Introduction to the Australian Fire Danger Rating System†
Jennifer J. Hollis A C * , Stuart Matthews A D , Paul Fox-Hughes
A
B
C Present address:
D Present address:
E Present address:
F Present address:
Abstract
Fire danger rating systems are used daily across Australia to support fire management operations and communications to the general public regarding potential fire danger.
In this paper, we introduce the Australian Fire Danger Rating System (AFDRS), providing a short historical account of fire danger rating in Australia as well as the requirements for an improved forecast system.
The AFDRS combines nationally consistent, spatially explicit fuel information with forecast weather and advanced fire behaviour models and knowledge to produce locally relevant ratings of fire behaviour potential.
A well-defined framework is essential for categorising and defining fire danger based on operational response, the potential for impact and observable characteristics of fire incidents. The AFDRS is modular, supporting continuous and incremental improvements and allowing upgrades to components in response to new science.
The AFDRS provides a new method to estimate fire danger based on the best available fire behaviour models, leading to potentially significant improvements in the way fire danger is calculated, forecast and interpreted.
The Australian Fire Danger Rating System was implemented in 2022, the most significant change to fire danger forecasting in Australia in more than 50 years.
Keywords: bushfire risk, bushfire hazard, fire behaviour index, fire danger index, fire management, forecast system, McArthur, suppression difficulty.
Introduction
Over the last two decades, the occurrence of significant catastrophic wildfire (bushfire) events has led to large numbers of human fatalities, significant asset losses and long term economic and social impacts in Australia (Cruz et al. 2012; Nolan et al. 2021; Mills et al. 2022) and disparate places including Chile (Gómez-González et al. 2018), Portugal (Castellnou et al. 2018), Greece (Xanthopoulos and Athanasiou 2019) and the United States of America (Nauslar et al. 2018). Recent variations to fire regimes including increased fire weather severity, frequency and extent have been linked to changes in climate and land use patterns in different regions around the world (Clarke and Evans 2018; Dowdy 2018; Syphard et al. 2019). With these changes, the need to minimise harm and severity of significant fire events has intensified, and as such, the ability to ensure operational preparedness and public awareness of potential fire threats and impacts has equally grown in importance. Systems for forecasting fire danger are an essential tool for addressing this need by quantifying the effect of environmental and anthropogenic factors on the potential for fire propagation, difficulty of control, ignition potential and impacts on human and environment values (McArthur 1958, 1977a; Chandler et al. 1983; San-Miguel-Ayanz et al. 2003;, Di Giuseppe et al. 2016).
Systems for rating fire danger have been a key component of fire management around the world for more than a century (Hardy and Hardy 2007). Largely developed to aid decision making for fire suppression operations, they are also a highly valuable tool in communicating fire danger to the community, increasing public awareness and triggering notifications regarding potential threats. The complexity of systems has ranged from simple dryness indexes (Nesterov 1949) to more complete systems such as the National Fire Danger Rating System in the United States (NFDRS; Deeming et al. 1972; Cohen and Deeming 1985) and the Canadian Forest Fire Danger Rating System (CFFDRS; Stocks et al. 1989; Taylor and Alexander 2006). The Canadian Forest Fire Weather Index (FWI; Van Wagner 1987) element of the CFFDRS has design features with potential for application beyond local fuel types. As a result, the system has been either tested or adapted for application in other countries such as Argentina, Chile, Fiji, Mexico, New Zealand (Fogarty et al. 1998), Venezuela and some parts of the United States (e.g. Alaska) and Europe (e.g. Portugal, Spain, Greece; Dimitrakopoulos et al. 2011). Recently, fire danger has been calculated at large regional and global scales (Di Giuseppe et al. 2016; Pettinari and Chuvieco 2017). Reviews of the structure and skill of fire danger rating systems have been presented by Chandler et al. (1983), Viegas et al. (1999), San-Miguel-Ayanz et al. (2003), Fujioka et al. (2009), de Groot et al. (2015) and Zacharakis and Tsihrintzis (2023).
