Forest fires in Mexico: an approach to estimate fire probabilities
Luis Galván A C and Víctor Magaña BA Posgrado en Geografía, Universidad Nacional Autónoma de México, Mexico City 04510, Mexico.
B Instituto de Geografía, Universidad Nacional Autónoma de México, Mexico City 04510, Mexico.
C Corresponding author. Email: cohuatl09@gmail.com
International Journal of Wildland Fire 29(9) 753-763 https://doi.org/10.1071/WF19057
Submitted: 11 April 2019 Accepted: 2 May 2020 Published: 3 June 2020
Journal Compilation © CSIRO 2020 Open Access CC BY-NC-ND
Abstract
The probabilities of forest fires in Mexico are estimated using information on precipitation and temperature, along with data on type of vegetation, human activities near forests and fire prevention policies. The proposed model addresses the factors that account for extreme wildfire hazard, and may provide a basis for fire prevention actions, reducing vulnerability factors.
Additional keywords: climate, drought, natural hazard, vulnerability.
Introduction
Forests provide important ecosystem services, such as climate regulation, recharge of aquifers, food production and greenhouse gas sequestration (e.g. Krieger 2001). For these services to continue, these ecosystems should be conserved or recovered, particularly in areas affected by human activities (Food and Agriculture Organization (FAO) 2014). In many parts of the world, forest fires frequently have negative impacts in the short and long term, because the recovery of the associated ecosystem services may take several years. It is estimated that each year, ~350 million ha of natural areas suffers fire damage around the world (FAO 2007). The resulting CO2 emissions are almost 50% of those produced by burning fossil fuels (Jolly et al. 2015). At times, forest fires may have positive effects in ecosystems (Ressl and Cruz 2012), as maintaining appropriate burning helps to reduce fuel hazard and to maintain the nutrient cycle in pine forests (e.g. Rodríguez-Trejo and Fulé 2003).
In several countries in the tropics, the main cause of forest fires is human activities related to land-use change or agriculture (Nobre and De Simone 2009; Costafreda-Aumedes et al. 2017). Various studies have used the term risk of forest fire to refer to the probability of anthropic and natural fires. However, fire effects in a risk analysis should consider the likelihood of a fire burning and the variety of values susceptible to wildland fire (Finney 2005).
Every year numerous forest fires in Mexico are reported, and preventive actions are still insufficient (Zúñiga-Vásquez et al. 2017a). Although there has been progress in actions to detect and control forest fires, there is still significant work to be done to prevent human-induced forest fires. Prevention requires appropriate climate information and complete analyses of vulnerability to adverse climatic conditions in order to have better estimates of fire probabilities, particularly during dry and hot years (Anderson et al. 2019). Forest fires in Mexico are estimated to be between 40 and 99% related to human activities (Comisión Nacional Forestal (CONAFOR) 2001; Pompa-Garcia et al. 2018). Meteorological droughts increase the chances of forest fires, as in 1998 and in 2011 (Magaña 1999; Vose et al. 2016). During an El Niño year, rainfall may be below normal in the central and southern parts of the country and, at times, this may turn into a severe meteorological drought. In 1997, the rainfall deficit associated with El Niño caused an extreme drought that contributed to a record number of fires in the tropical forests of Mexico during the spring of 1998 (CONAFOR 2009). In 2011, a prolonged extreme drought took place in northern Mexico, and anomalously high temperatures exceeded 40°C for several days during the spring months. Lightning activity in northern Mexico appears to have led to several forest fires that devastated large areas of coniferous forests in the state of Coahuila (Magaña and Neri 2012; Zúñiga-Vásquez et al. 2017b).
The frequency of forest fires in Mexico is partially related to climate anomalies and frequently to human activities that involve the use of fire. The vulnerability of forests to anomalously dry and hot climatic conditions depends, among other things, on the proximity of agricultural activities to forests. It is estimated that agricultural activities induce at least 40% of forest fires in Mexico (CONAFOR 2001), owing to the traditional practice of slash and burn. Intentional burning for agricultural purposes also results in forest fires (Bravo‐Espinosa et al. 2014), particularly during the dry season or periods of meteorological drought. Vulnerability also depends on accessibility to forest ecosystems, the distance of forests to other human activities (e.g. road traffic), or practices aimed at land-use change (Pérez et al. 2013). Consequently, the probabilities of forest fires come from a climatic hazard related to meteorological droughts (precipitation deficit, positive temperature anomalies and hydrologically stressed vegetation), in a vulnerability context, mainly associated with human activities (e.g. agriculture). This approach to estimate climatic risk has been followed in several studies that analyse the potential impacts of climate change (Intergovernmental Panel on Climate Change (IPCC) 2012).
