Efficacy of short-term cold storage prior to cryopreservation of spermatozoa in a threatened lizard
Lachlan Campbell A , John Clulow A , Belinda Howe A , Rose Upton

A School of Environmental and Life Sciences, University of Newcastle, Callaghan, NSW 2308, Australia.
B Department of Biological Sciences, University of South Florida, St. Petersburg, FL 33701, USA.
C Institute for Applied Ecology, University of Canberra, Bruce, ACT 2617, Australia.
D Department of Biological Sciences, Macquarie University, Sydney, NSW 2109, Australia.
E Corresponding author. Email: simon.clulow@canberra.edu.au
Reproduction, Fertility and Development 33(9) 555-561 https://doi.org/10.1071/RD20231
Submitted: 7 September 2020 Accepted: 21 January 2021 Published: 2 March 2021
Journal Compilation © CSIRO 2021 Open Access CC BY-NC
Abstract
Assisted reproductive technologies (ARTs) have a significant role to play in reptile conservation, yet are severely lacking. Previous attempts to cryopreserve spermatozoa in the threatened lizard Varanus panoptes achieved approximately 48% motile sperm post-thaw for samples frozen immediately after collection. However, the feasibility of extended cold storage before cryopreservation has not been tested. We held V. panoptes spermatozoa at either 25°C or 4°C for 8 days, assessing sperm motility at days 1, 2, 4 and 8. Subsamples were cryopreserved on days 1 and 4 following the previously reported protocol for this species. Percentage motility decreased rapidly at 25°C, but did not decrease significantly until 4 days after collection at 4°C, with >30% motility maintained after 8 days. There was no significant difference in post-thaw motility or viability of samples cryopreserved after 1 or 4 days storage at 4°C, yielding substantial results for both parameters (mean motility 23.8% and 28.1% and mean viability 50.1% and 57.5% after 1 and 4 days respectively). We demonstrate the capacity to extend sperm viability for up to 8 days in unfrozen samples and to produce acceptable post-thaw motility in samples frozen after 4 days of storage, contributing to the development of valuable ARTs for lizards and other reptiles.
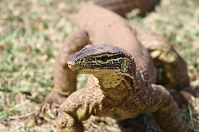
Keywords: assisted reproductive technologies, conservation, genome storage, reptile, squamate.
Introduction
Assisted reproductive technologies (ARTs) have provided an array of potential conservation tools for the effective management of wildlife genetic diversity from as early as the 1980s (Wildt et al. 1986, 2001; Holt et al. 2003; Pukazhenthi and Wildt 2004; Pukazhenthi et al. 2006; Clulow and Clulow 2016; Ryder and Onuma 2018; Upton et al. 2018), with a recent report also demonstrating significant economic benefits (Howell et al. 2020). However, these technologies have been based largely on models developed in mammalian livestock and humans (Comizzoli et al. 2018), with few taxon-specific protocols existing for non-model vertebrates such as amphibians and reptiles (Lawson et al. 2013; Clulow and Clulow 2016; Browne et al. 2019; Clulow et al. 2019). This is particularly the case for reptiles: despite current estimates that one in five reptilian species is threatened with extinction (Gibbons et al. 2000; Böhm et al. 2013), there appears to be little to no systematic management of gametes using ARTs to counter the loss of genetic diversity within affected populations (Clulow and Clulow 2016; Young et al. 2017). For example, lizards make up over 50% of all known reptiles, with more than 6000 species worldwide (Böhm et al. 2013), yet there have been only two reported studies investigating the effects of sperm cryopreservation for this group (Young et al. 2017; Campbell et al. 2020). In addition, little progress has been made to understand the fundamental conditions of sperm maintenance before or without cryopreservation (e.g. sperm cooling, extension medias) in lizards (Watson and Holt 2001; Molinia et al. 2010), which further limits the development of successful cryopreservation protocols. We are aware of only three studies that have investigated the holding of lizard spermatozoa at temperatures above 0°C (Molinia et al. 2010; Zimmerman et al. 2013; Young et al. 2017).
Short-term sperm storage at temperatures above freezing may be an important tool in cases where sperm samples need to be transported from the site of collection to the site of cryopreservation (e.g. remote field locations) or where females are held in different locations to males but it is possible to get to them within hours or days. Short-term sperm storage at temperatures >0°C before cryopreservation may allow for the successful transport of gametes before freezing without a significant loss of sperm motility and viability, although the small number of reports in reptiles to date suggests the response of spermatozoa to short-term storage varies among species (Gist et al. 2000; Fahrig et al. 2007; Zimmerman et al. 2013; Johnston et al. 2014; Young et al. 2017). Sperm motility in lizards following cold storage at 4°C has been investigated in the green iguana Iguana iguana (Zimmerman et al. 2013) and the black and white tegu Tupinambis merianae (Young et al. 2017), with promising values for the retention of motility after 48 h storage (77% and 60% of the initial motility respectively). Spermatozoa from McCann’s skink Oligosoma maccanni have been maintained at >70% motility for up to 5 days when stored at 4°C (Molinia et al. 2010).
