Enhancing deep sowing success: genetic diversity in mesocotyl and coleoptile length, and field establishment of oats (Avena sativa)
Angelia Tanu



A
B
C
Abstract
Early and deep sowing practices have revolutionised Australian winter cropping. Oats (Avena sativa) are the only winter-cereal with a mesocotyl, potentially allowing them to successfully emerge from deep sowing. This study examined the genetic differences in mesocotyl and coleoptile length, the effect of temperature on these traits, and undertook a field validation of deep-sown oats compared to selected wheat (Triticum aestivum) and barley (Hordeum vulgare) genotypes. A controlled environment experiment on 195 oat genotypes revealed long combined mesocotyl and coleoptile lengths (112–219 mm) with significant genotypic variation. A further controlled environment study compared the mesocotyl and coleoptile lengths of 42 genotypes across four temperatures (15–30°C). This revealed that temperatures exceeding 20°C reduced coleoptile and mesocotyl length by 3.7 mm and 1.1 mm per °C. Five field experiments compared the emergence of 19 oat, four wheat, and two barley genotypes from deep (110 mm) and shallow sowing (40 mm). Oats had greater emergence at depth compared to wheat and barley genotypes. The results indicate that oats are highly suited to early and deep sowing conditions due to their long mesocotyl and combined mesocotyl and coleoptile length.
Keywords: coleoptile, deep sowing, early sowing, emergence, establishment, genetic diversity, mesocotyl, oats.
Introduction
Germination and emergence are vital steps in the growth and yield of any crop. Without the establishment of sufficient plant density all subsequent processes of vegetative and reproductive growth are limited. In rainfed farming systems like Western Australia where germination and establishment of a crop rely on the timing of autumn rainfall, the timing of the first significant autumn rainfall (break-of-season) is critical in determining the timing of opportunities to sow crops (Hunt et al. 2019). Across these regions, the break-of-season is becoming less predictable and shifting later in the season (Pook et al. 2009; Dey et al. 2019; Flohr et al. 2021).
Farmers in these regions are exploring various solutions including earlier sowing to address the variability in the break-of-season. Early sowing promotes deeper root growth, prolongs crops’ access to water resources, and reduces soil evaporation (Passioura and Angus 2010; Kirkegaard et al. 2015; Rasmussen and Thorup-Kristensen 2016). Sowing early-adapted wheat in early April instead of the conventional early-mid-May timing could increase mean yields by 25% and maximise water-use-efficiency with less predictable in-season rainfall patterns (Hunt et al. 2019). The benefits are even greater when compounded at the level of the whole farm. However, early sowing relies on sufficient rainfall early in the season, or crops need to be sown dry (Fletcher et al. 2016). To address this, deeper sowing (50–200 mm) is being explored as a management option because it allows the seedlings to access sub-surface soil moisture (deeper than 50 mm) from summer or small autumn rainfall events (also known as false-breaks), increasing the germination rate (Rich et al. 2022; Zhao et al. 2022). Deep sowing requires crops to successfully emerge from depth.
In wheat (Triticum aestivum), the ability of seedlings to emerge from depth is determined by the length of the coleoptile. The coleoptile is a sheath-like modified leaf that protects the first leaf and shoot apex in monocotyledons, assisting the emergence of the first leaf through the soil (Srivastava 2002). Modern wheat genotypes bred for maximum yield during the ‘Green Revolution’ possess semi-dwarfing genes known as Rht-B1b and Rht-D1b (Ellis et al. 2002). These semi-dwarfing genes reduce plant height and coleoptile length by reducing cell size (Richards 1992). This reduction in coleoptile length of the semi-dwarf wheats results in poor crop emergence and establishment when sown deep, negatively impacting grain production and quality (Zhao et al. 2022). Except for the old Halberd genotype, DPIRD (2023a) showed that all 20 Australian modern wheat genotypes tested exhibit coleoptile lengths less than 100 mm, with the majority being medium (60–80 mm). Halberd is an older wheat genotype that lacks the semi-dwarfing genes, giving it a longer coleoptile length of 110 mm (Rebetzke et al. 1999). In recent years, there has been a renewed focus on alternative dwarfing genes (Rht 13 and Rht18) that reduce plant height but do not decrease coleoptile length. These long-coleoptile wheat genotypes can emerge from deep sowing and provide increased yield (Rebetzke et al. 2007).
Oats (Avena sativa) have a unique morphology compared to the other winter cereals that means they may be able to unlock the potential of deep sowing. Unlike the other winter cereal crops (e.g. wheat; barley, Hordeum vulgare), oats possess an elongated mesocotyl (Hoshikawa 1969). The mesocotyl is the internodal tissue between the coleoptile node and the scutella node on the seed. Together, the mesocotyl and coleoptile facilitate penetration of the shoot tip through the soil during germination (Mgonja et al. 1993). Thus, the mesocotyl in oats effectively extends the distance between the seed and the tip of the coleoptile compared with wheat and barley. Although mesocotyls are a common feature among grass species, not all species exhibit elongation. For example, maize (Zea mays) and rice (Oryza sativa) demonstrate mesocotyl elongation; however, in wheat and barley, the mesocotyl does not elongate at all (Hoshikawa 1969). Long mesocotyls in deep-sown rice and maize contribute to faster seedling establishment, enhanced root anchorage and early vigour, and a lowered risk of sowing failure due to drought in water-scarce conditions. In contrast, seedlings with shorter mesocotyls experience difficulties with emergence when sown deeply (Turner et al. 1982; Mgonja et al. 1993; Ohno et al. 2018).
While it is known that oats possess an elongated mesocotyl, there is limited knowledge of genetic variation in this trait. On a small set of genotypes, DPIRD (2023a) measured the total coleoptile and mesocotyl lengths (combined length) and documented that oats have either very long (100–120 mm) or extremely long (more than 120 mm) combined lengths, no maximum length was provided. Radford and Key (1993) studied 16 oat genotypes and found that oats have long combined lengths, of up to 252 mm, with no significant differences between genotypes. Thus, there is a need to examine a diverse range of oat genetics for differences in mesocotyl elongation. Furthermore, although it makes sense that the mesocotyl will allow oats to better emerge from depth compared to wheat and barley, there is little or no evidence in the literature.
Early autumn sowing will likely expose seedlings to higher soil temperatures, potentially exceeding 30°C in the Western Australian wheatbelt during sowing (Sudmeyer et al. 2016). Increased temperature significantly influences mesocotyl and coleoptile lengths. Radford and Key (1993) found that 15°C is the optimal temperature for the longest combined length and observed a decline in combined length beyond 20°C, mainly attributed to reduced coleoptile length. Similarly, Radford (1987) and Rebetzke et al. (1999) documented a significant decrease in coleoptile length amongst the wheat genotypes as temperatures increase above 15°C. Additionally, Radford and Key (1993) observed genetic variation at 30°C, suggesting a potential solution to poor emergence at moderately high soil temperatures, with positive correlations between glasshouse germination percentage and seedling elongation.
Unlike other species, there has been no extensive study of variation in mesocotyl and coleoptile lengths in oats and how they correlate with emergence from depth. Publications on the mesocotyl length of oats were mainly published in the 1990s with limited numbers of genotypes, emphasising the impact of temperature on germination and coleoptile and mesocotyl lengths (Radford and Key 1993). Furthermore, no field validation has been conducted to ensure that deep-sown oats could emerge from depth.
This paper examines genetic differences in mesocotyl, coleoptile, and combined length in oats. Furthermore, the independence of mesocotyl coleoptile length in oats is examined. These components are compared across different temperatures to evaluate the impact of temperatures on mesocotyl, coleoptile, and combined length of oats. In addition, the establishment of oats from deep sowing is compared with other winter cereals (wheat and barley) under field conditions. The genetic diversity examined encompasses international, Australian, and unreleased oat genotypes. The hypotheses tested are: (1) oats have long combined (coleoptile and mesocotyl) length suitable for deep sowing; (2) temperatures exceeding 15°C will result in a reduced mesocotyl and coleoptile length; and (3) oats have a greater ability to emerge from depth compared to wheat and barley genotypes in field conditions, due to the mesocotyl and its longer combined length.
Materials and methods
Two controlled environment experiments and five field experiments were carried out to test these hypotheses.