In general, climatic risk is given in terms of the probabilities of natural hazard times vulnerability, as an index that reflects social, economic and environmental conditions, and the exposure of the system that may be affected (IPCC 2012; Neri and Magaña 2016). Finney (2005) states that fire risk analysis depends on characterising and combining fire behaviour probabilities and effects, i.e. the consequences. Meteorological droughts are considered one of the most serious climatic hazards that increase forest fire probabilities. McKee et al. (1993) determined that moderate drought occurs 9.2% of the time, severe drought 4.4% and extreme drought 2.3% worldwide. Therefore, the probability of a meteorological drought (moderate to extreme), characterised with a Standardised Precipitation Index (SPI-3) less than –1, is ~16%. Actual manifestations of this type of climatic hazard have occurred in 1998 in central southern México, during an El Niño event, and in 2011 in northern Mexico, conditions that are referred to as hazardous events. The chances of positive temperature anomalies are 50%, assuming a normal distribution of the variable. Similarly, the probability for a negative anomaly of the Normalized Difference Vegetation Index (NDVI) is 50%, assuming a normal distribution of this variable. Consequently, the joint probability for a natural hazard that may lead to forest fires is of the order of 4%, i.e. a hazardous climatic condition of this kind may approximately occur once every 25 years. However, forest fires in Mexico occur much more frequently than that. This implies that probabilities of forest fires should include the vulnerability of the natural system, mainly related to human factors (Avila-Flores et al. 2010; Pompa-Garcia et al. 2018).
In Mexico, response and recovery actions after natural disasters are more common than prevention. The capacity to control forest fires appears to have improved more than the capacity to prevent them. The number of forest fires during the last 45 years has increased at a more rapid rate than affected area (Fig. 1). Dry conditions, like the severe meteorological drought in 2011 in central northern Mexico, are the most important climatic factor that leads to a record area affected by fire. However, forest fires in the central and southern regions appear to depend mostly on human factors (Pompa-Garcia et al. 2018).
There is interest within the Mexican government to prevent forest fires, but this effort requires a diagnosis of the factors (natural and human) that induce fires, and risk management strategies through the reduction of vulnerability to drought, for instance. The main objective of the present paper is to develop a method for the quantification of the probabilities of forest fire in Mexico that helps to promote forest fire prevention strategies, identifying the importance of natural and human factors.
Data and methodology
An evaluation of probabilities of forest fire requires characterisation of the climatic conditions and the corresponding physical, economic and social vulnerability factors. Climatic hazards are usually expressed in terms of probability based on historical records. Meteorological drought (i.e. the natural hazard) is a recurrent condition that may be represented in terms of indices, such as the SPI (McKee et al. 1993, 1995). This factor serves to describe adverse climatic conditions related to drought. In the present study, the climatic hazard corresponds to a combination of factors, including:
Three-month SPI-3 data (Chen et al. 2002) to describe meteorological drought conditions in 0.5° × 0.5° grids, for the 1970–2015 period. Fernandes et al. (2011) and Galván (2011) found a strong correlation between SPI-3 and forest fires;
Monthly surface maximum temperature anomalies from the North American Regional Reanalysis (NARR 2004), in a 0.3° × 0.3° mesh, for the 1979–2015 period; and
Bi-weekly anomalies of the NDVI (Rouse et al. 1974), obtained from the US Geological Survey (Huete et al. 2002), in 250 × 250-m grids, for the 2001–15 period.
To combine hazard data into a single grid, coarse-resolution data were linearly interpolated into a 250 × 250-m grid, corresponding to the high spatial resolution of the NDVI data. In each grid cell, SPI-3, temperature anomalies and NDVI data were normalised between 0 and 1 using the relationship:
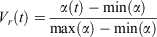
where Vr refers to the normalised value of the climate variable, α(t) is a climate variable and max (α) and min (α) correspond to its maximum and minimum values. The linear combination of the normalised climate variables results in a climatic hazard index.
Vulnerability may be estimated using information that relates to physical, environmental and socioeconomic factors. In this way, qualitative information is translated into quantitative data of vulnerability using official agencies’ data for recent years. The various vulnerability indicators vary in time to reflect the dynamic nature of vulnerability.
According to CONAFOR (2010), the main causes of forest fires are related to the use of fire in agricultural or cattle ranching activities. Vulnerability of natural ecosystems to dry periods also increases from other human activities related to land-use change, illegal forest activities, rights of way, burning of garbage or fire for poaching. These factors can be represented directly or indirectly with data from official sources. For instance, data on the density of the rural population in Mexico and their activities, along with data on built-up areas, roads, railroads, shorelines, land use and cover, and night-time lighting have been used to construct vulnerability indicators. These factors, along with data on the Mexican population, were used to construct a Human Influence Index (HII) (Wildlife Conservation Society Center for International Earth Science Information Network (WCS-CIESIN) 2005). The HII provides information on the direct influence of humans on ecosystems over North America. Its scale ranges from 0 to 100, where 0 represents no human influence and 100 represents maximum human influence.