We recently reported the development of a successful cryopreservation protocol for sperm harvested from the vas deferens of the yellow-spotted monitor Varanus panoptes (Campbell et al. 2020). This Australian lizard has recently become a threatened species due to the invasion of the cane toad Rhinella marina (Doody et al. 2009, 2017). In that study, spermatozoa were cryopreserved immediately after collection from the tract to determine appropriate cryodiluents and concentrations to maximise post-thaw motility, achieving recovery of approximately 48% motile spermatozoa following freeze–thawing (Campbell et al. 2020). In the present study, we aimed to investigate whether short-term sperm storage at temperatures >0°C in V. panoptes was possible to extend the time and range of collection and transport methods available. Specifically, our aims were to determine: (1) the length of time in which sperm motility can be maintained at either 4°C or 25°C; and (2) the effect that cold storage (4°C) has on the post-thaw motility of sperm stored for extended periods (1 or 4 days) before cryopreservation.
Materials and methods
Study species
The yellow-spotted monitor V. panoptes is a large (up to 1.5 m) Australian varanid lizard (Cogger 2014) that has been severely affected by the invasive cane toad R. marina (Doody et al. 2009, 2014, 2017). Affected populations decline through increased mortality rates resulting from lethal toxic ingestion when the toad is consumed as prey (Doody et al. 2009; Ujvari and Madsen 2009; Shine 2010). Since its introduction to Australia in 1935 (Lever 2006), the cane toad has caused severe population declines of up to 97% in V. panoptes (Doody et al. 2009), as well as apparent extirpations of this and at least one other monitor lizard in some parts of northern Australia (Doody et al. 2017). Because V. panoptes is a keystone predator, its decline has caused community-wide shifts in the abundance of predators and prey via trophic cascades (Doody et al. 2013, 2015, 2017). Thus, V. panoptes forms an ideal model for the development of reptile sperm storage and ARTs because it is abundant ahead of the toad front for conducting experiments and banking spermatozoa, but is threatened behind the invasion vanguard and exerts a disproportionately large effect over its ecosystem, thus warranting urgent conservation attention (Tingley et al. 2017).
Collection of animals, reproductive tracts and spermatozoa
V. panoptes males (n = 5) were collected from Fitzroy Crossing, Western Australia (−18.192410°S, 125.566893°E) in December 2017 during the wet season (November–April), when V. panoptes is active and mating is known to occur. Animals were captured in cage traps (810 mm × 254 mm × 305 mm; Havahart) baited with kangaroo tails and checked twice daily. Animals were killed within 2 days of capture by blunt cranial displacement followed by immediate severing of the spinal cord. Postmortem sample collection was necessary because other non-lethal collection methods (e.g. abdominal massage and electroejaculation) have not yet been established for monitor lizards. In addition, monitor lizards are frequently captured and used by the local Indigenous communities, which provided an opportunity to collect samples from individuals already designated as a food source. Each animal was weighed and measured (snout–vent length) immediately after it was killed and the reproductive tracts were removed by careful dissection. The intact tracts (including testes and vasa deferentia) were immersed in Dulbecco’s phosphate-buffered saline (PBS; Ca2+ and Mg2+ free; Sigma-Aldrich) to prevent desiccation. Both vasa deferentia were separated from the testes (which were weighed separately) and macerated in a 60 mm × 15 mm Petri dish containing 1 mL PBS by making several incisions along its entire length using surgical scissors and No. 4 forceps (Electron Microscopy Sciences). Each dish was allowed to stand for 2 min following maceration to release the spermatozoa from the tract. Free tissue was removed from each dish to create the final sperm suspension for experiments. The number of spermatozoa in each dish was determined for each pair of macerated vasa deferentia from duplicate counts in an improved Neubauer haemocytometer at a magnification of ×400, and motility at time zero (within 2 min of maceration) was assessed based on a duplicate count of at least 200 spermatozoa per dish.
All animal experiments and procedures were conducted with the approval of the University of Newcastle Animal Care and Ethics Committee (Ethics authorisation A-2016-601) and under Western Australian Scientific Licence 08-001546-1.