Evolution of the Australian Fire Danger Rating project
In 2014, the Australia and New Zealand Emergency Management Committee (ANZEMC) and Ministerial Council for Police and Emergency Management (MCPEM) recognised that a thorough review of the approach to defining and calculating fire danger rating was required. A collaborative study was subsequently conducted in 2015 involving scientists and representatives from fire and land management agencies from all Australian jurisdictions. The study produced detailed requirements for building and implementing a new fire danger rating system that included six factors: weather, fuels, fire behaviour, ignition likelihood, suppression capacity and fire impacts (Cube Group 2015). A phased approach to development was adopted by ANZEMC to manage the risk and cost of implementing a new system with large potential impacts on community and fire management operations.
The first phase of the process began in 2017 with development of the Australian Fire Danger Rating System in a collaborative effort by the New South Wales Rural Fire Service and Bureau of Meteorology, together with representatives from Australian fire agencies. During the southern fire season (October 2017–March 2018), a live trial evaluation of a Research Prototype version (AFDRSRP) of the AFDRS was conducted to assess performance and reliability. The live trial demonstrated that a new national system was achievable and could provide clear community, economic and operational benefits (Grootemaat et al. 2024). The AFDRSRP was subsequently endorsed by the Australian Government’s MCPEM and moved to an implementation phase (Ministerial Council for Police and Emergency Management 2018) where additional practical components and local systems integration could be completed.
The series of five papers presented in this Special Section provide the background to approaches, components, performance and climatology of the Australian Fire Danger Rating System:
In this paper, we introduce the Australian Fire Danger Rating System (AFDRS), providing a short history of fire danger rating in Australia together with requirements to make advancements. We describe the development, design principles and supporting framework of the AFDRS;
Hollis et al. (2024) identify and present a framework for defining and categorising fire danger;
Kenny et al. (2024) describe a system for implementing fire behaviour calculations to forecast fire danger;
Grootemaat et al. (2024) evaluate the performance of the AFDRS Research Prototype; and
Sauvage et al. (2024) present a climatology of the AFDRS Research Prototype.
This collective work provided the foundation for operational implementation of the AFDRS throughout Australia in September 2022; however, it is important to note that some modifications have since been made to the system that were necessary to improve performance and accuracy and to reflect public-facing Fire Danger Rating requirements.
Fire danger rating in Australia
In Australia, fire danger ratings have evolved from early hazard stick assessments in the 1930s through to the McArthur-based ratings in operational use up till 2022 when systems were replaced by the AFDRS (Fig. 1).
Timeline of key publications, reports and advances in fire danger rating in Australia, 1935–2022. (WA, Western Australia; SA, South Australia; FDR, Fire Danger Rating).
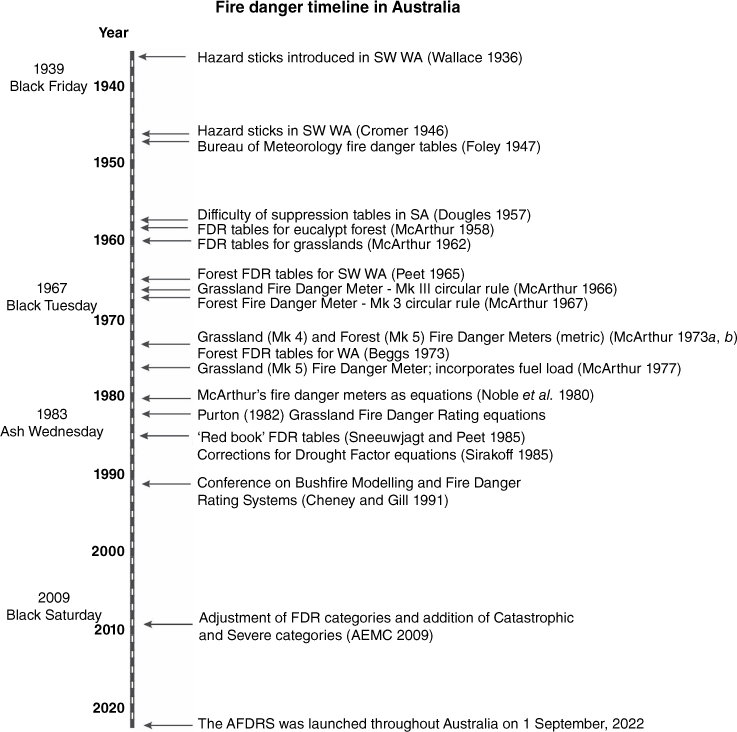