Experiment 1: wide screening experiment
A wide screening experiment was conducted comparing the mesocotyl and coleoptile lengths of 195 diverse genotypes of oats (Avena sativa) (see Supplementary Table S1). The 195 genotypes were arranged using a completely randomised design with six replicates per genotype. The seeds were pre-screened to between 35 and 55 mg (husk not removed). Seeds were placed on the surface of 40 mm2 Jiffy pots (http://www.jiffypot.com) filled with course vermiculite and lightly covered with a thin layer of vermiculite (5 mm depth). These conditions were selected based on protocols from those previously developed for wheat (Triticum aestivum) (Rebetzke et al. 2007) and adapted for oats through a pilot experiment, which revealed that coleoptile and mesocotyl lengths were not significantly affected by sowing depth (5 mm or 150 mm) or substrate type (potting mix or vermiculite) when grown in darkness (Table S2). Jiffy pots were organised in a 112-cell tray, with a row spacing between each block to minimise entanglement. Trays were placed in lightproof black tubs and watered (about 3 L) so that water availability did not limit seedling growth. The tub lids were taped at the corners to keep light out, as even brief exposure to light impedes cell division in the mesocotyl, resulting in growth inhibition (Mer 1966). The experiment was conducted in a room at a constant temperature of 20 (±0.7) °C for 240°-days (°Cd; 12 days). The experiment’s duration was determined based on Radford and Key’s (1993) findings that 231.4°Cd was sufficient to complete elongation of both the mesocotyl and coleoptile. After 12 days, the seedlings were carefully removed from the vermiculite, and mesocotyl (oats), coleoptile (all cereals), and the combined length for each seedling was measured by extending them along a ruler. The mesocotyl-to-coleoptile length ratio (MCR) was calculated for the oat genotypes to analyse the relationship between the mesocotyl and coleoptile length:
The broad-sense heritability of the mesocotyl, coleoptile, and combined length was calculated based on Murphy et al.’s (2008) formula:
where is broad-sense heritability, is the mean sums of squares of the genetic component of variation, and is the mean sums of squares of the total variation in the population
In addition to analysing the 195 oat genotypes as a whole set, they were also categorised and analysed as three distinct groups: (1) 20 Australian commercial genotypes; (2) 71 international; and (3) 104 pre-breeding lines. Australian genotypes represent those that are (or were) commercially available in Australia. International genotypes include a wide genotype of oat genotypes sourced worldwide. Pre-breeding lines were unreleased genotypes that are still under selection by the InterGrain National oat breeding program.
Experiment 2: multiple temperature screening
This experiment evaluated the variation of mesocotyl and coleoptile lengths of 42 oat genotypes across four temperatures: (1) 15 ± 0.6°C; (2) 20 ± 0.7°C; (3) 25 ±0.7°C; and (4) 30 ± 0.9°C. The experiment was set up following the same method as the wide screening experiment (see above, Experiment 1). It included 42 genotypes with six replicates completely randomised within each temperature block. They were located in four constant-temperature rooms with each set to a different temperature and running simultaneously. The 42 oat genotypes were chosen to encompass all tested in the field validation experiment (Experiment 3), plus pre-breeding genotypes that offered genetic diversity from within the InterGrain breeding program and overseas genotypes found to have to have contrasting MCR in Experiment 1 (Table S1).
For consistency, at each temperature regime, mesocotyl and coleoptile length data were collected at 240°Cd, so the 15°C, 20°C, 25°C and 30°C treatments were assessed as above at 16, 12, 10, and 8 days, respectively.
Experiment 3: field experiments
Five field experiments were conducted comparing the emergence of 25 Australian cereal genotypes: 19 oats; four wheats; and two barleys. The field experiments were conducted at four different sites in Western Australia: (1) Merredin (31°28′54.9″S, 118°13′44.5″E); (2) Kunjin (32°17′03.0″S, 117°44′01.8″E); (3) Highbury (33°04′14.0″S, 117°15′46.9″E); and (4) Kurrenkutten (32°15′58.5″S, 118°00′09.7″E). Two experiments were carried out in 2023 at Merredin and Kunjin, followed by three more in 2024 at Merredin, Highbury, and Kurrenkutten analysed as a Multi-Environment Trials (MET) experiment.
Each of the field experiment used a randomised split plot design with two replicates of each genotype, with sowing depth (shallow at 40 mm and deep at 110 mm) as the main plots and genotypes as subplots. Seeds for the field experiments were obtained from InterGrain, an Australian cereal breeding company, sourced from the 2022 commercial seed purification increase, with seed weights ranging between 35 and 55 mg (husk not removed). Seed viability and quality were confirmed before the experiment, using standard germination tests.
The oat genotypes selected were those present in the 2023 season of the National Genotype Trials and relevant to Western Australia. Four yet-to-be-released oat genotypes were also included in the experiment. Wheat and barley genotypes were selected based on varying coleoptile lengths, year of release, and significance, to the Western Australian cereal cropping system. To contrast the semi-dwarfed wheat genotypes, the historical wheat genotype Halberd was also included. A full description of each genotype is in Table 1.
Genotype | Crop | Combined length A | Plant type | Plant height B | End use | Year of release | Seed weight (mg) | |
---|---|---|---|---|---|---|---|---|
Bannister | Oat | Extremely long | Dwarf | Tall | Dual purpose | 2017 | 34.4 | |
Koala | Oat | Extremely long | Dwarf | Medium-Tall | Dual purpose | 2023 | ||
Carrolup | Oat | Extremely long | Non-dwarf | Medium-Tall | Dual purpose | 1993 | 34.1 | |
Yallara | Oat | Extremely long | Non-dwarf | Medium-Tall | Dual purpose | 2008 | 36.5 | |
Goldie | Oat | Unknown | Unknown | Medium-Tall | Dual purpose | 2024 | 42.3 | |
Wandering | Oat | Extremely long | Dwarf | Tall | Grain | 2000 | 31.9 | |
Mitika | Oat | Extremely long | Dwarf | Short | Grain | 2005 | 39.5 | |
Kowari | Oat | Extremely long | Dwarf | Short | Grain | 2018 | 39.2 | |
Bilby | Oat | Extremely long | Dwarf | Tall | Grain | 2023 | 44.2 | |
Williams | Oat | Extremely long | Non-dwarf | Medium-Tall | Grain | 2017 | 34.3 | |
BL75 | Oat | Unknown | Unknown | Medium | Grain | – | 38.0 | |
BL76 | Oat | Unknown | Unknown | Tall | Grain | – | 37.2 | |
15175-56 | Oat | Unknown | Unknown | Short | Grain | – | 41.5 | |
15010-09 | Oat | Unknown | Unknown | Short | Grain | – | 42.5 | |
Wintaroo | Oat | Extremely long | Non-dwarf | Tall | Hay | 2001 | 38.6 | |
Kingbale | Oat | Extremely long | Non-dwarf | Tall | Hay | 2019 | 34.7 | |
Wallaby | Oat | Extremely long | Non-dwarf | Medium-Tall | Hay | 2023 | 37.5 | |
Kultarr | Oat | Extremely long | Non-dwarf | Tall | Hay | 2023 | 39.3 | |
Archer | Oat | Extremely long | Non-dwarf | Medium | Hay | 2022 | 38.6 | |
Vixen | Wheat | Medium | Dwarf | Medium | Grain | 2008 | 42.8 | |
DS Pascal | Wheat | Short | Dwarf | Medium | Grain | 2015 | 36.0 | |
Halberd | Wheat | Extremely long | Non-dwarf | Tall | Grain | 1969 | 47.1 | |
Ninja | Wheat | Medium | Dwarf | Medium | Noodle-wheat | 2020 | 52.2 | |
La Trobe | Barley | Medium | Dwarf | Medium | Grain | 2017 | 45.2 | |
Spartacus | Barley | Short | Dwarf | Medium | Grain | 2018 | 45.3 |
‘Combined length’ (coleoptile length for wheat and barley genotypes, total coleoptile and mesocotyl length for oats genotypes) and ‘plant type’ (dwarf or non-dwarf) data were obtained from the 2023 Western Australian Crop Sowing Guide (DPIRD 2023a). The ‘year of release’ and ‘plant height’ information was obtained from Australian Plant Breeder’s Rights (Australian Government 2023). The pre-breeding genotype information was obtained from InterGrain’s oat breeder.