Short-term sperm storage
Two 500-µL samples were taken from the prepared sperm suspension of each animal (n = 5) and placed into 1.5-mL Eppendorf tubes (n = 10 tubes). One tube from each animal was held at 4°C in a standard refrigerator, whereas the other was held at ambient room temperature (25°C) for a period of 8 days (n = 5 for both treatments). A 10-µL subsample was removed from each tube at 24, 48, 96 and 192 h (1, 2, 4 and 8 days) postmortem and assessed for motility, determined as the percentage of forward-progressing spermatozoa. Spermatozoa that were twitching but not progressing forward were classed as immotile. Assessments were made in duplicate for each animal under a light microscope with phase optics (Olympus BH-2) at a magnification of ×400, scoring at least 200 spermatozoa from a minimum of three randomly selected fields for each of the duplicate replicates.
Cryopreservation
Three 50-µL subsamples were taken from each sperm suspension held at 4°C after 1 and 4 days incubation and added to 250-µL Cassou straws (IMV Technologies) for cryopreservation following the protocols developed previously by Campbell et al. (2020; n = 15 straws for each of Day 1 and 4). Briefly, each subsample was prepared by diluting the sperm–PBS solution 1:1 with a 20% v/v PBS–dimethyl sulfoxide (DMSO) solution to achieve a final concentration of 10% v/v DMSO. The straws were then cooled rapidly at a mean rate of approximately −32°C min−1 by suspending the straws horizontally on a rack 5 cm above liquid nitrogen in a styrofoam ice box, as described by Campbell et al. (2020). After 5 min, the straws were plunged directly into the liquid nitrogen before being transferred to a liquid nitrogen dewar (Taylor-Wharton) for storage. The samples were subsequently thawed after 24–29 months storage by immersing the straws into a 500-mL water bath at 35°C for 1 min. Each thawed straw was diluted 1:1 in PBS (Ca2+ and Mg2+ free) with caffeine (Sigma-Aldrich) added to produce a final concentration of 10 mM (to assess maximum motility), at which time the samples were left to incubate for 10 min at room temperature before assessment of sperm motility (Campbell et al. 2020). The percentage sperm motility for each straw was then assessed as described above. The percentage of viable spermatozoa with an intact plasma membrane in each straw was assessed in duplicate by staining samples with SYBR 14 and propidium iodide (Thermo Fisher) and examining them under a fluorescent microscope (Zeiss AX10), where spermatozoa with a damaged plasma membrane fluoresce red and those with an undamaged membrane fluoresce green (Zee et al. 2007). At least 200 spermatozoa were assessed for viability from a minimum of three randomly selected fields of view for each duplicated count.
Statistical analysis
Where appropriate, data are presented as the mean ± s.e.m. All data were analysed using the non-parametric Kruskal–Wallis test to determine the significance of differences between motility and viability across all time points within each temperature treatment on the assumption of non-normal data distribution. The non-parametric one-tailed Wilcoxon test was used for comparisons between individual matched treatments. Morphometric analysis was conducted using linear regressions. All analyses were performed in JMP Version 11 (SAS Institute).
Results
The body mass of individuals ranged between 1650 and 5150 g, but there were no significant differences in initial sperm motility between animals (χ2 = 6.27, d.f. = 4, P = 0.18; Table 1). Snout-to-vent length and bodyweight did not affect the weight of the testes (R2 = 0.63 (P = 0.11) and R2 = 0.75 (P = 0.06) respectively). Testes mass did not affect the sperm concentration (R2 = 0.12; P = 0.56).
Effect of sperm storage on sperm parameters before cryopreservation
Mean percentage motility decreased significantly with incubation period for unfrozen samples stored at both 4°C (χ2 = 33.97, d.f. = 3, P < 0.001) and 25°C (χ2 = 30.17, d.f. = 3, P < 0.001; Fig. 1). Storage temperature strongly affected the percentage motility of stored spermatozoa, with cold-stored sperm (4°C) maintaining higher motility after 1 day (χ2 = 21.77, d.f. = 1, P < 0.001) and 2 days (χ2 = 27.83, d.f. = 1, P < 0.001) than sperm stored at 25°C. After 4 days storage, all spermatozoa stored at 25°C had disintegrated and were no longer comparable to spermatozoa stored at 4°C, which maintained >30% motility throughout the 8-day experiment. There were no significant decreases in sperm motility from fresh collected spermatozoa (86.0 ± 0.7% motility at time zero) until 4 days storage, with spermatozoa held for 1 and 2 days at 4°C maintaining 87.2 ± 1.4% (Z = 0.71, P = 0.48) and 82.5 ± 2.7% (Z = −0.73, P = 0.47) motility respectively. There was a significant decrease in motility after 4 days cold storage (from 82.5% to 71.6 ± 3.9%; Z = −3.86, P < 0.001), and a further decrease after 8 days (to 33 ± 7%; Z = −4.37, P < 0.001), compared with the motility of fresh spermatozoa.