The earliest fire danger rating systems in Australia were largely based on weather indices, without accounting for fuel factors (Cheney 1991). In the south-west of Western Australia, the moisture content of dowels or hazard sticks (Gisborne 1933) was used to indicate the potential of fire to start and spread (Wallace 1936; Cromer 1946). Similar systems using fire weather stations and fire hazard forecasts were also established in Tasmania and throughout south-eastern Australia (Foley 1947). Difficulty of suppression tables were later developed in South Australia in which five classes of suppression difficulty were related to the type and amount of fuel, the fire hazard (based on hazard stick moisture) and wind velocity (Douglas 1957). The use of hazard sticks for the assessment of fuel moisture was later dropped in favour of using only meteorological elements (McArthur and Luke 1963).
In the 1950s and 1960s, more comprehensive fire danger ratings were produced based on the predicted rate of spread and the difficulty of suppression of fires burning in grasslands (McArthur 1960) and dry sclerophyll forests (McArthur 1958, 1967) from the analysis of a large number of experimental fires, supplemented with data from high-intensity wildfires (Cheney 1968).
Difficulty-of-suppression tables for forests were originally presented (McArthur 1958) based on a rating of ‘difficulty experienced in suppressing the fire perimeter’ against wind speed and relative humidity (for a set range of temperatures) within 89 experimental fires. Ratings were determined on a six-point scale where: Nil = fires will not spread; Low = low difficulty of suppression; Moderate = moderate difficulty of suppression; High = high difficulty of suppression; Very High = very high difficulty of suppression, and Extreme = extreme difficulty of suppression.
Each difficulty of suppression rating primarily took fuel moisture and wind velocity into account as well as spotting distance and potential fire intensity and atmospheric instability (McArthur 1958).
Suppression difficulty classes were later redefined as fire danger classes and the scale of fire danger was set between 1 and 100, where an index of 100 represented hypothetical worst-case summer conditions of: temperature 35°C; relative humidity 10%, mean wind speed 45 km h−1, coupled with average summer drought. Classes were then related to meteorological variables alone so that fire danger ratings could be based on weather forecasts. Fire danger rating tables for grasslands were also developed (McArthur 1960), and evolved over several years to incorporate the effect of grassland curing and wind speed (McArthur 1966, 1973b) and later fuel load (Purton 1982); however, the fuel load function was not consistently applied across Australia.
Two systems, the Forest Fire Danger Index (FFDI) and the Grassland Fire Danger Index (GFDI) were published as either linear or circular slide rules (Fig. 2) with revisions to incorporate improvements in fire spread predictions (McArthur 1966, 1967, 1977b). There was little documentation to accompany developments (Cheney 1988). Equations were fitted to the McArthur slide rules (Noble et al. 1980; Purton 1982; Sirakoff 1985; Griffiths 1999) to allow calculation using computers. These systems for fire danger rating were designed for general forecasting purposes and for local application by fire authorities for fire behaviour prediction on the fireline and as a rough fire suppression guide (Cheney 1988). McArthur (1977a) argued that the Australian Forest Fire Danger system would be suitable for global application; however, use has remained focused within Australia.
Tools used to determine fire danger rating in Australia include: (a) slide rule for Grassland fuels (McArthur 1977b), and circular meters for (b) Grassland (McArthur 1973b) and (c) Forest (McArthur 1973a) fuel types.