Plots at all environments comprised seven rows spaced 0.25 m apart (total plot width of 1.5 m) and were either 12 m long at Merredin or 11 m long (Kunjin, Highbury, and Kurrenkutten). The number of seeds per plot for each genotype was adjusted by individual seed weight (Table 1) with a target plant density of 240 plants per m2. Trials were managed by local grower practices, with adequate fertiliser and standard herbicide application, and sprayed twice with fungicide to prevent foliar disease. Two replicate emergence counts of 0.5 m2 were randomly taken in each plot around 14 days after sowing (DAS), avoiding edge rows (Table 2).
Experiment | Sowing date | Soil type A | Rainfall zone (data from 2000–2018) B | Rainfall (mm) in April, May C | Max air temp (°C) in April, May C | |
---|---|---|---|---|---|---|
2023 | ||||||
Merredin | 5 May | Red shallow sandy loam duplex with clay subsoil | Low B | 31.2, 11.6 | 23.1, 21.9 | |
Kunjin | 18 Apr | Sandy duplex soil with a non-alkaline soil | Medium D | 81.9, 43.2 | 22.2, 21.4 | |
2024 | ||||||
Merredin | 30 Apr | Red shallow sandy loam duplex with clay subsoil | Low B | 0, 19.4 | 25.7, 25.1 | |
Kurrenkutten | 17 Apr E | Sandy duplex soil with a non-alkaline soil | Medium D | 0, 14.8 | 25.7, 24.4 | |
Highbury | 30 Apr | Loamy, sand duplex with a non-alkaline subsoil | Medium F | 0.4, 54.4 | 24.9, 22.5 |
Emergence counts were analysed as the ratio of emergence for deep-to-shallow sown seed:
This approach was used to eliminate the influence of variations in seed viability between genotypes. It uses emergence from shallow depth as the reference for emergence from depth. A higher deep-to-shallow emergence ratio indicates an increased ability of the genotypes to emerge from deep-sown (110 mm) compared to shallow-sown (40 mm) treatments.
Statistical analysis
The statistical analysis was conducted with the R program (R Core Team 2023).
In the first (wide screening) and second (temperature screening) experiment, the Shapiro–Wilk Test was used to check for data normality (Shapiro and Wilk 1965). Levene’s Test was used to ensure normality and error variance homogeneity between groups (Levene 1960). When the null hypothesis of equal group variances was rejected (P < 0.05), a heteroskedastic robust covariance matrix was implemented under the R statistical package ‘sandwich’ (Zeileis 2006). The distribution of the mesocotyl length, coleoptile length, combined length, and MCR in the wide screening experiment and temperature experiment (Experiments 1 and 2) were first examined with descriptive statistics from boxplot summaries.
ANOVA was then performed using the generalised linear model procedure included in the base R statistics package. The analysis examined the variation in mesocotyl length, coleoptile length, combined length, and MCR between genotypes within oats, within the three oat groups (Australian, international, and pre-breeding). Additionally, ANOVA was used to determine the effect of different temperatures on these traits. If ANOVA detected significance at P < 0.05, a pairwise post hoc test (Tukey’s ‘Honestly Significant Difference’; HSD) was implemented to test for significant differences between means. The effect of blocks was also evaluated in the ANOVA.
The third (field) experiment was analysed as a Multi-Environment Trial (MET) experiment. The variation in emergence ratio between genotypes and cereal crop species (wheat, barley, and oats) was analysed using Levene’s test, ANOVA, and pairwise post hoc test using the same methods outlined above.
Furthermore, the linear regression model, implemented under the generalised linear model procedure in the base R statistics package, was used to estimate the relationship between temperature and emergence ratio with combined length and to analyse the correlations between mesocotyl and coleoptile length.
Results
Experiment 1: wide screening experiment
There were significant differences in combined length, mesocotyl length, coleoptile length, and MCR between the oat genotypes (P < 0.001; Table S3). Across the 195 oat genotypes, combined lengths ranged from 112 mm to 219 mm, with a mean of 169 (±2) mm (Fig. 1). The mesocotyl length was more variable and shorter than the coleoptile length, with a mean of 73 (±1) mm compared to 96 (±1) mm, respectively. The mesocotyl lengths ranged from 32 mm to 119 mm, while the coleoptile lengths ranged from 21 mm to 71 mm. Furthermore, the mesocotyl-to-coleoptile length ratio (MCR) ranged from 0.31 to 1.92, with a mean of 0.8 (±0.03). Notably, 75% of the data (3rd quartile) exhibited MCR of 0.9 (Fig. 1). Mesocotyl length and coleoptile length were not correlated (R2 = 0.004).
Boxplot of total combined length (TL), mesocotyl length (ML), coleoptile length (CL), and mesocotyl-to-coleoptile ratio (MCR) of the 195 oat genotypes in the wide screening experiment, where seedlings were grown at 20°C for 240°Cd. The boxplot indicates the median (middle pinch line), interquartile range (box length – lower quartile represent the 25th percentile and the upper quartile represent the 75th percentile), and overall spread of the data (whisker – smallest and largest sample value), along with any potential outliers (indicated by black dots). Each variety means was also represented in the coloured dots.
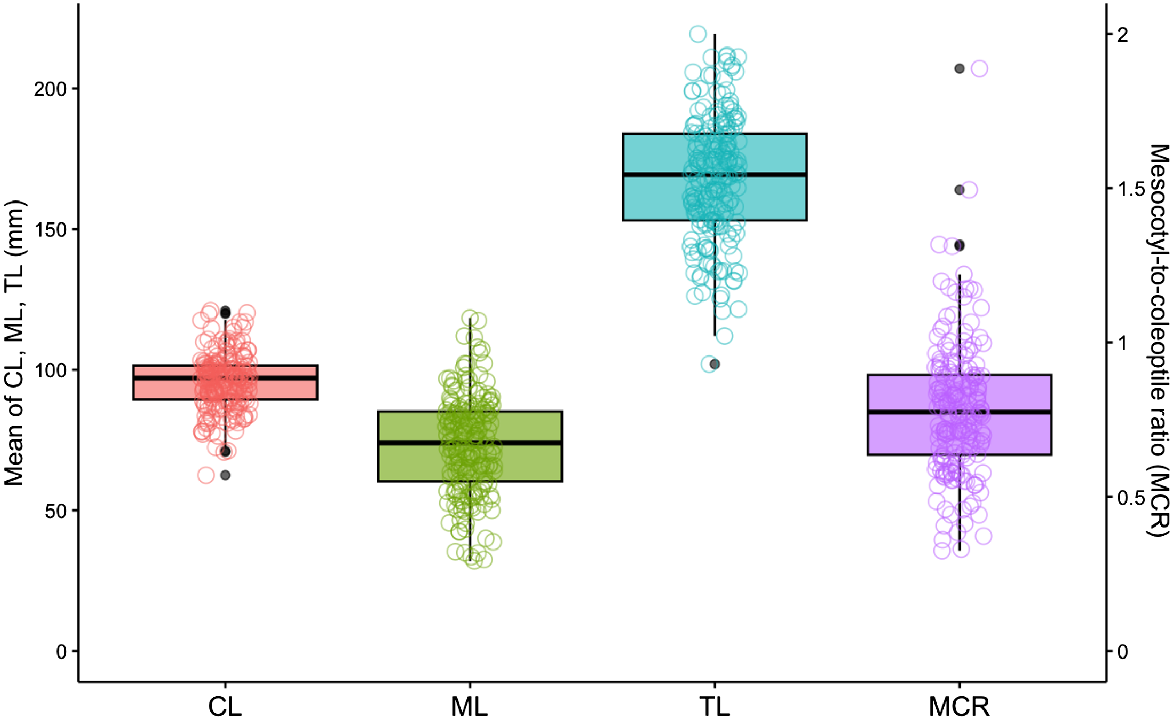
The combined length and mesocotyl length of the 195 oat genotypes were highly heritable with of 0.81 (±0.10) and 0.85 (±0.10), respectively. This suggests that the majority of the observed (phenotypic variance) variances in oat genotypes’ combined length and mesocotyl length were attributable to genetic factors. In contrast, the coleoptile length was less heritable, with a value of 0.54 (±0.10), indicating that roughly half of the observed differences in coleoptile length can be attributed to genetic factors.