![]() |
Effect of sperm storage on subsequent cryopreservation and post-thaw recovery
Cryopreservation significantly reduced sperm motility of samples frozen and thawed after 1 and 4 days of storage at 4°C compared with the unfrozen spermatozoa sampled at the same time points. Mean post-thaw motility of spermatozoa cryopreserved after 1 day of storage at 4°C was 23.8% compared with a prefreeze motility of 87.2% in spermatozoa sampled at the same time point (χ2 = 19.29, d.f. = 1, P < 0.001; Fig. 2a). Mean post-thaw motility of spermatozoa stored for 4 days at 4°C before cryopreservation was 28.1%, compared with a prefreeze motility of spermatozoa sampled at the same storage time point of 71.6% (χ2 = 14.96, d.f. = 1, P < 0.001; Fig. 2a).
However, there was no significant difference in the post-thaw motility of spermatozoa stored for 1 or 4 days at 4°C (23.8 ± 4.6% vs 28.1 ± 5.1% respectively; χ2 = 0.13, d.f. = 1, P = 0.719; Fig. 2a). Similarly, there was no significant difference in post-thaw viability of spermatozoa frozen after 1 or 4 days storage at 4°C (50.1 ± 7.4% vs 57.5 ± 7.5%; χ2 = 0.23, d.f. = 1, P = 0.63; Fig. 2b). That is, cryopreservation after 4 days of storage was no worse for post-thaw sperm recovery than cryopreservation after 1 day of storage.
Discussion
The findings of the present study demonstrate two main outcomes with respect to short-term cold-storage and subsequent cryopreservation of V. panoptes spermatozoa: (1) short-term cold-storage of spermatozoa at 4°C for up to 8 days is possible, still yielding around 30% mean motility; and (2) spermatozoa can be cryopreserved after 4 days of cold storage at 4°C and recovered with relatively high rates of motility (28.1 ± 5.1%) and plasma membrane integrity (50.1 ± 7.5%). For comparison, ~48% motile spermatozoa were recovered previously in V. panoptes sperm cryopreserved immediately after individuals had been killed (Campbell et al. 2020). Together, these data demonstrate that short-term cold-storage of squamate reptile spermatozoa is a viable method for extending the amount of time that spermatozoa can be held before subsequent cryopreservation (e.g. spermatozoa collected from remote locations without access to liquid nitrogen for immediate cryopreservation) or for moving spermatozoa over large distances for inseminations requiring hours to days without cryopreservation at all. Although we did not test AI in the present study, these findings are novel for a threatened lizard species and are critical for future research involving insemination of cryopreserved spermatozoa in this species.
Spermatozoa retained the capacity for >70% mean motility for up to 4 days, which is markedly stronger than the results of two other studies that investigated sperm extension in lizards (Zimmerman et al. 2013; Young et al. 2017). Those studies found a significant decline in motility following just 1 day storage at 4°C; fresh motility of black and white tegu T. merianae spermatozoa declined from 93% to 77%, whereas the green iguana I. iguana exhibited even greater motility loss (from 78% to 60%; Zimmerman et al. 2013; Young et al. 2017). Nevertheless, the retention of overall high levels of motility in spermatozoa from all three phylogenetically distant lizard species for 1–4 days suggests that short-term storage of unfrozen spermatozoa may be a widely applicable ART tool across a broad range of lizard species. This could be encouraging for future integration of this routine into the management and manipulation of lizard gametes in ART procedures in conservation programs.
Differences in sperm survival and motility following storage at temperatures above 0°C between this and the other two reports may be due to species differences or to differences in sperm handling methods and collection procedures. For example, electroejaculation was used to collect semen samples from live individuals for the green iguana (Zimmerman et al. 2013), whereas spermatozoa were collected directly from the vas deferens in yellow-spotted monitors that had been killed (Campbell et al. 2020) and for the black and white tegu (Young et al. 2017). Sperm extension media also varied between Dulbecco’s PBS (Ca2+ and Mg2+ free), M199 with HEPES buffer and Ham’s F-10 with albumin (Zimmerman et al. 2013; Young et al. 2017; Campbell et al. 2020). Due to these potentially confounding differences in medium conditions and sperm collection procedures, it is not possible from the available data to determine whether the response of sperm motility differed between studies due to species specificity (e.g. Clulow et al. 2018) or the procedures themselves. Nevertheless, the results from the present study provide promising results even with a simple buffered saline medium. Future refinement may yield extended storage outcomes beyond those achieved in this study.