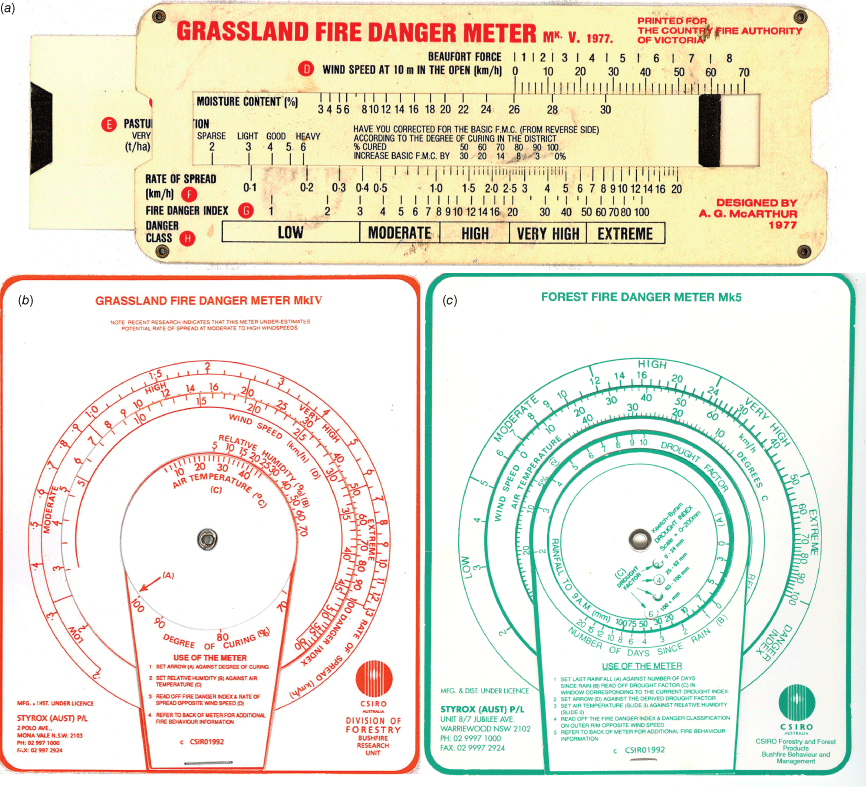
McArthur’s ratings were developed to assist fire managers to answer the following questions (McArthur 1958):
> What rates of spread and fire intensity could be expected for fires under forecast conditions and is there potential for short- or long-range spotting?
> What fire area, perimeter and rates of spread could be expected at various times after ignition?
> How difficult would a fire be to control and would mechanical equipment be required or could it be suppressed effectively by a standard initial attack crew?
> Would a fire be limited to surface fuels or is there potential for crown fires? Is there a possibility of a fire ‘blowing up’?
The forecasts have also been used operationally to represent a measure of expected fire load in order to plan pre-suppression activities (e.g. detection services, crew placement and park closures).
During the same time period, an alternative approach to fire danger rating was developed specific to jarrah and karri forests in Western Australia (Peet 1965; Sneeuwjagt and Peet 1998). In these forests, five fire danger classes were categorised against rate of head-fire spread, similarly to the spread rates predicted by McArthur’s Forest Fire Danger Model (Cheney 1988). Equations were later developed to allow computation (Beck 1995).
After three decades of use, the systems appeared to provide a sufficient guide to enact appropriate levels of preparedness and public warning (Cheney et al. 1990). However, growing intensity and severity of recent bushfire experiences across the country prompted Australian fire managers to recognise that the McArthur system, practices and processes to support effective community safety outcomes no longer matched the increasing levels of risk and expectations. The Black Saturday fire events in 2009 in Victoria resulted in losses at an unprecedented scale, tragically burning over 450 000 ha and resulting in 173 human fatalities (Teague et al. 2010). At this time, fire management authorities confirmed the McArthur system was being applied beyond the original design (Fogarty et al. 2010). In response, the National Bushfire Warnings Taskforce through the Australian Emergency Management Committee implemented revisions incorporating changes to the index scale and categories for both forest and grass fuels. This specifically included adding an additional fire danger rating category (Catastrophic/Code Red) beyond the existing highest level of Extreme, adjusting the existing fire danger ratings to correspond to higher Fire Danger Index values and developing a revised fire severity scale for use in bushfire warnings based on new fire danger ratings (Australian Emergency Management Committee – National Bushfire Warnings Taskforce 2009).