Regional or local public policies aimed at preventing the occurrence of forest fires are also a key element in the reduction of vulnerability, and therefore, the probability of forest fires. Data on the number of forest fires indicate that in Natural Protected Areas (NPAs), fire prevention practices are more efficient than in the outside rural regions. This makes NPAs less vulnerable to meteorological droughts, which can be represented as an indicator of vulnerability between 0 and 1 (CONAFOR 2012; Comisión Nacional de Áreas Naturales Protegidas (CONANP) 2013).
Various studies have been developed to characterise the sensitivity of vegetation to fire (e.g. Pyrke and Marsden 2005). In Mexico, this type of characterisation of vulnerability based on the susceptibility of forests and rainforests to fire has been developed by the National Commission on Biodiversity (CONABIO 1998). Certain types of vegetation are more likely to catch fire than others (Table 1). These data have been converted into an indicator of vulnerability between 0 and 1.
![]() |
An index of vulnerability to meteorological drought is constructed by averaging a normalised version (between 0 and 1) of the three indicators listed above. For instance, in northern Coahuila (102°W, 29°N), the human influence index is between 0.2 and 0.3 (low vulnerability), the susceptibility of the vegetation is between 0.6 and 0.8 (high vulnerability), and the protected area condition corresponds to ~0.1 (low vulnerability). The average normalised vulnerability index is ~0.3–0.4, which corresponds to low vulnerability. The vulnerability index is evaluated in a 250 × 250-m grid. It is clear the vulnerability factors are dynamic and should be updated periodically. Using the hazardous event data and vulnerability index, an estimate of the probability of forest fires is obtained to develop a case study for the spring of 2011, when a severe prolonged meteorological drought affected northern Mexico.
A measure of forest fire activity was obtained using hotspots detected by satellite (MODIS (Moderate-Resolution Imaging Spectroradiometer) Collection 6), defined with an 80% or above confidence level. This information was obtained from the National Aeronautics and Space Administration (NASA)-Fire Information for Resource Management System (FIRMS) for the 2001–15 period. The accuracy of the forest fires estimates for the 2011 episode was determined by comparing the ranks of probabilities of forest fires with the density of observed hotspots. The number and location of hotspots were also compared with climate information only to establish the advantage of using vulnerability information for the probabilities of forest fires.
Results
Forest fires in Mexico: temporal behaviour
In Mexico, the number of forest fires shows a maximum during the spring months (April to May) (Fig. 2), i.e. during the period of minimum precipitation, when the highest maximum temperatures are observed (Magaña 1999). After the onset of the rainy season, between June and July, few forest fires are detected. Therefore, most of the analysis focuses on forest fire activity during the first half of the year. The seasonality in forest fires also coincides with the beginning of the spring–summer rain-fed agricultural cycle, when slash and burn practices are common over most of the country.
![]() |
The years 2003 and 2011 correspond to periods of prolonged (2 to 3 years) and intense (SPI-3 <−2) meteorological droughts in northern Mexico. The periods with minimum forest fire activity were related to normal or wet winter–spring conditions, as in 2014. A large number of forest fires may occur even in wet years (e.g. 1986), suggesting the importance of human activity in the occurrence of these events. As the rural population increases, more human activities raise the probability of forest burns (Fig. 3). The rural population has increased approximately at a rate of 0.5% per year, from ~33 million in 1970 to more 58 million in 2015 (World Bank 2017). As there is no clear trend in dry or wet periods in Mexico in recent decades, the tendency for more forest fires may be related to more rural population and human activities. A preliminary (spatially averaged) estimate of probabilities of forest fires may be obtained by combining information on the increase in rural population and the spatial average of SPI-3. In some years, droughts tend to favour a significant increase in the number of fires, particularly in northern México (Pompa-Garcia et al. 2018). The correlation between preliminary approximation of the chances of forest fire activity and actual number of fires is 0.85 for northern Mexico, and 0.54 for southern Mexico. In this preliminary approximation, which combines rural population and SPI-3 data (as a precipitation hazard index between 0 and 1), the correlation mostly reflects the coherence in high-frequency variations regulated by climate variability, whereas the positive long-term trend in forest fires appears to be associated with the increase in human activities of the rural population.
![]() |
Forest fire prevention policies reduce the vulnerability to drought. In NPAs, there are forest fire prevention policies that make these regions less vulnerable during dry and hot conditions (CONANP 2014). These preventive policies include clearing paths in the forests, removal of fuel, and skill creation for the personnel in charge of forest surveillance.
The chances of forest fire may also depend on the type of exposed vegetation, which may determine fire spread.