The ability to store spermatozoa and maintain motility is extremely beneficial for the collection of gametes from rare or genetically important individuals, which is an increasingly common challenge in conservation biology. In addition, short-term storage of cooled, but unfrozen, spermatozoa can be particularly useful in a conservation context where individuals are in remote or inaccessible sites in the wild, which is often the case for populations of the present study species (V. panoptes), with populations geographically distributed across remote and far north regions of the tropical savannahs of Australia. Cryopreservation protocols often rely on the use of specialised equipment, as well as the availability and transport of liquid nitrogen, which can be difficult to deploy and maintain in field conditions in the absence of laboratory facilities. Storing spermatozoa without requiring immediate cryopreservation while maintaining the capacity for relatively high motility greatly alleviates these logistical constraints. This capacity may reduce the safety risks for researchers dealing with liquid nitrogen in remote locations, while also eliminating the cell damage that can occur through sperm cryopreservation if spermatozoa can be collected, transported to a destination and used without the need for cryopreservation (e.g. such as may occur with transport to zoos and use in captive breeding programs).
Although the recovery of thawed spermatozoa of V. panoptes did not differ in samples cryopreserved between 1 and 4 days of unfrozen cold storage before freezing, the recovery did decline compared with post-thaw motility recorded for spermatozoa frozen immediately from fresh samples in a previous study: ~48% recovery recorded in samples frozen immediately after death in Campbell et al. (2020), compared with approximately 24% and 28% for 1 and 4 days respectively in the present study. Nonetheless, the observation that spermatozoa can survive 4 days of cold storage followed by freezing and subsequent thawing while still yielding approximately 28% motility is extremely promising. It is also worth noting that many of the non-motile spermatozoa after cryopreservation were still maintaining plasma membrane integrity as a measure of viability (almost as many as those that recovered motility). Although it was not tested in the present study, these may still be viable in the future for technologies such as intracytoplasmic sperm injection, that require functional nuclei and cytoplasmic content, but not motile sperm cells (Dozortsev et al. 1995). There is also the possibility that some of the spermatozoa processed that were viable, but not motile, may regain motility with the addition of other signal transduction pathway initiators (Armstrong et al. 1994). Further studies investigating the addition of extracellular compounds such as methylxanthine phosphodiesterase inhibitors other than caffeine and/or cAMP may provide a mechanism to increase post-thaw motility.
In summary, we demonstrated that V. panoptes spermatozoa can be cold stored for up to 4 days before cryopreservation without a significant decline in motility and for up to 8 days maintaining >30% motility. Spermatozoa can also be cryopreserved after 4 days of cold storage and recovered with relatively high rates of motility and plasma membrane integrity. Percentage motility and viability are parameters known to strongly predict the fertilising potential of spermatozoa (Birkhead et al. 1999; Burness et al. 2004; Gage et al. 2004). The strong post-thaw motility from the present study suggests that V. panoptes spermatozoa recovered after cryopreservation may be capable of fertilisation, although that hypothesis remains to be tested. Indeed, there are not yet reports of the successful generation of live young following insemination with cryopreserved spermatozoa in squamates or any other reptile. We hope the present study moves us one step closer to that goal.
Conflicts of interest
John Clulow and Simon Clulow are guest Associate Editors of Reproduction, Fertility and Development. Despite this relationship, they did not at any stage have editor-level access to this manuscript while in peer review, as is the standard practice when handling manuscripts submitted by an editor of this Journal. The authors have no further conflicts of interest to declare.
Acknowledgements
The authors acknowledge the Traditional Owners of Bunuba land, where this research was performed. The authors thank Julian Carson for assistance in the field and Shenae Cafe for assistance in the laboratory. This project was conducted with funding from the Jane Fenwick Foundation.
References
Armstrong, V. L., Clulow, J., Murdoch, R. N., and Jones, R. C. (1994). Intracellular signal transduction mechanisms of rat epididymal spermatozoa and their relationship to motility and metabolism. Mol. Reprod. Dev. 38, 77–84.| Intracellular signal transduction mechanisms of rat epididymal spermatozoa and their relationship to motility and metabolism.Crossref | GoogleScholarGoogle Scholar | 8049068PubMed |
Birkhead, T. R., Martinez, J. G., Burke, T., and Froman, D. P. (1999). Sperm mobility determines the outcome of sperm competition in the domestic fowl. Proc. Biol. Sci. 266, 1759–1764.
| Sperm mobility determines the outcome of sperm competition in the domestic fowl.Crossref | GoogleScholarGoogle Scholar | 10577160PubMed |
Böhm, M., Collen, B., Baillie, J. E. M., Bowles, P., Chanson, J., Cox, N., Hammerson, G., Hoffmann, M., Livingstone, S. R., Ram, M., et al. (2013). The conservation status of the world’s reptiles. Biol. Conserv. 157, 372–385.