At the same time, research was initiated to better understand the links between fire weather and house loss (Blanchi et al. 2012), to investigate consequence-based approaches to ratings (Penman et al. 2015) and to construct a climatology of fire danger events (Kepert et al. 2012). However, none of this work led to changes in the rating system because it was not possible to make adjustments to the mechanics of the McArthur indices to include new knowledge. Fogarty et al. (2010) suggested that the scientific variables and computational methods that underpinned the system required significant review and consideration, specifically drawing on contemporary understanding of physical and social aspects of fire science, and associated communication implications, all this requiring a concerted research effort to fill critical knowledge gaps.
Apart from the revisions made in 2009, the McArthur system remained largely based on the two original rating scales developed in the 1950 and 1960s.
Operational insights from using the McArthur fire danger rating system
A recognised shortcoming of the McArthur-based fire danger rating system was the inability to make use of contemporary fire behaviour knowledge developed since the pioneering work of A.G. McArthur (Fogarty et al. 2010; Cube Group 2015). This includes new fire spread models (e.g. Cheney et al. 2012; Cruz et al. 2013; Anderson et al. 2015) and other processes known to affect fire danger such as wind changes (Hungerford et al. 1991; Huang and Mills 2006), spotting (Gould et al. 2007) and coupling of fires to the atmosphere, commonly assessed through measures of atmospheric instability (Mills and McCaw 2010). Another limitation of the system was its inability to provide location-specific advice about potential fires (Kilinc et al. 2013). Although provision of general information over a broad area was appropriate in the past (Cheney and Gould 1995), with the availability of gridded weather forecasts, there was a desire for more detailed fire danger forecasts. A key gap that prevented local application of the FFDI and GFDI was their inability to represent fire danger in vegetation types that are structurally dissimilar to forest or grass, key examples being shrublands (Anderson et al. 2015) and spinifex (Burrows et al. 2018) vegetation.
The form of the equations fitted by Noble et al. (1980) makes the McArthur fire danger forecasts very sensitive to uncertainty in both inputs (weather and curing) and the models used to calculate danger (Cruz and Alexander 2013). Cheney and Gould (1995) found that forecasts can be expected to vary by at least one fire danger rating category and greater uncertainty is expected at more elevated fire danger (Cheney 1988). Attempts to improve the accuracy of the rating system were also hampered by the lack of clear, standardised definitions of what the rating classes mean, making it difficult to assess the performance of the system over time beyond general or anecdotal observations. The McArthur system was also unable to incorporate lessons from more recent extreme events (Fogarty et al. 2010).
Originally intended to primarily assess suppression difficulty, fire danger ratings were used to make a wide range of decisions including informing ignition management controls (e.g. Total Fire Bans), setting preparedness levels and informing the community of the need to prepare for potential fires. Investigation of the impacts of fires on community loss found that although most house losses occur at elevated fire danger levels (Blanchi et al. 2010), the McArthur system was often a poor predictor of the scale of losses (Harris et al. 2012; Kilinc et al. 2013). A 2019 survey showed that understanding and engagement with the McArthur rating system was also poor (O’Donohue et al. 2019). In order to address these issues, the scope of an improved system needed to also consider ignition likelihood, suppression capacity and potential fire impacts.
Requirements for a new fire danger rating system
Developing an appropriate framework for fire danger rating for the wide variety of vegetation types in Australia that incorporates current scientific knowledge, the diversity of operational standards across jurisdictions, fire suppression requirements and community expectations is complex and challenging. Ultimately, the system developed needs to support a fire manager’s ability to make improved decisions in response to fire danger based on fire risk, likely fire behaviour and many other important factors such as allocation of burn permits and resource constraints (Luke and McArthur 1978). Roy Headley (1943), as Director of Fire Control for the United States Forest Service (1919–1941) stated ‘one of the major needs is for a system that will allow a man in charge of a going fire to be less of a gambler and more of a manager’.