There were significant differences in combined length, mesocotyl length, and coleoptile length between the oat groups: Australian commercial; international; and pre-breeding genotypes (P < 0.001; Table S4). No significant differences were seen in the MCR (P = 0.40; Table S4). However, post hoc tests revealed no significant difference between the pre-breeding and Australian genotypes in the mesocotyl, coleoptile, or combined lengths (P = 0.07; P = 0.96; P = 0.99). International genotypes have greater combined lengths, measuring 20 mm more than Australian commercial genotypes and 15 mm more than pre-breeding genotypes (Table S4). They also have longer coleoptile and mesocotyl lengths (Fig. 2).
Four boxplots of coleoptile length (mm), mesocotyl length (mm), mesocotyl-to-coleoptile length ratio (MCR), and combined (mesocotyl and coleoptile) length (mm) of 195 oat genotypes through different oat categories (Australian, international, pre-breeding). The boxplot indicates the median (middle line), interquartile range (box length – lower quartile represent the 25th percentile and the upper quartile represent the 75th percentile), and overall spread of the data (whisker – smallest and largest sample value), along with any potential outliers (indicated by dots).
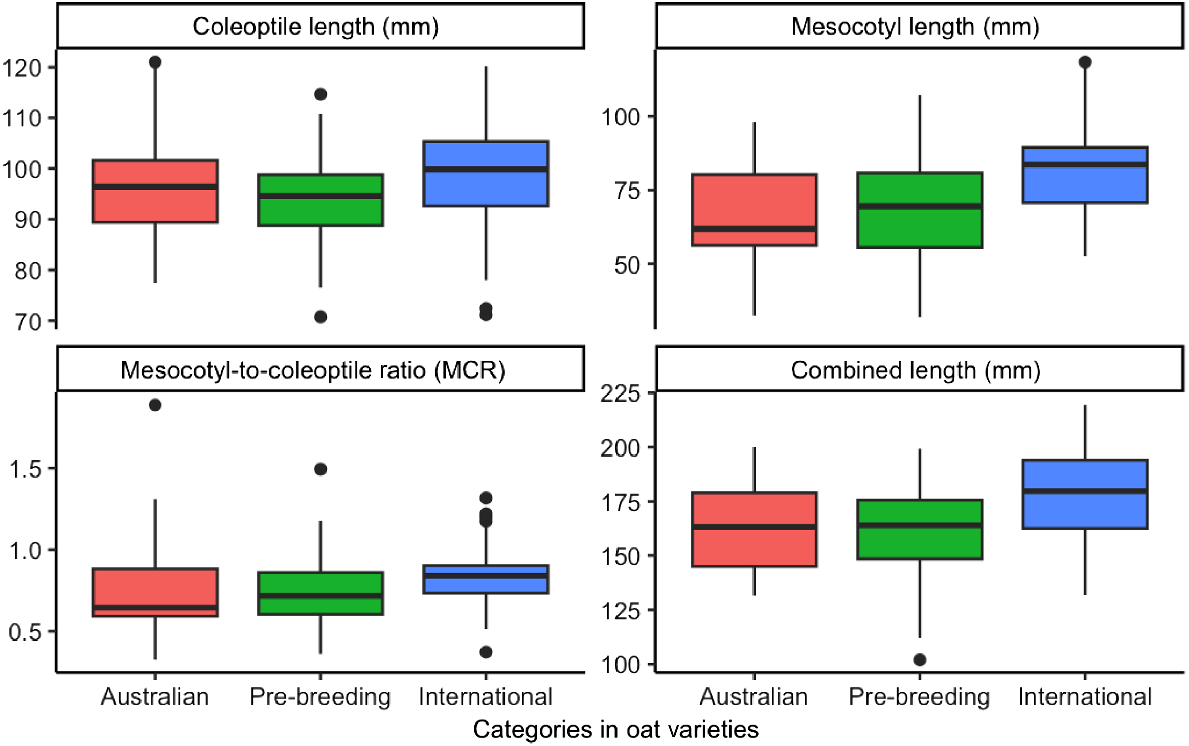
Experiment 2: temperature response
There was a strong response to temperature with increasing temperature from 15 to 30°C significantly decreased the combined length, mesocotyl length, and coleoptile length of 42 oat genotypes (P < 0.001; Fig. 3; Table S5). The mean combined length decreased from 185 (±4) mm, 165 (±4) mm, 132 (±3) mm, to 103 (±3) at 15°C, 20°C, 25°C, and 30°C, respectively. There was a significant interaction between genotype and temperature to combined length (P < 0.001), indicating that some genotypes were more sensitive to temperature than others; however, a consistent reduction in combined length with increasing temperature was observed across all the oat genotypes. The MCR increased with temperature, but this was only significant at 30°C (P < 0.001; Table S5), suggesting that coleoptile length decreased to a greater extent than mesocotyl length in higher temperatures. At 25°C and 30°C, the minimum combined length was 89 mm and 68 mm, respectively. Coleoptile and mesocotyl lengths were reduced by 3.67 mm and 1.05 mm per °C increase in temperature (Fig. 3).
Four boxplots of total combined length (mm), coleoptile length (mm), mesocotyl length (mm), and mesocotyl-to-coleoptile length ratio (MCR) of 42 oat genotypes through different temperatures (15°C, 20°C, 25°C, 30°C). The boxplot indicates the median (middle line), interquartile range (box length – lower quartile represent the 25th percentile and the upper quartile represent the 75th percentile), and overall spread of the data (whisker – smallest and largest sample value), along with any potential outliers (indicated by dots).
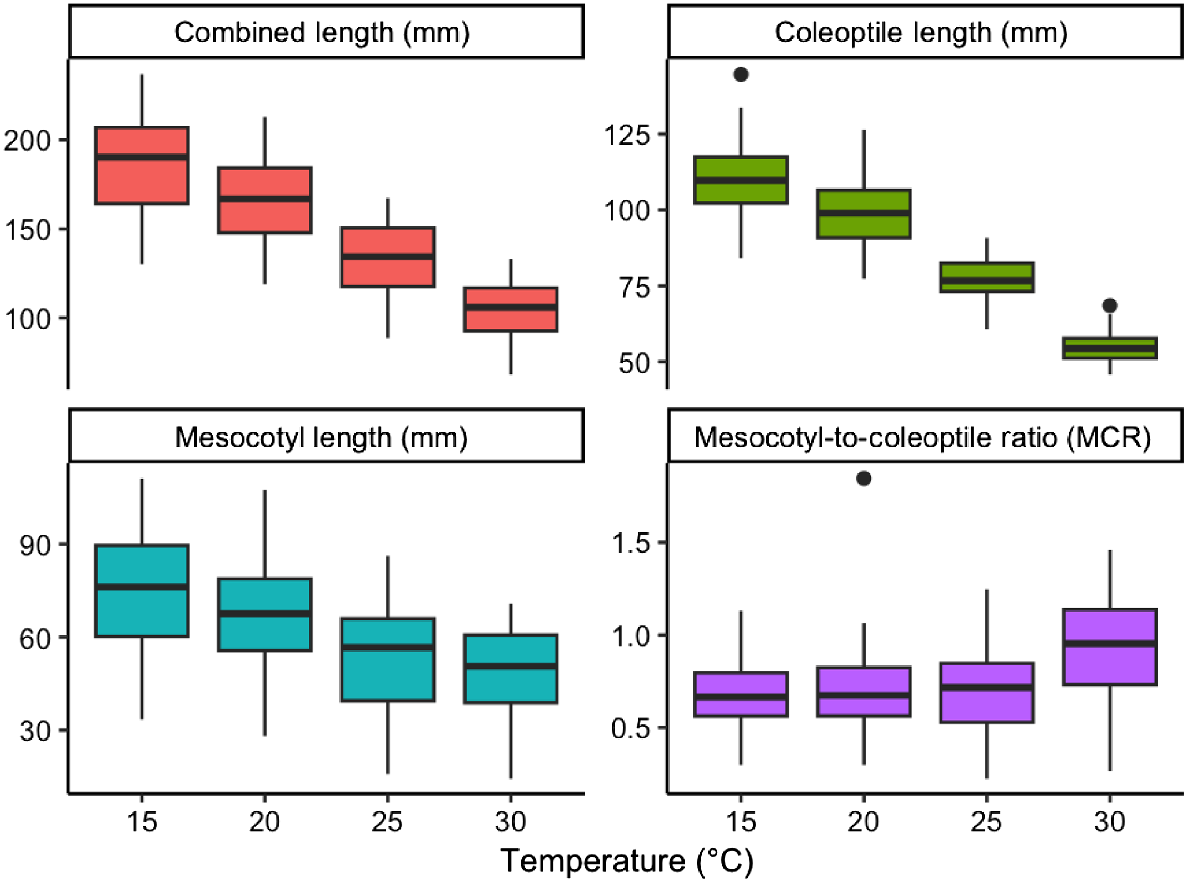
Field experiments
There were no significant differences between emergence ratio at an environment (P = 0.18), replicate (P = 0.88), or experiment and genotype interaction (P = 0.58) levels. There were significant differences in emergence ratio between crop species (Fig. 4; P < 0.001). Oats had a higher average emergence ratio (0.79 (±0.01)) than wheat and barley genotypes (0.56 (±0.03) and 0.51 (±0.09), respectively). However, there were no significant difference in emergence ratio between wheat and barley genotypes (P = 0.97). Most oat genotypes have a higher emergence ratio value than the wheat and barley genotypes, except for Halberd, the older non-dwarfed wheat genotype with a longer coleoptile (Fig. 4). There was no significant variation in emergence ratio across the oat genotypes (P = 0.76).