| The conservation status of the world’s reptiles.Crossref | GoogleScholarGoogle Scholar |
Browne, R. K., Silla, A. J., Upton, R., Della-Togna, G., Marcec-Greaves, R., Shishova, N. V., Uteshev, V. K., Proaño, B., Pérez, O. D., Mansour, N., Kaurova, S. A., Gakhova, E. N., Cosson, J., Dyzuba, B., Kramarova, L. I., McGinnity, D., Gonzalez, M., Clulow, J., and Clulow, S. (2019). Sperm collection and storage for the sustainable management of amphibian biodiversity. Theriogenology 133, 187–200.
| Sperm collection and storage for the sustainable management of amphibian biodiversity.Crossref | GoogleScholarGoogle Scholar | 31155034PubMed |
Burness, G., Casselman, S. J., Schulte-Hostedde, A. I., Moyes, C. D., and Montgomerie, R. (2004). Sperm swimming speed and energetics vary with sperm competition risk in bluegill (Lepomis macrochirus). Behav. Ecol. Sociobiol. 56, 65–70.
| Sperm swimming speed and energetics vary with sperm competition risk in bluegill (Lepomis macrochirus).Crossref | GoogleScholarGoogle Scholar |
Campbell, L., Cafe, S. L., Upton, R., Doody, J. S., Nixon, B., Clulow, J., and Clulow, S. (2020). A model protocol for the cryopreservation and recovery of motile lizard sperm using the phosphodiesterase inhibitor caffeine. Conservation Physiology 8, coaa044.
| A model protocol for the cryopreservation and recovery of motile lizard sperm using the phosphodiesterase inhibitor caffeine.Crossref | GoogleScholarGoogle Scholar | 32607239PubMed |
Clulow, J., and Clulow, S. (2016). Cryopreservation and other assisted reproductive technologies for the conservation of threatened amphibians and reptiles: bringing the ARTs up to speed. Reprod. Fertil. Dev. 28, 1116–1132.
| Cryopreservation and other assisted reproductive technologies for the conservation of threatened amphibians and reptiles: bringing the ARTs up to speed.Crossref | GoogleScholarGoogle Scholar |
Clulow, J., Pomering, M., Herbert, D., Upton, R., Calatayud, N., Clulow, S., Mahony, M. J., and Trudeau, V. L. (2018). Differential success in obtaining gametes between male and female Australian temperate frogs by hormonal induction: a review. Gen. Comp. Endocrinol. 265, 141–148.
| Differential success in obtaining gametes between male and female Australian temperate frogs by hormonal induction: a review.Crossref | GoogleScholarGoogle Scholar | 29859744PubMed |
Clulow, J., Upton, R., Trudeau, V., and Clulow, S. (2019). Amphibian assisted reproductive technologies: moving from technology to application. In ‘Reproductive Sciences in Animal Conservation.’ pp. 413–463. (Springer)
Cogger, H. (2014). ‘Reptiles and amphibians of Australia.’ 7th edn. (CSIRO Publishing: Collingwood)
Comizzoli, P., Paulson, E., and McGinnis, L. (2018). The mutual benefits of research in wild animal species and human-assisted reproduction. J. Assist. Reprod. Genet. 35, 551–560.
| The mutual benefits of research in wild animal species and human-assisted reproduction.Crossref | GoogleScholarGoogle Scholar | 29470702PubMed |
Doody, J. S., Green, B., Rhind, D., Castellano, C. M., Sims, R., and Robinson, T. (2009). Population-level declines in Australian predators caused by an invasive species. Anim. Conserv. 12, 46–53.
| Population-level declines in Australian predators caused by an invasive species.Crossref | GoogleScholarGoogle Scholar |
Doody, J. S., Castellano, C. M., Rhind, D., and Green, B. (2013). Indirect facilitation of a native mesopredator by an invasive species: are cane toads re-shaping tropical riparian communities? Biol. Invasions 15, 559–568.
| Indirect facilitation of a native mesopredator by an invasive species: are cane toads re-shaping tropical riparian communities?Crossref | GoogleScholarGoogle Scholar |
Doody, J. S., Mayes, P., Clulow, S., Rhind, D., Green, B., Castellano, C., D’Amore, D., and McHenry, C. (2014). Impacts of the invasive cane toad on aquatic reptiles in a highly modified ecosystem: the importance of replicating impact studies. Biol. Invasions 16, 2303–2309.
| Impacts of the invasive cane toad on aquatic reptiles in a highly modified ecosystem: the importance of replicating impact studies.Crossref | GoogleScholarGoogle Scholar |
Doody, J. S., Soanes, R., Castellano, C. M., Rhind, D., Green, B., McHenry, C., and Clulow, S. (2015). Invasive toads shift predator–prey densities in animal communities by removing top predators. Ecology 96, 2544–2554.
| Invasive toads shift predator–prey densities in animal communities by removing top predators.Crossref | GoogleScholarGoogle Scholar | 26594710PubMed |
Doody, J. S., Rhind, D., Green, B., Castellano, C., McHenry, C., and Clulow, S. (2017). Chronic effects of an invasive species on an animal community. Ecology 98, 2093–2101.