During his time as fire researcher with the United States Forest Service, RM Nelson (research forester, US Forest Service, Southern Forest Experiment Station, Asheville, NC; Nelson 1955) stated, ‘I do not want to leave the impression that I think a good system of danger measurement is the answer to all fire control and management problems. It can be a guide, and a very useful one, but it can never take the place of cool, calculating, and experienced judgement’. In this way, the usefulness of the system will always be limited by the capability and experience of operational fire managers.
In Australia, McArthur (1977a) in a similar way supported the need for experienced judgement, stating ‘a fire danger rating system is not intended to take the place of experience and judgement or the ability of a fire boss to ‘size up’ a fire situation and estimate fire behaviour’.
System requirements (Box 1) were used to design a modern fire danger rating system based on literature (e.g. Fogarty et al. 2010; Cube Group 2015; National Wildfire Coordinating Group 2019) and through consultation with stakeholders including fire scientists and operational fire managers (Hollis et al. 2024).
Many of the limitations identified stemmed from the FFDI and GFDI having both a fixed structure and vaguely defined meaning, which prevented assessment and improvement. To address these limitations and to allow assessment of system performance, we required the AFDRS to use clearly defined ratings based on operational response, potential for impact and observable characteristics of fire incidents. The system also needed to be designed in a modular fashion to support continuous improvement and to allow upgrades to components in response to new science without needing to rebuild the entire system (Countryman 1966; Taylor and Alexander 2006). A modular approach would also support incremental investment to address weaknesses in a prioritised way.
To meet these requirements, the most suitable approach is a system that is based on fire behaviour calculations rather than fire frequency or index climatology (Alexander 2010). Using fire behaviour calculations also allows use of vegetation-specific models to provide locally relevant information. The system should include the ability to capture related measures that affect fire danger such as wind changes, spotting and atmospheric instability. The system also needs to use the best available weather and fuel information to provide forecasts that are accurate and suitable for use at a variety of temporal and spatial scales.
To support rigorous decision making and clear communication to fire managers and the general public, the system needed to be suitable for consistent and standardised use across the various Australian states and territories without requiring ad hoc local adjustments. Development of the AFDRS and dissemination of fire danger ratings and warnings needed to consider how to communicate messages to users in a clear and actionable way.
One of the strengths of the McArthur system was its simplicity, resulting in it being used at a variety of levels with ease of computation and interpretation. Recognising different users require varying amounts of information and detail, the AFDRS needed to be simple to use but make detail available when needed (Van Wagner 1971; Sharples et al. 2009). The scope of the system also needed to be broad enough to cover more aspects of fire management and include fire behaviour, ignition likelihood, suppression capacity and potential impacts to support improved decision-making.
Overview of the Australian Fire Danger Rating System
A research prototype system of fire danger rating (AFDRSRP) based on fire behaviour calculations was developed to meet the requirements outlined in Box 1. The AFDRSRP was used as the foundation to build the AFDRS that was implemented in 2022, which required development of additional system architecture including Fuel State Editor and Seasonal Outlook components.
In the AFDRSRP, fire danger is determined using established forward rate of spread models (Cruz et al. 2015), together with forecast weather at hourly intervals on a 1.5 km grid across Australia (Bureau of Meteorology 2015) to perform calculations of forward rate of fire spread and fireline intensity from which fire danger is determined (Fig. 3). Eight models of rate of forward spread were used as recommended by Cruz et al. (2015), namely those specific to eucalypt native forest (Cheney et al. 2012), grassland (Cheney et al. 1998), spinifex (Burrows et al. 2017; Burrows et al. 2018), pine plantation (Cruz et al. 2008), northern grassland (savanna woodland) (Cheney and Sullivan 2008), mallee–heath (Cruz et al. 2013), shrubland (Anderson et al. 2015) and buttongrass (Marsden-Smedley and Catchpole 1995) fuel types. These major fuel types were subdivided on the basis of vegetation structure (e.g. wet and dry eucalypt forest) and classified into several hundred individual fuel types, to differentiate local variation in fuel attributes. The system assigned model fuel inputs for each spatial grid cell based on fuel attributes (e.g. fire history) (Kenny et al. 2024).