Deep-to-shallow emergence ratio of the oat, wheat, and barley genotypes 14 days after sowing (DAS) at the five environments: Merredin and Kunjin in 2023; Merredin, Kurrenkutten, and Highbury in 2024. Seedlings are deep-sown at 110 mm and shallow-sown at 40 mm. Bars are mean ± s.e., n = 4.
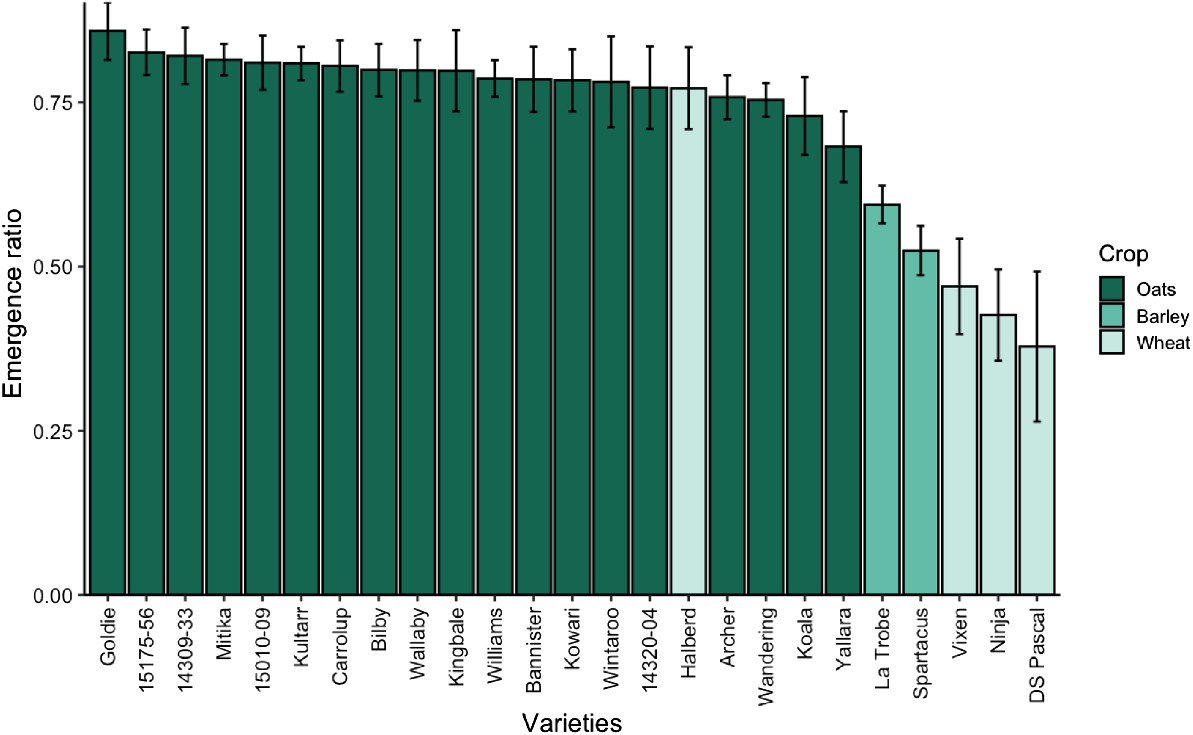
The mesocotyl, coleoptile, and combined length data for the genotypes included in the field trials were extracted from the wide-screen results (see Fig. 5) and showed significant differences in combined lengths (Fig. 5; P < 0.001). Compared to oat genotypes, barley and wheat genotypes exhibited lower combined length differences by 89 mm and 57 mm, respectively. All the oat genotypes displayed longer combined lengths compared to the wheat and barley genotypes, except for Halberd. Halberd, which is the older, non-dwarf wheat genotype, exhibited the longest coleoptile length at 162 (±14) mm, while other wheat and barley genotypes in the trial ranged from 58 mm to 82 mm in combined lengths (i.e. medium length class).
Combined (mesocotyl and coleoptile) length (mm) of oat, wheat, and barley genotypes included in the field experiments. Coleoptile and mesocotyl lengths were measured on seedlings grown in the dark for 20°C for 240°Cd. Oat genotypes have two stacked bars, with mesocotyl length indicated in the lower bar. Bars are mean ± s.e., n = 6.
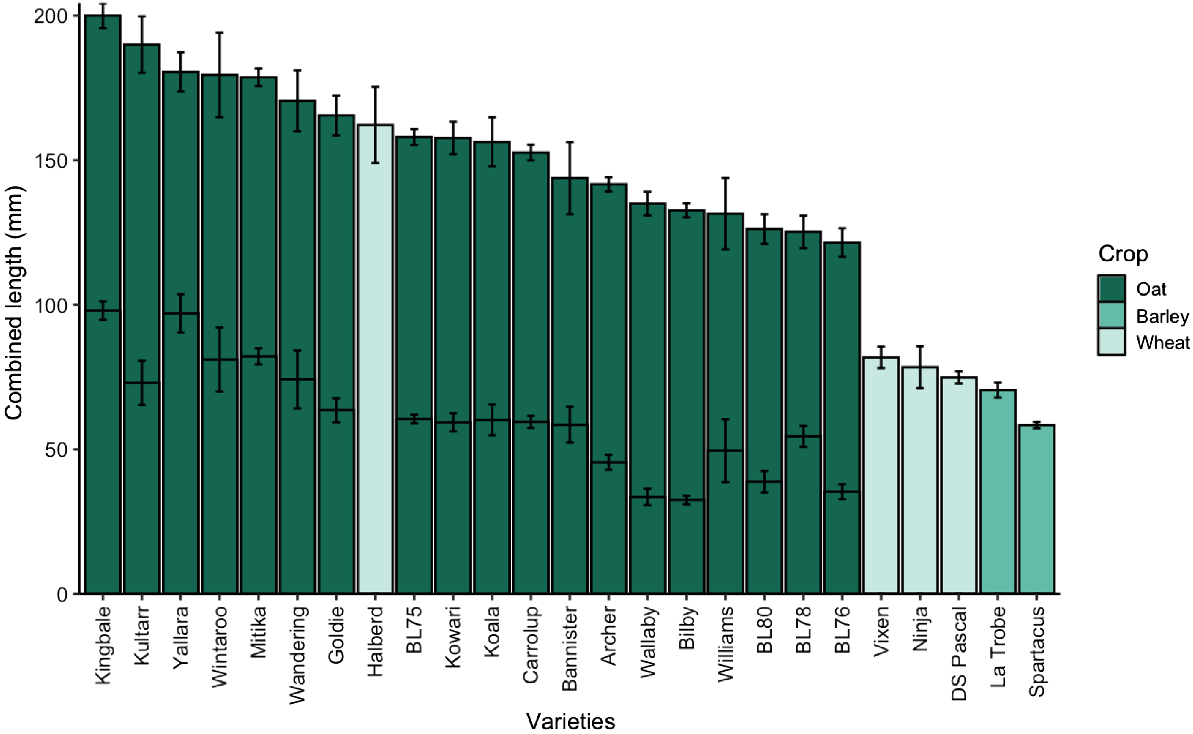
Furthermore, there were significant differences between emergence ratio and genotype (P < 0.001), meaning that observations were consistent across all environments and thus could be analysed as replicates. The type of crop (wheat, barley, or oat) was found to have a significant effect on emergence ratio (P < 0.001). Any genotype with combined lengths greater than 110 mm (deep sowing depth in this study) had a similar emergence ratio, with no significant correlation (R2 = 0.01; P = 0.57). This group included all the oat genotypes and the older wheat genotype Halberd. However, the second group, with combined lengths less than 110 mm, did not emerge well from depth, with low emergence ratio values (Fig. 6).