The previous vulnerability factors may be quantified in terms of indicators that in turn may be averaged into a consolidated vulnerability index. In the present analysis, a larger weight (0.5) was empirically given to the HII, considering it plays a major role in the number of forest fires. Public policies related to the level of protection or ecosystem management, as well as the characteristics of the exposed vegetation, were given a weight of 0.25 each in the averaging process to obtain the vulnerability index. When this index was used in combination with climatic hazardous event data, an estimate of the probabilities of forest fires was obtained. The estimate of forest fires was used to evaluate the specific case of the spring of 2011, a particularly dry year.
Case study: the drought of 2011
The natural hazard
To analyse spatial contrasts in the occurrence of forest fires in Mexico, the conditions during the severe drought of 2011 were analysed. A large area (~300 000 ha) of pastures, forests and rainforests burned, mainly in northern Mexico during that year (Zúñiga-Vásquez et al. 2017b). The SPI-3 during April 2011 reached values below –2, which is considered an extreme drought (Fig. 4a). The maximum temperature anomalies in the northern and central regions of Mexico were between +2 and +3°C above average (Fig. 4b). These conditions combined with negative NDVI anomalies that correspond to severe water stress in the vegetation, mostly over north-western and north-eastern Mexico (Fig. 4c). Water stress in the vegetation along the Gulf of Mexico and over the Yucatan peninsula was also large. When these factors were combined into a climate hazard index, north-eastern Mexico faced an important hazardous event, and the region was at high risk of forest fires (Fig. 4d).
The spatial pattern of the 2011 drought in northern Mexico included areas severely affected by forest fires, but a closer look at the distribution of hotspots shows that these events also occurred in regions not affected by drought, such as the western-central part of Mexico (Fig. 4d).
Vulnerability
The HII is an important factor in the probability of forest fires. In order to determine the origin of recurrent burn activity in some parts of Mexico, this factor should be examined at the regional and local level. Most of southern and central Mexico forests are particularly vulnerable given that more than 60% of the Mexican population is concentrated there (Fig. 5a). Agricultural practices frequently make use of slash and burn (Gómez et al. 1993) and further, some fires in recent years have been intentional, in order to expand the areas for avocado production and other crops, particularly in western Mexico (Bravo‐Espinosa et al. 2014). The type of vegetation is also a factor in vulnerability. For instance, during the dry season from March to May, low deciduous forest tends to be highly flammable (Table 1), as in the western Yucatan Peninsula (Fig. 5b). Finally, ecosystem management policies appear to make NPAs (Fig. 5c) less vulnerable during dry and hot climatic episodes. The weighted average of the normalised indicators results in a consolidated vulnerability index (Fig. 5d), showing areas of high vulnerability mostly where the HII is large. The regions of high forest fire activity are mainly located in southern and central Mexico, which are in turn areas of high vulnerability.
The highest climatic vulnerability is located in the southern and coastal parts of western Mexico and the Gulf of Mexico. In the Yucatan peninsula for instance, the western part is more vulnerable than the eastern part, corresponding with the type of vegetation and its flammability. The western part of Yucatan, as well as the western-central part of Mexico, are two regions that are severely affected by forest fires year after year, almost independently of climatic conditions (Fig. 5d). When the hazard index was combined with the vulnerability index, an estimate of probabilities of forest fires was obtained, which can be represented in an informal ranking as very high, high, medium, low and very low probabilities.
Probabilities of forest fires in spring 2011
During the spring of 2011, the number of forest fires significantly exceeded the seasonal average (Neri and Magaña 2016), and most hotspots were located in regions of very high and high burn probabilities (Fig. 6). In the northern part of the state of Coahuila, forest fires mainly occurred in regions of very high and high risk, outside NPAs. However, some forest fires were also detected within NPAs with low and very low probability values that resulted from the propagation of fires from neighbouring zones. High and very high forest fire probabilities were observed in some parts of the Yucatan Peninsula or in western central Mexico, mainly related to high and very high vulnerability. Nevertheless, numerous forest fires occurred in regions of medium, low and very low probability of fire, because the number of induced fires was difficult to estimate.
![]() |
A simple evaluation of the forest fire (estimate) model was conducted using the number of hotspots in the areas of very low to very high probabilities. Results show that most forest fires (72%) occur when probabilities are very high, high and medium (Fig. 7a). When only climatic hazard information is used, results tend to be less accurate (Fig. 7b). Therefore, the analysis that combines climatic hazards and vulnerability factors is more accurate than the climatic information (hazardous condition for April 2011) to estimate forest fire probabilities. Areas with a low level of fire probability also include a large number of hotspots. This is mainly related to a significant number of fires, of medium and low fire probability ranking, in the western state of Michoacan and in the Yucatan peninsula. In the former, the large number of hotspots reflects a vulnerability level higher than estimated, because intentional burning of natural forests to expand area for avocado orchards (Barsimantov and Antezana 2012; Bravo‐Espinosa et al. 2014) was not considered. This effect was not well captured by the HII used in the present analysis. In the Yucatan peninsula, a similar tendency to a large number of forest fires exists in relation to forest clearing activities for agricultural and cattle ranching expansion purposes (Cheng et al. 2013). The evaluation tends to be more biased towards higher ranks of probability of fire when Michoacan and Yucatan are not included in the evaluation. This implies that the HII should be modified in these regions considering the exacerbated use of fire.
