 September 2016. Journal of Southern Hemisphere Earth Systems Science 73(2), 178-193.
| Crossref | Google Scholar |