Discussion
This research tested three key hypotheses related to coleoptile and mesocotyl length in oats. The first hypothesis was that all oats have a long total length (combined mesocotyl and coleoptile length), suited for deep sowing. The results supported this hypothesis with the widescreen of 195 oat genotypes all having a combined length greater than 112 mm, with large variations between genotypes ranging from 112 mm to 219 mm (Fig. 1). There were also differences between the groups of oat genotypes, with international genotypes tending to have longer combined lengths than commercial Australian and pre-breeding lines (Fig. 2). These results support the findings of Radford and Key (1993) and DPIRD (2023a) on smaller sets of genotypes. Zhou et al. (2024) measured the coleoptile length of 243 oat varieties; they showed a wide range in coleoptile lengths which is consistent with our results. However, they reported a mean coleoptile length approximately 30 mm shorter than our results. This difference may be attributed to their use of opaque bags, which despite limiting light exposure, may still allow some light to pass through, potentially impacting coleoptile length. Yan et al. (2021) compared the coleoptile length of a tall and dwarf oat variety. They found a coleoptile length of approximately 110 mm. They also found that coleoptile length was approximately 15% longer in the tall variety. Surprisingly, neither Zhou et al. (2024) or Yan et al. (2021) measured mesocotyl length in their studies. Turner et al. (1982) and Radford and Key (1993) established that combined lengths are reliable indicators of potential sowing depth.
Genetic differences in both coleoptile and mesocotyl length have also been identified in tropical crops like rice (e.g. Li et al. 2024) and maize (e.g. Yang et al. 2024). Other winter crop species such as wheat show wide genetic variation in coleoptile length and that these have been associated with a range of different genes (Mohan et al. 2013; Rebetzke et al. 2014; Bovill et al. 2019). Differences in wheat coleoptile length have often been associated with different dwarfing genes (Rebetzke et al. 2012, 2022a).
The second hypothesis was that temperatures exceeding 20°C would result in reduced mesocotyl and coleoptile length of oat. The results supported this hypothesis, with the coleoptile length reduced by 3.67 mm and mesocotyl length by 1.05 mm per 1°C increase in temperature. These results were consistent across oat genotypes (Fig. 3). Radford and Key (1993) had comparable results, determining that 15°C was the best temperature for maximum combined length, with a subsequent decrease in length at temperatures over 20°C. Similarly, Rebetzke et al. (2004) showed that coleoptile length of wheat was reduced at temperatures greater than 19°C. Additionally, while the average lengths of both coleoptile and mesocotyl lengths decreased uniformly with rising temperatures, we found a significant shift in the MCR at 30°C. At this temperature, the rate of decrease in coleoptile length exceeded that of the mesocotyl, indicating that temperatures above 25°C have a bigger influence on reducing coleoptile length than the mesocotyl. Notably, most oat genotypes exhibited longer coleoptile lengths than mesocotyl lengths, with no apparent correlation between these two traits. Together with the high heritability value for mesocotyl length, this indicates that there is a significant opportunity to increase mesocotyl length in oats to further improve the ability of oats to emerge from deep sowing.
The third hypothesis was that the mesocotyl in oats would allow it to emerge from depth more effectively than wheat and barley, due to its increased combined length. The results supported this hypothesis, showing that across oat genotypes deep sowing had an average reduction in plant number of 21% when sown at a depth of 110 mm compared to 40 mm. In contrast, wheat and barley genotypes exhibited average reductions of 44% and 49%, respectively (Fig. 4). Longer combined lengths improve the emergence of oat, wheat, and barley genotypes from deeper depths. However, to achieve high emergence levels (>75%, Figs 4 and 6), the combined length only needs to exceed the sowing depth (110 mm in this study). Indeed, amongst the oat genotypes tested and Halberd, there was no association between total length and emergence ratio. Thus, given that all oat genotypes have combined lengths greater than 110 mm, it is questionable whether genetic selection for mesocotyl length is required unless sowing deeper than 110 mm is to be considered or soil temperatures increase substantially. In contrast, wheat genotypes containing a dwarfing gene (Rht-B1b or Rht-D1b) and barley genotypes all had coleoptile lengths shorter than 110 mm and no mesocotyl. Therefore, selection for increased coleoptile length in these crops is warranted for deeper sowing (e.g. Rebetzke et al. 2022b). Wheat and barley genotypes with combined lengths (only coleoptile length with no mesocotyl) below 110 mm experienced reduced emergence rates, indicating their inability to reach the sowing depth during emergence. These results are consistent with the results of Rebetzke et al. (2007), Stummer et al. (2023) and Mohan et al. (2013), which showed that from deep sowing increased coleoptile length in wheat can improve establishment. Our results extend this analysis and show that for oats, the existence of the mesocotyl extends the coleoptile and the ability to emerge from depth in oats.
Implications of oats in early and deep-sown conditions
Early sowing of winter cereals offers numerous advantages, including deeper root penetration, increased weed competition, greater biomass accumulation, higher water use efficiency (WUE), and reduced exposure to heat as it enables crops to flower early (Passioura and Angus 2010; Kirkegaard et al. 2015; Rasmussen and Thorup-Kristensen 2016). Early sowing could expose the seedlings to maximum soil temperatures above 30°C in the Western Australian wheatbelt during the sowing window (Sudmeyer et al. 2016). Climate change compounds this issue, as autumn weather is predicted to become hotter, drier and more variable. Some climate models indicate that by 2060, sowing season air temperatures could rise by as much as 4°C (Whetton et al. 2015; Frederiksen and Osbrough 2022). Our findings revealed that at 25°C and 30°C, the minimum mean combined length of the oat genotypes were 89 mm and 68 mm, respectively. This suggests that when sowing early with high soil temperatures, the combined length of the shortest genotypes may be below the 110 mm threshold and therefore may have limited emergence from deep sowing. Radford and Key (1993) found that higher temperatures caused temporary dormancy, resulting in further poor field emergence of oats at high soil temperatures, causing germination delays and insufficient seedling length. This highlights the need for a genetic solution, encouraging breeders to prioritise the selection of oat genotypes with longer lengths adapted for hotter temperatures.
The field results showed no relationship between emergence from depth and oat genotypes’ combined lengths, as soil temperatures were not extreme and seeding depth did not exceed 110 mm, which is shorter than the total combined length of all oats genotypes tested. It is likely that in higher-strength soils, combined with warmer soil temperatures, having the genetic potential for a longer combined length would be beneficial to increase emergence ability. The possibility for obtaining such genetic traits from international oat genotypes is promising. International oat genotypes had combined mesocotyl and coleoptile lengths that were 20 mm greater than Australian genotypes and 15 mm greater than unreleased genotypes. This indicates that international genotypes might include useful genetics for increased combined length. Furthermore, breeders should target increased mesocotyl, as it exhibits higher heritability and greater genetic variation compared to coleoptile length and is also less affected by higher temperatures. Specifically, mesocotyl length was three times more genetically variable than coleoptile length, emphasising its potential as a key trait in breeding programs aimed at enhancing seedling emergence and early vigour. Fortunately, there was no correlation between mesocotyl and coleoptile lengths which indicates they are under separate genetic control. This variation presents a valuable opportunity for further research to identify and associate these traits with genetic markers or quantitative trait loci (QTLs) for deployment of simultaneous, multi-trait genomic selection (e.g. Meuwissen et al. 2001; Azizinia et al. 2023). By deploying appropriate genomic tools, breeders could develop more resilient oat genotypes with optimised mesocotyl and coleoptile lengths. Potential future studies could focus on mapping these traits to specific genetic regions, exploring the underlying genetic mechanisms, and evaluating the performance of selected genotypes in different environmental conditions.