| Chronic effects of an invasive species on an animal community.Crossref | GoogleScholarGoogle Scholar | 28477376PubMed |
Dozortsev, D., Rybouchkin, A., De Sutter, P., and Dhont, M. (1995). Sperm plasma membrane damage prior to intracytoplasmic sperm injection: a necessary condition for sperm nucleus decondensation. Hum. Reprod. 10, 2960–2964.
| Sperm plasma membrane damage prior to intracytoplasmic sperm injection: a necessary condition for sperm nucleus decondensation.Crossref | GoogleScholarGoogle Scholar | 8747054PubMed |
Fahrig, B. M., Mitchell, M. A., Eilts, B. E., and Paccamonti, D. L. (2007). Characterization and cooled storage of semen from corn snakes (Elaphe guttata). J. Zoo Wildl. Med. 38, 7–12.
| Characterization and cooled storage of semen from corn snakes (Elaphe guttata).Crossref | GoogleScholarGoogle Scholar | 17469269PubMed |
Gage, M. J., Macfarlane, C. P., Yeates, S., Ward, R. G., Searle, J. B., and Parker, G. A. (2004). Spermatozoal traits and sperm competition in Atlantic salmon: relative sperm velocity is the primary determinant of fertilization success. Curr. Biol. 14, 44–47.
| 14711413PubMed |
Gibbons, J. W., Scott, D. E., Ryan, T. J., Buhlmann, K. A., Tuberville, T. D., Metts, B. S., Greene, J. L., Mills, T., Leiden, Y., and Poppy, S. (2000). The Global Decline of Reptiles, Déjà Vu Amphibians. Bioscience 50, 653–666.
| The Global Decline of Reptiles, Déjà Vu Amphibians.Crossref | GoogleScholarGoogle Scholar |
Gist, D. H., Turner, T. W., and Congdon, J. D. (2000). Chemical and thermal effects on the viability and motility of spermatozoa from the turtle epididymis. J. Reprod. Fertil. 119, 271–277.
| Chemical and thermal effects on the viability and motility of spermatozoa from the turtle epididymis.Crossref | GoogleScholarGoogle Scholar | 10864839PubMed |
Holt, W., Abaigar, T., Watson, P., and Wildt, D. (2003) Genetic resource banks for species conservation. In ‘Reproductive Science and Integrated Conservation.’ (Eds W. V. Holt, A. R. Pickard, J. C. Rodger and D. E. Wildt) pp. 267–280. (Cambridge University Press: Cambridge)
Howell, L. G., Frankham, R., Rodger, J. C., Witt, R. R., Clulow, S., Upton, R. M., and Clulow, J. (2020). Integrating biobanking minimises inbreeding and produces significant cost benefits for a threatened frog captive breeding programme. Conserv. Lett , .
| Integrating biobanking minimises inbreeding and produces significant cost benefits for a threatened frog captive breeding programme.Crossref | GoogleScholarGoogle Scholar |
Johnston, S., Lever, J., McLeod, R., Qualischefski, E., Brabazon, S., Walton, S., and Collins, S. (2014). Extension, osmotic tolerance and cryopreservation of saltwater crocodile (Crocodylus porosus) spermatozoa. Aquaculture 426–427, 213–221.
| Extension, osmotic tolerance and cryopreservation of saltwater crocodile (Crocodylus porosus) spermatozoa.Crossref | GoogleScholarGoogle Scholar |
Lawson, B., Clulow, S., Mahony, M. J., and Clulow, J. (2013). Towards gene banking amphibian maternal germ lines: short-term incubation, cryoprotectant tolerance and cryopreservation of embryonic cells of the frog, Limnodynastes peronii. PLoS One 8, e60760.
| Towards gene banking amphibian maternal germ lines: short-term incubation, cryoprotectant tolerance and cryopreservation of embryonic cells of the frog, Limnodynastes peronii.Crossref | GoogleScholarGoogle Scholar | 23577155PubMed |
Lever, C. (2006). ‘The Cane Toad: The History and Ecology of a Successful Colonist.’ (Westbury Academic & Scientific Publishing: Otley)
Molinia, F. C., Bell, T., Norbury, G., Cree, A., and Gleeson, D. M. (2010). Assisted breeding of skinks or how to teach a lizard old tricks. Herpetol. Conserv. Biol. 5, 311–319.