![]() |
Predicting the probabilities of forest fires
One of the goals of environmental policies in Mexico is to reduce the number of forest fires. For this purpose, the use of climate information is important for climate risk management with preventive actions. Seasonal climate forecasts, regularly issued, and vulnerability estimates may be combined to produce predictions of probabilities of forest fires. For instance, the meteorological drought of spring 2011 constitutes a good example on how this type of information may be constructed. The 1-month climate forecast, such as that prepared by the Geophysical Fluid Dynamics Laboratory (GFDL) in March 2011 (Kirtman et al. 2014; NOAA-GFDL 2014), indicated that the meteorological drought would continue during April 2011, mainly in the states adjacent to the Gulf of Mexico, with precipitation anomalies close to –2 (Fig. 8a). Temperature anomalies between +1 and +1.5°C were also predicted, mainly in northern Mexico (Fig. 8b). Such conditions would lead to severe water stress in the vegetation. However, forecasts of NDVI anomalies were not available, so the hazardous event index was calculated with precipitation and temperature anomalies only. The combination of these elements resulted in high and very high hazardous climatic conditions in north-eastern Mexico, and medium, low and very low climatic hazard in the rest of the country (Fig. 8c).
When the information on the predicted climatic hazard was combined with a recent estimate of vulnerability, a forecast of forest fire probabilities was obtained, for instance, 1 month in advance (Fig. 9). The climate forecast for April 2011 indicated that areas of forests and rainforests in north-eastern Mexico and the Gulf of Mexico states could be affected by fire. A comparison of this forecast with the hotspots observed in April 2011 indicates that forest fire occurrence was approximately predicted in north-eastern Mexico in a better way than could have been predicted with the climate forecast only. However, in the western part of the Yucatan peninsula and western central Mexico (Michoacan state), where medium levels of burn probabilities were predicted, numerous hotspots were observed. These regions have been identified as zones of recurrent and intense forest fire activity, well above the rest of the country. This result suggests a more regional analysis should be conducted in these locations, considering that most forest fires are mainly due to negligence or lit on purpose.
![]() |
Conclusions
The number of forest fires in Mexico has increased in recent decades and their environmental, economic and social costs are significant. The present study estimates the probabilities of forest fires in terms of climatic anomalies and vulnerability conditions mainly related to human activities. There are no universally accepted methods to quantify vulnerability, but it may be estimated based on indicators related to human activities, the characteristics of vegetation and public policies aimed at preventing forest fires. The combination of these factors and hazard data results in a model that better explains probabilities of forest fires than the approach based on climate information only. Vulnerability explains the trends and low-frequency (decadal) variability in the number of forest fires, while climate information modulates the interannual variations.
Some regions of Mexico experience forest fires even when no significant climatic anomalies are observed, as in the western central part of Mexico or the Yucatan peninsula. It has been determined that these fires are intentionally caused to clear forest for agriculture, to remove residual crop biomass and to maintain already modified land (milpa, sugarcane and pasture) (Cheng et al. 2013). The present analysis suggests that, in terms of the probabilities of forest fires, climate anomalies are more important in the northern part of Mexico, while vulnerability factors (mainly human activity) are more relevant in the central southern part.
Identification of the factors that result in vulnerability is a key step in risk management. The present analysis scheme consists essentially of translating qualitative diagnosis of vulnerability into quantifications that lead to an index of vulnerability under meteorological drought. Results indicate that the weight given to vulnerability should vary from one region to another. It should be larger in regions where forest fires are intentionally caused by human activities owing to negligence or intentionally during the dry season, as in Michoacan or Yucatan. In these regions, public policies should be improved to reduce or eliminate practices such as slash and burn. Other policies such as an early warning system are particularly relevant when meteorological droughts are predicted in highly vulnerable areas.
Evaluation of the forest fire probability model of the present study requires a recurrent update of vulnerability factors. Unfortunately, the HII factor or vegetation susceptibility to fire is not always easy to evaluate in a yearly manner. Thus, the uncertainty in the predictions of probabilities of forest fires should be examined. Constructing actual time series of vulnerability, at least every 5 years, and then developing an evaluation of the model for a period such as 1980–2015 would improve the model.
A more accurate approach of predicting probabilities of forest fires should consider an ensemble of climate predictions and the consequences of such events (Finney 2005). This would serve to determine the level of critical risk considered as the threshold, for instance, for the implementation of an early warning system.
One of the major challenges that tropical and subtropical regions will face under climate change is the prevention of forest fires. Identification of the causes of such type of events opens the possibility of implementing more efficient adaptation measures in the long term. The adequate management of climatic risk may be crucial in the conservation of the planet’s natural resources.