Sowing early could expose winter cereals to frost during flowering, unless genotype phenology is matched so flowering time balances the risks of frost and heat stress and maximises the period for growth (Flohr et al. 2017; Cann et al. 2023). The increased risk of frost when flowering early is a relative advantage for oats. Oats demonstrate greater frost tolerance compared to wheat or barley. Oats tend to be more tolerant to frost than wheat and barley (DPIRD 2023b) and therefore, oats are a lower-risk option for farmers managing early sowing or frost-prone paddocks. This frost tolerance enhances their suitability for early sowing systems. Furthermore, the adoption of dual-purpose oats (genotype that can be both a grain and/or hay) offers further benefits, including increased profits, enhanced management flexibility, and improved risk mitigation. The early-sown dual-purpose oats give farmers flexible exit options, including grazing, haymaking, silage production, or grain harvest. This flexibility minimises risk exposure and maximises profit across diverse economic scenarios (Kirkegaard et al. 2019).
Limitations and future directions
The field experiment included 19 oat genotypes, four wheat genotypes, and two barley genotypes, which could introduce potential biases when comparing emergence. The small number of wheat and barley genotypes do not represent the full range of coleoptile lengths in these species. Nonetheless, the coleoptile lengths we observed were representative of DPIRD’s (2023a) assessments of modern wheat and barley genotypes. Wheat emergence presented correlated with the findings of Rebetzke et al. (2007). Although there is currently a lack of field data on emergence and biomass for deep-sown barley genotypes. Future research should aim to collect data on oats’ suitability to be deep-sown across a broader range of soil types in locations spanning different rainfall and climatic zones. Research investigating the impact of deep-sown oats on biomass and yield should also be done. Here, only data on emergence have been presented. Recent research by Zhao et al. (2023) and Niu et al. (2020) indicates that mesocotyl growth is more sensitive to chemical and environmental changes compared to the coleoptile during early growth stages. This heightened sensitivity suggests that the mesocotyl is more responsive to pre-emergent environmental conditions. Further research is required to identify oat genotypes better adapted to variable pre-emergence conditions.
Conclusions
With changing rainfall patterns and soil temperature at seeding across the Australian grain-growing regions, there is a pressing need for research into climate-resilient cereal genotypes. This research has highlighted the opportunity for deep-sown oats to mitigate the climate risks associated with crop establishment. Regardless of genotype, all oat varieties tested were able to successfully emerge from deep sowing. However, within the diverse range of oat genotypes screened, there was significant genetic diversity in mesocotyl and coleoptile length. This paper has highlighted the potential for oats with longer combined length, enabling deeper sowing, to be a factor in improving the climate-resilience of oats. By selecting genotypes with a longer combined length, suited for higher temperature zones, breeders could facilitate deeper sowing of oats. This would help farmers to achieve timely sowing, mitigate poor field emergence, and maximize yields.
Data availability
The data that support this study will be shared upon reasonable request to the corresponding author.
Declaration of funding
This research investment was funded by the Processed Oat Partnership (POP), an industry-led program supported by a A$10.12 m State Government investment. The POP aims to increase the WA production of processed oats, hectares sown, and support industry to capture a greater share of growing international demand for oat products.
Acknowledgements
The authors thank Shayne Micin at CSIRO Floreat, Perth for providing technical assistance during this research. We also thank InterGrain, the Grains Research and Development Corporation and The South Australian Research and Development Institute for provision of germplasm and facilities to undertake this research.
References
Australian Government (2023) Plant breeder’s rights search - IP Australia. Available at https://ipsearch.ipaustralia.gov.au/pbr/
Azizinia S, Mullan D, Rattey A, Godoy J, Robinson H, Moody D, Forrest K, Keeble-Gagnere G, Hayden MJ, Tibbits JFG, Daetwyler HD (2023) Improved multi-trait prediction of wheat end-product quality traits by integrating NIR-predicted phenotypes. Frontiers in Plant Science 14, 1167221.
| Crossref | Google Scholar |
BOM (2024) Climate data online. Available at http://www.bom.gov.au/climate/data/index.shtml
Bovill WD, Hyles J, Zwart AB, Ford BA, Perera G, Phongkham T, Brooks BJ, Rebetzke GJ, Hayden MJ, Hunt JR, Spielmeyer W (2019) Increase in coleoptile length and establishment by Lcol-A1, a genetic locus with major effect in wheat. BMC Plant Biology 19, 332.
| Crossref | Google Scholar |
Cann DJ, Hunt JR, Porker KD, Harris FAJ, Rattey A, Hyles J (2023) The role of phenology in environmental adaptation of winter wheat. European Journal of Agronomy 143, 126686.
| Crossref | Google Scholar |
Dey R, Lewis SC, Arblaster JM, Abram NJ (2019) A review of past and projected changes in Australia’s rainfall. WIREs Climate Change 10(3), e577.
| Crossref | Google Scholar |
DPIRD (2021a) Climate change in the Merredin area, Western Australia. Available at https://www.agric.wa.gov.au/climate-change/climate-change-merredin-area-western-australia
DPIRD (2021b) Climate change in the Corrigin area, Western Australia. Available at https://www.agric.wa.gov.au/climate-change/climate-change-corrigin-area-western-australia
DPIRD (2022) Climate change in the Corrigin area, Western Australia. Available at https://www.agric.wa.gov.au/climate-change/climate-change-corrigin-area-western-australia
DPIRD (2023a) 2023 Western Australian crop sowing guide. Available at https://www.agric.wa.gov.au/sites/gateway/files/2023%20WA%20Crop%20Sowing%20Guide_3.pdf
DPIRD (2023b) Managing frost risk. Available at https://www.agric.wa.gov.au/frost/managing-frost-risk?page=0%2C1
Ellis M, Spielmeyer W, Gale K, Rebetzke G, Richards R (2002) “Perfect” markers for the Rht-B1b and Rht-D1b dwarfing genes in wheat. Theoretical and Applied Genetics 105, 1038-1042.
| Crossref | Google Scholar | PubMed |
Fletcher A, Lawes R, Weeks C (2016) Crop area increases drive earlier and dry sowing in Western Australia: implications for farming systems. Crop & Pasture Science 67(12), 1268-1280.
| Crossref | Google Scholar |
Flohr BM, Hunt JR, Kirkegaard JA, Evans JR (2017) Water and temperature stress define the optimal flowering period for wheat in south-eastern Australia. Field Crops Research 209, 108-119.
| Crossref | Google Scholar |
Flohr BM, Ouzman J, McBeath TM, Rebetzke GJ, Kirkegaard JA, Llewellyn RS (2021) Redefining the link between rainfall and crop establishment in dryland cropping systems. Agricultural Systems 190, 103105.
| Crossref | Google Scholar |
Frederiksen JS, Osbrough SL (2022) Tipping points and changes in Australian climate and extremes. Climate 10(5), 73.
| Crossref | Google Scholar |
Hoshikawa K (1969) Underground organs of the seedlings and the systematics of Gramineae. Botanical Gazette 130(3), 192-203.
| Crossref | Google Scholar |
Hunt JR, Lilley JM, Trevaskis B, Flohr BM, Peake A, Fletcher A, Zwart AB, Gobbett D, Kirkegaard JA (2019) Early sowing systems can boost Australian wheat yields despite recent climate change. Nature Climate Change 9(3), 244-247.
| Crossref | Google Scholar |
Kirkegaard JA, Lilley JM, Hunt JR, Sprague SJ, Ytting NK, Rasmussen IS, Graham JM (2015) Effect of defoliation by grazing or shoot removal on the root growth of field-grown wheat (Triticum aestivum L.). Crop & Pasture Science 66(4), 249-259.
| Crossref | Google Scholar |
Kirkegaard J, Sprague S, Lilley J, Bell L, Swan T (2019) Maximising systems benefits from dual-purpose crops – early sowing and grazing strategies. Available at https://grdc.com.au/resources-and-publications/grdc-update-papers/tab-content/grdc-update-papers/2019/02/maximising-systems-benefits-from-dual-purpose-crops-early-sowing-and-grazing-strategies
Li R, Wei Z, Wang Y, Ma M, Zhang Q, Bai T, Li Z, Zhang Z, Yin H, Wang Y (2024) Association mapping of mesocotyl and coleoptile length in rice using various genome-wide association study models. Crop Science 64(6), 3417-3429.
| Crossref | Google Scholar |
Mer CL (1966) The inhibition of cell division in the mesocotyl of etiolated oat plants by light of different frequencies. Annals of Botany 30(1), 17-23.