Pukazhenthi, B. S., and Wildt, D. E. (2004). Which reproductive technologies are most relevant to studying, managing and conserving wildlife? Reprod. Fertil. Dev. 16, 33–46.
| Which reproductive technologies are most relevant to studying, managing and conserving wildlife?Crossref | GoogleScholarGoogle Scholar | 14972101PubMed |
Pukazhenthi, B., Comizzoli, P., Travis, A. J., and Wildt, D. E. (2006). Applications of emerging technologies to the study and conservation of threatened and endangered species. Reprod. Fertil. Dev. 18, 77–90.
| Applications of emerging technologies to the study and conservation of threatened and endangered species.Crossref | GoogleScholarGoogle Scholar | 16478605PubMed |
Ryder, O. A., and Onuma, M. (2018). Viable cell culture banking for biodiversity characterization and conservation. Annu. Rev. Anim. Biosci. 6, 83–98.
| Viable cell culture banking for biodiversity characterization and conservation.Crossref | GoogleScholarGoogle Scholar | 29447472PubMed |
Shine, R. (2010). The ecological impact of invasive cane toads (Bufo marinus) in Australia. Q. Rev. Biol. 85, 253–291.
| The ecological impact of invasive cane toads (Bufo marinus) in Australia.Crossref | GoogleScholarGoogle Scholar | 20919631PubMed |
Tingley, R., Ward-Fear, G., Schwarzkopf, L., Greenlees, M. J., Phillips, B. L., Brown, G., Clulow, S., Webb, J., Capon, R., Sheppard, A., Strive, T., Tizard, M., and Shine, R. (2017). New weapons in the Toad Toolkit: a review of methods to control and mitigate the biodiversity impacts of invasive cane toads (Rhinella marina). Q. Rev. Biol. 92, 123–149.
| New weapons in the Toad Toolkit: a review of methods to control and mitigate the biodiversity impacts of invasive cane toads (Rhinella marina).Crossref | GoogleScholarGoogle Scholar | 29562120PubMed |
Ujvari, B., and Madsen, T. (2009). Increased mortality of naïve varanid lizards after the invasion of non-native cane toads (Bufo marinus). Herpetol. Conserv. Biol. 4, 248–251.
Upton, R., Clulow, S., Mahony, M. J., and Clulow, J. (2018). Generation of a sexually mature individual of the Eastern dwarf tree frog, Litoria fallax, from cryopreserved testicular macerates: proof of capacity of cryopreserved sperm derived offspring to complete development. Conserv. Physiol. 6, coy043.
| Generation of a sexually mature individual of the Eastern dwarf tree frog, Litoria fallax, from cryopreserved testicular macerates: proof of capacity of cryopreserved sperm derived offspring to complete development.Crossref | GoogleScholarGoogle Scholar | 30151196PubMed |
Watson, P., and Holt, W. V. (2001). ‘Cryobanking the genetic resource: Wildlife conservation for the future?’ (Taylor & Francis: London)
Wildt, D. E., Schiewe, M., Schmidt, P., Goodrowe, K., Howard, J., Phillips, L. G., O’Brien, S. J., and Bush, M. (1986). Developing animal model systems for embryo technologies in rare and endangered wildlife. Theriogenology 25, 33–51.
| Developing animal model systems for embryo technologies in rare and endangered wildlife.Crossref | GoogleScholarGoogle Scholar |
Wildt, D. E., Ellis, S., and Howard, J. G. (2001). Linkage of reproductive sciences: from’quick fix’to’integrated’conservation. J. Reprod. Fertil. Suppl. 57, 295–307.
| 11787164PubMed |
Young, C., Ravida, N., Curtis, M., Mazzotti, F., and Durrant, B. (2017). Development of a sperm cryopreservation protocol for the Argentine black and white tegu (Tupinambis merianae). Theriogenology 87, 55–63.
| Development of a sperm cryopreservation protocol for the Argentine black and white tegu (Tupinambis merianae).Crossref | GoogleScholarGoogle Scholar | 27639519PubMed |
Zee, Y. P., Holt, W. V., Allen, C. D., Nicolson, V., Burridge, M., Lisle, A., Carrick, F. N., and Johnston, S. D. (2007). Effects of cryopreservation on mitochondrial function and heterogeneity, lipid raft stability and phosphatidylserine translocation in koala (Phascolarctos cinereus) spermatozoa. Reprod. Fertil. Dev. 19, 850–860.
| Effects of cryopreservation on mitochondrial function and heterogeneity, lipid raft stability and phosphatidylserine translocation in koala (Phascolarctos cinereus) spermatozoa.Crossref | GoogleScholarGoogle Scholar | 17897588PubMed |
Zimmerman, D. M., Mitchell, M. A., and Perry, B. H. (2013). Collection and characterization of semen from green iguanas (Iguana iguana). Am. J. Vet. Res. 74, 1536–1541.
| Collection and characterization of semen from green iguanas (Iguana iguana).Crossref | GoogleScholarGoogle Scholar | 24274892PubMed |