Conflicts of interest
The authors declare no conflicts of interest.
Acknowledgements
This paper was partially supported by CONACYT-INEGI fund 209932 and PAPIIT IN112717. The first author was financially supported with a CONACYT scholarship. We appreciate the technical assistance of Mr Gustavo Vázquez. Some of the data used for the present study were obtained in collaboration with the Laboratorio Nacional de Observación de la Tierra (LANOT)-UNAM. The technical support of VARICLIM is appreciated. The comments of two anonymous reviewers are highly appreciated.
References
Anderson LO, Marchezini V, Morello TF, Cunningham C (2019) Modelo conceitual de sistema de alerta e de gestão de riscos associados a incêndios florestais e desafios para políticas públicas no Brasil. Territorium 26, 45–63.| Modelo conceitual de sistema de alerta e de gestão de riscos associados a incêndios florestais e desafios para políticas públicas no Brasil.Crossref | GoogleScholarGoogle Scholar |
Avila-Flores FD, Pompa GM, Antonio NS, Rodríguez TD, Vargas PE, Santillán PJ (2010) Driving factors for forest fire occurrence in Durango state of Mexico: a geospatial perspective. Chinese Geographical Science 20, 491–497.
| Driving factors for forest fire occurrence in Durango state of Mexico: a geospatial perspective.Crossref | GoogleScholarGoogle Scholar |
Barsimantov J, Antezana NJ (2012) Forest cover change and land tenure change in Mexico’s avocado region: is community forestry related to reduced deforestation for high value crops? Applied Geography 32, 844–853.
| Forest cover change and land tenure change in Mexico’s avocado region: is community forestry related to reduced deforestation for high value crops?Crossref | GoogleScholarGoogle Scholar |
Bravo‐Espinosa M, Mendoza ME, Carlón-Allende T, Medina L, Sáenz‐Reyes JT, Páez R (2014) Effects of converting forest to avocado orchards on topsoil properties in the Trans‐Mexican volcanic system, Mexico. Land Degradation & Development 25, 452–467.
| Effects of converting forest to avocado orchards on topsoil properties in the Trans‐Mexican volcanic system, Mexico.Crossref | GoogleScholarGoogle Scholar |
Chen M, Xie P, Janowiak JE, Arkin PA (2002) Global land precipitation: a 50-yr monthly analysis based on gauge observations. Journal of Hydrometeorology 3, 249–266.
| Global land precipitation: a 50-yr monthly analysis based on gauge observations.Crossref | GoogleScholarGoogle Scholar |
Cheng D, Rogan J, Schneider L, Cochrane M (2013) Evaluating MODIS active fire products in subtropical Yucatán forest. Remote Sensing Letters 4, 455–464.
| Evaluating MODIS active fire products in subtropical Yucatán forest.Crossref | GoogleScholarGoogle Scholar |
Comisión Nacional de Áreas Naturales Protegidas (CONANP) (2013) Áreas Naturales Protegidas Federales de México. Available at http://sig.conanp.gob.mx/website/interactivo/anps/ [Verified 16 May 2020]
Comisión Nacional de Áreas Naturales Protegidas (CONANP) (2014) Programa Nacional de Áreas Naturales Protegidas 2014–2018. Plan Nacional de Desarrollo 2013–2018. Gobierno de la República. México. Available at https://www.conanp.gob.mx/documentos/PNANP20142018.pdf [Verified 16 May 2020]
Comisión Nacional Forestal (CONAFOR) (2001) Programa estratégico forestal para México 2025. Publicación Especial de la Comisión Nacional Forestal. (Zapopan, Jalisco, México).
Comisión Nacional Forestal (CONAFOR) (2009) ‘Programa Nacional de Protección contra Incendios Forestales. Resultados 2008. Primera edición.’ (Zapopan, Jalisco, México).
Comisión Nacional Forestal (CONAFOR) (2010) Incendios forestales. Guía práctica para comunicadores. Secretaría de Medio Ambiente y Recursos Naturales (SEMARNAT). (Zapopan, Jalisco, México).
Comisión Nacional Forestal (CONAFOR) (2012) Zonificación forestal. Available at https://snigf.cnf.gob.mx/zonificacion-forestal/ [Verified 16 May 2020]
Comisión Nacional Forestal (CONAFOR) (2016) Reporte semanal de resultados de incendios forestales 2016. Programa Nacional de Prevención de Incendios Forestales/Centro Nacional de Control de Incendios Forestales-CONAFOR. (Zapopan, Jalisco, México).