| Crossref | Google Scholar |
Meuwissen THE, Hayes BJ, Goddard ME (2001) Prediction of total genetic value using genome-wide dense marker maps. Genetics 157(4), 1819-1829.
| Crossref | Google Scholar | PubMed |
Mgonja MA, Ladeinde TAO, Aken’Ova ME (1993) Genetic analysis of mesocotyl length and its relationship with other agronomic characters in rice (Oryza sativa L.). Euphytica 72, 189-195.
| Crossref | Google Scholar |
Mohan A, Schilinger WF, Gill KS (2013) Wheat seedling emergence from deep planting depths and its relationship with coleoptile length. PLoS ONE 8(9), e73314.
| Crossref | Google Scholar | PubMed |
Murphy K, Balow K, Lyon SR, Jones SS (2008) Response to selection, combining ability and heritability of coleoptile length in winter wheat. Euphytica 164, 709-718.
| Crossref | Google Scholar |
Niu L, Hao R, Wu X, Wang W (2020) Maize mesocotyl: role in response to stress and deep-sowing tolerance. Plant Breeding 139(3), 466-473.
| Crossref | Google Scholar |
Ohno H, Banayo NPMC, Bueno CS, Kashiwagi J-I, Nakashima T, Corales AM, Garcia R, Sandhu N, Kumar A, Kato Y (2018) Longer mesocotyl contributes to quick seedling establishment, improved root anchorage, and early vigor of deep-sown rice. Field Crops Research 228, 84-92.
| Crossref | Google Scholar |
Pook M, Lisson S, Risbey J, Ummenhofer CC, McIntosh P, Rebbeck M (2009) The autumn break for cropping in southeast Australia: trends, synoptic influences and impacts on wheat yield. International Journal of Climatology 29(13), 2012-2026.
| Crossref | Google Scholar |
R Core Team (2023) R: a language and environment for statistical computing. Available at https://www.R-project.org/
Radford BJ (1987) Effect of constant and fluctuating temperature regimes and seed source on the coleoptile length of tall and semidwarf wheats. Australian Journal of Experimental Agriculture 27(1), 113-117.
| Crossref | Google Scholar |
Radford BJ, Key AJ (1993) Temperature affects germination, mesocotyl length and coleoptile length of oats genotypes. Australian Journal of Agricultural Research 44(4), 677-688.
| Crossref | Google Scholar |
Rasmussen IS, Thorup-Kristensen K (2016) Does earlier sowing of winter wheat improve root growth and N uptake? Field Crops Research 196, 10-21.
| Crossref | Google Scholar |
Rebetzke GJ, Richards RA, Fischer VM, Mickelson BJ (1999) Breeding long coleoptile, reduced height wheats. Euphytica 106, 159-168.
| Crossref | Google Scholar |
Rebetzke GJ, Richards RA, Sirault XRR, Morrison AD (2004) Genetic analysis of coleoptile length and diameter in wheat. Australian Journal of Agricultural Research 55(7), 733-743.
| Crossref | Google Scholar |
Rebetzke GJ, Richards RA, Fettell NA, Long M, Condon AG, Forrester RI, Botwright TL (2007) Genotypic increases in coleoptile length improves stand establishment, vigour and grain yield of deep-sown wheat. Field Crops Research 100(1), 10-23.
| Crossref | Google Scholar |
Rebetzke GJ, Bonnett DG, Ellis MH (2012) Combining gibberellic acid-sensitive and insensitive dwarfing genes in breeding of higher-yielding, sesqui-dwarf wheats. Field Crops Research 127, 17-25.
| Crossref | Google Scholar |
Rebetzke GJ, Verbyla AP, Verbyla KL, Morell MK, Cavanagh CR (2014) Use of a large multiparent wheat mapping population in genomic dissection of coleoptile and seedling growth. Plant Biotechnology Journal 12(2), 219-230.
| Crossref | Google Scholar | PubMed |
Rebetzke GJ, Rattey AR, Bovill WD, Richards RA, Brooks BJ, Ellis M (2022a) Agronomic assessment of the durum Rht18 dwarfing gene in bread wheat. Crop & Pasture Science 73, 325-336.
| Crossref | Google Scholar |
Rebetzke G, Kirkegaard J, McBeath T, Stummer B, Flohr B, Fletcher A, Rich S, Lamond M, Haskins B, Whitworth R, Bechaz K, Gullifer L, Aisthrope D, Ware A, Meiklejohn R (2022b) Early learnings from multi-site, multi-system assessment of new long-coleoptile genetics for deep sowing of wheat. Available at https://grdc.com.au/resources-and-publications/grdc-update-papers/tab-content/grdc-update-papers/2022/02/early-learnings-from-multi-site,-multi-system-assessment-of-new-long-coleoptile-genetics-for-deep-sowing-of-wheat#:~:text=Conclusions,sowing%20for%20maximising%20water%20productivity
Rich S, Oliver Y, Richetti J, Lawes R (2022) Chasing water: increasing sowing opportunity with small changes to sowing depth across the low and medium rainfall zones of Western Australia. Available at https://www.agronomyaustraliaproceedings.org/images/sampledata/2022/Climate/Agronomy-for-managing-water/ASArich_s_507s.pdf
Richards RA (1992) The effect of dwarfing genes in spring wheat in dry environments. II. Growth, water use and water-use efficiency. Australian Journal of Agricultural Research 43(3), 529-539.
| Crossref | Google Scholar |
Schoknecht NR, Pathan S (2013) Soil groups of Western Australia: a simple guide to the main soils of Western Australia. 4th edn. Department of Agriculture and Food. Available at https://library.dpird.wa.gov.au/rmtr/348.
Shapiro SS, Wilk MB (1965) An analysis of variance test for normality (complete samples). Biometrika 52(3–4), 591-611.
| Crossref | Google Scholar |
Stummer BE, Flohr BM, Rebetzke GJ, Meiklejohn R, Ware A, Haskins B, Whitworth R, McBeath T (2023) Long coleoptile genotype and soil texture interactions determine establishment success and early growth parameters of wheat sown at depth. Environmental Research Communications 5(5), 055015.
| Crossref | Google Scholar |
Turner FT, Chen CC, Bollich CN (1982) Coleoptile and mesocotyl lengths in semidwarf rice seedlings. Crop Science 22(1), 43-46.
| Crossref | Google Scholar |
Whetton P, Holper P, Clarke J, Leanne W, Hennessy K, Colman R, Moise A, Power S, Braganza K, Watterson I, Murphy B, Timbal B, Hope P, Dowdy A, Bhend J, Kirono D, Wilson L, Grose M, Ekstrom M, Rafter T, Heady C, Narsey S, Bathols J, McInnes K, Monselesan D, Church J, Lenton A, O’Grady J, Bedin T, Erwin T, Li Y (2015) ‘Climate change in Australia. Projections for Australia’s NRM regions.’ (CSIRO and Bureau of Meteorology: Melbourne)
Yan H, Yu K, Xu Y, Zhou P, Zhao J, Li Y, Liu X, Ren C, Peng Y (2021) Position validation of the dwarfing gene Dw6 in oat (Avena sativa L.) and its correlated effects on agronomic traits. Frontiers in Plant Science 12, 668847.
| Crossref | Google Scholar | PubMed |
Yang J, Liu Z, Liu Y, Fan X, Gao L, Li Y, Hu Y, Hu K, Huang Y (2024) Genome-wide association study identifies quantitative trait loci and candidate genes involved in deep-sowing tolerance in maize (Zea mays L.). Plants 13(11), 1533.
| Crossref | Google Scholar | PubMed |
Zeileis A (2006) Object-oriented computation of sandwich estimators. Journal of statistical software 16, 1-16.
| Google Scholar |
Zhao Z, Wang E, Kirkegaard JA, Rebetzke GJ (2022) Novel wheat varieties facilitate deep sowing to beat the heat of changing climates. Nature Climate Change 12(3), 291-296.
| Crossref | Google Scholar |
Zhao X, Niu Y, Hossain Z, Zhao B, Bai X, Mao T (2023) New insights into light spectral quality inhibits the plasticity elongation of maize mesocotyl and coleoptile during seed germination. Frontiers in Plant Science 14, 1152399.
| Crossref | Google Scholar | PubMed |
Zhou P, Liu Y, Yang M, Yan H (2024) Genome-wide association study uncovers genomic regions associated with coleoptile length in a worldwide collection of oat. Genes 15(4), 411.
| Crossref | Google Scholar |