Comisión Nacional para el Conocimiento y Uso de la Biodiversidad (CONABIO) (1998) Los incendios en México: un diagnóstico de su efecto en la diversidad biológica. Informe entregado a la SEMARNAT. Available at http://conabio.gob.mx/mapaservidor/incendios/modis/tablas2007/tablas2007/vegetacion.html [Verified 16 May 2020]
Costafreda-Aumedes AS, Comas C, Vega GC (2017) Human-caused fire occurrence modelling in perspective: a review. International Journal of Wildland Fire 26, 983–998.
| Human-caused fire occurrence modelling in perspective: a review.Crossref | GoogleScholarGoogle Scholar |
Fernandes K, Baethgen W, Bernardes S, DeFries R, DeWitt DG, Goddard L, Lavado W, Dong EL, Padoch C, Pinedo VM, Uriarte M (2011) North tropical Atlantic influence on western Amazon fire season variability. Geophysical Research Letters 38, L12701
| North tropical Atlantic influence on western Amazon fire season variability.Crossref | GoogleScholarGoogle Scholar |
Finney M (2005) The challenge of quantitative risk analysis for wildland fire. Forest Ecology and Management 211, 97–108.
| The challenge of quantitative risk analysis for wildland fire.Crossref | GoogleScholarGoogle Scholar |
Food and Agriculture Organization of the United Nations (FAO) (2007) ‘Situación de los bosques del Mundo.’ (FAO: Rome, Italy).
Food and Agriculture Organization of the United Nations (FAO) (2014) ‘El estado de los bosques del mundo. Potenciar los beneficios socioeconómicos de los bosques.’ (FAO: Rome, Italy).
Galván OL (2011) Impacto de la sequía meteorológica en la vegetación en distintas regiones climáticas de México (1982–2006). Masters thesis, Universidad Nacional Autónoma de México (UNAM), Mexico City. Available at 132.248.9.195/ptb2011/octubre/0674287/Index.html [Verified 16 May 2020]
Gómez PA, Kaus A, Jimenez OJ, Bainbridge D, Rorive VM (1993) Mexico. In ‘Sustainable agriculture and the environment in the humid tropics’. (Eds BJ Rice, JL Overton) pp. 483–548. (The National Academies Press: Washington, DC)
Huete A, Didan K, Miura T, Rodriguez EP, Gao X, Ferreira LG (2002) Overview of the radiometric and biophysical performance of the MODIS vegetation indices. Remote Sensing of Environment 83, 195–213.
| Overview of the radiometric and biophysical performance of the MODIS vegetation indices.Crossref | GoogleScholarGoogle Scholar |
IPCC (2012) ‘Managing the risks of extreme events and disasters to advance climate change adaptation.’ A special report of Working Groups I and II of the Intergovernmental Panel on Climate Change. (Eds CB Field, V Barros, TF Stocker, D Qin, DJ Dokken, KL Ebi, MD Mastrandrea, KJ Mach, GK Plattner, SK Allen, M Tignor, PM Midgley). (Cambridge University Press: Cambridge, UK).
Jolly WM, Cochrane MA, Freeborn PH, Holden ZA, Brown TJ, Williamson GJ, Bowman DM (2015) Climate-induced variations in global wildfire danger from 1979 to 2013. Nature Communications 6, 7537
| Climate-induced variations in global wildfire danger from 1979 to 2013.Crossref | GoogleScholarGoogle Scholar | 26172867PubMed |
Kirtman BP, Dughong M, Johnna MI (2014) The North American Multimodel Ensemble: Phase-1 seasonal-to-interannual prediction; Phase-2 toward developing intraseasonal prediction. Bulletin of the American Meteorological Society 95, 585–601.
| The North American Multimodel Ensemble: Phase-1 seasonal-to-interannual prediction; Phase-2 toward developing intraseasonal prediction.Crossref | GoogleScholarGoogle Scholar |
Krieger DJ (2001) The economic value of forest ecosystem services: a review. The Wilderness Society, Washington DC. Available at https://www.sierraforestlegacy.org/Resources/Conservation/FireForestEcology/ForestEconomics/EcosystemServices.pdf [Verified 18 May 2020]
Magaña RV (Ed.) (1999) ‘Los impactos de El Niño en México.’ (Centro de Ciencias de la Atmósfera, Universidad Nacional Autónoma de México, Secretaría de Gobernación: México City). Available at http://centro.paot.org.mx/index.php/porinstituciones/otros-organismos/73-varios/745-los-impactos-de-el-nino-en-mexico [Verified 18 May 2020]
Magaña RV, Neri C (2012) El reto de la sequía en México. Revista Mexicana de Sociologia 1, 12–14.
McKee TB, Doesken NJ, Kleist J (1993) The relationship of drought frequency and duration to time scales. In ‘Eighth conference on applied climatology’, 17–22 January 1993, Anaheim, CA. (American Meteorological Society: Boston, MA, USA).
McKee TB, Doesken NJ, Kleist J (1995) Drought monitoring with multiple time scales. In ‘Proceedings of the ninth conference on applied climatology’, 15–20 January 1995, Dallas, TX. (American Meteorological Society: Boston, MA, USA).
NASA Fire Information for Resource Management (FIRMS) Active fire data: MODIS Collection 6 NRT – MODIS Collection 6 NRT Hotspot/Active Fire Detections MCD14DL. Available at https://earthdata.nasa.gov/earth-observation-data/near-real-time/firms [Verified 18 May 2020]
Neri C, Magaña RV (2016) Estimation of vulnerability and risk to meteorological drought in Mexico. Weather, Climate, and Society 8, 95–110.
| Estimation of vulnerability and risk to meteorological drought in Mexico.Crossref | GoogleScholarGoogle Scholar |
NOAA-GFDL (2014) GFDL-CM2p1 model output prepared for CMIP5, served by ESGF. World Data Center for Climate (WDCC) at Deutsches Klimarechenzentrum (DKRZ), Hamburg Germany. Available at https://cera-www.dkrz.de/WDCC/ui/cerasearch/entry?acronym=NGG2 [Verified 18 May 2020]
Nobre CA, De Simone BL (2009) ‘Tipping points’ for the Amazon forest. Current Opinion in Environmental Sustainability 1, 28–36.
| ‘Tipping points’ for the Amazon forest.Crossref | GoogleScholarGoogle Scholar |
North American Regional Reanalysis (NARR) (2004) A long-term, consistent, high-resolution climate dataset for the North American domain, as a major improvement upon the earlier global reanalysis datasets in both resolution and accuracy. Fedor Mesingeret al. [BAMS.]. Available at https://psl.noaa.gov/data/gridded/data.narr.html [Verified 20 May 2020]
Pérez VG, Márquez LM, Cortés OA, Salmerón MM (2013) Análisis espacio-temporal de la ocurrencia de incendios forestales en Durango, México. Madera y Bosques 19, 37–58.
| Análisis espacio-temporal de la ocurrencia de incendios forestales en Durango, México.Crossref | GoogleScholarGoogle Scholar |
Pompa-Garcia GM, Camarero JJ, Rodríguez-Trejo DA, Vega-Nieva DJ (2018) Drought and spatiotemporal variability of forest fires across Mexico. Chinese Geographical Science 28, 25–37.
| Drought and spatiotemporal variability of forest fires across Mexico.Crossref | GoogleScholarGoogle Scholar |
Pyrke AF, Marsden SJB (2005) Fire-attributes categories, fire sensitivity, and flammability of Tasmanian vegetation communities. Tasforests 16, 35–46.
Ressl R, Cruz I (2012) Detección y monitoreo de incendios forestales mediante imágenes de satélite. Biodiversitas 100, 12–13.
Rodríguez-Trejo DA, Fulé PZ (2003) Fire ecology of Mexican pines and a fire management proposal. International Journal of Wildland Fire 12, 23–37.
| Fire ecology of Mexican pines and a fire management proposal.Crossref | GoogleScholarGoogle Scholar |
Rouse JW, Haas RH, Schell JA, Deering DW (1974) Monitoring vegetation systems in the Great Plains with ERTS. In ‘Proceedings, third earth resources technology satellite-1 symposium’, 10–14 December 1973, Washington DC. (Eds CF Stanley, PM Enrico, AB Margaret) pp. 309–317. (Scientific and Technical Information Office, National Aeronautics and Space Administration: Washington DC). Available at https://ntrs.nasa.gov/archive/nasa/casi.ntrs.nasa.gov/19740022614.pdf [Verified 18 May 2020].
Vose JM, Clark JS, Luce CH, Patel-Weynand T (Eds) (2016) Effects of drought on forests and rangelands in the United States: a comprehensive science synthesis. USDA Forest Service, General Technical Report WO-93b. (Washington, DC, USA)
Wildlife Conservation Society Center for International Earth Science Information Network (WCS-CIESIN) (2005) Last of the wild Project, version 2, 2005 (LWP-2): Global Human Influence Index (HII) dataset (geographic). Available at http://sedac.ciesin.columbia.edu/data/set/wildareas-v2-human-influence-index-geographic [Verified 18 May 2020]
World Bank (2017) World development indicators. (Washington, DC, USA). Available at http://data.worldbank.org/data-catalog/world-development-indicators [Verified 18 May 2020]
Zúñiga-Vásquez VJM, Cisneros GD, Pompa GM, Rodríguez TDA, Pérez VG (2017a) Spatial modeling of forest fires in Mexico: an integration of two data sources. Bosque 38, 563–574.
| Spatial modeling of forest fires in Mexico: an integration of two data sources.Crossref | GoogleScholarGoogle Scholar |
Zúñiga-Vásquez VJM, Cisneros GD, Pompa GM (2017b) Drought regulates the burned forest areas in Mexico: the case of 2011, a record year. Geocarto International 34, 1–14.
| Drought regulates the burned forest areas in Mexico: the case of 2011, a record year.Crossref | GoogleScholarGoogle Scholar |