For operational applications, the rate of spread outputs were used to calculate fire behaviour metrics that were then applied to calculate a Fire Behaviour Index and to assign one of up to six fire danger rating categories that were defined for each of the eight fuel types (Hollis et al. 2024). Transition points between each category were identified based on transitions in fire behaviour that result in application of different fire management strategies or associated with variation in potential consequences and impacts. Each transition point was assigned a threshold value depending on fuel type. In some fuel types, transition points were adequately captured by processes within the fire behaviour model (as was the case for semi-arid mallee–heath and spinifex fuel types). However, where appropriate variables and values were not part of fire spread models, we applied fireline intensity (Byram 1959) to allocate transition points between categories (i.e. in dry eucalypt forest, grassland, exotic pine plantation, northern grasslands (savanna) and temperate shrubland fuel types).
Definitions describing possible fire behaviour, prescribed burning potential, suppression and containment, and potential consequences of a fire (Hollis et al. 2024) were assigned to each category. Fire danger rating categories were summarised using unique descriptions of Relevance for each fuel type. The example provided in Table 1 pertains to forest fuel types.
Fire danger category | ||||||
---|---|---|---|---|---|---|
1 | 2 | 3 | 4 | 5 | 6 | |
![]() | ![]() | ![]() | ![]() | ![]() | ![]() | |
Mostly self-extinguishing, trouble-free fires | Typical prescribed burning conditions | Most bushfires in this category | Initial attack success critical to prevent large fire development | Increased likelihood of community loss and significant consequences | High probability of loss of life and property | |
Fires are likely to be mostly self-sustaining | Fires typically suppressed with offensive fire management strategies (i.e. direct, parallel or indirect attack) | Increasing focus on defensive suppression strategies | Conditions limit strategic suppression options | Elevated risk to firefighter safety | ||
Fires are generally easy to suppress and contain | Transition to medium distance spotting | Elevated risk to firefighter safety | Initial attack success critical to prevent large fire development | |||
Aerial suppression less likely to be effective at controlling fire | Initial attack success critical to prevent large fire development | Conditions limit strategic suppression options. Wind speed and limited visibility may ground some aviation resources | ||||
Transition to long distance spotting |
Three Red Flag warnings were also defined to identify conditions where fires were likely to be affected by a wind change, long-distance spotting or atmospheric instability. The Red Flag warnings are provided for each forecast district (Fire Weather Districts are established based on shared common meteorological characteristics; Bureau of Meteorology 2011, 2023). The Red Flag warnings were defined as:
> Wind change: significant wind changes are identified when the Wind Change Danger Index is above 40 (Huang and Mills 2006) over at least 10% of a Fire Weather District.
> Spotting: 90th percentile daily maximum spotting distance calculated using the model of Gould et al. (2007) over a Fire Weather District. A threshold for significant spotting was not identified to provide a yes/no flag.
> Instability: daily maximum C-Haines index (Mills and McCaw 2010; Dowdy and Pepler 2018) above the 95th percentile climatological value covering at least 10% of a Fire Weather District indicates significant potential instability.
System outputs were summarised for the AFDRSRP into daily rating tables and maps by calculating the 90th percentile rating of the daily maximum rating in each Fire Weather District. Daily rating tables, similar to those currently produced by the Bureau of Meteorology, were provided on a static website summarising daily maximum fire danger rating and Red Flag warnings for each Fire Weather District.
Components of the Australian Fire Danger Rating System. Details of Fire Behaviour Index and Categorisation are presented in Table 1.
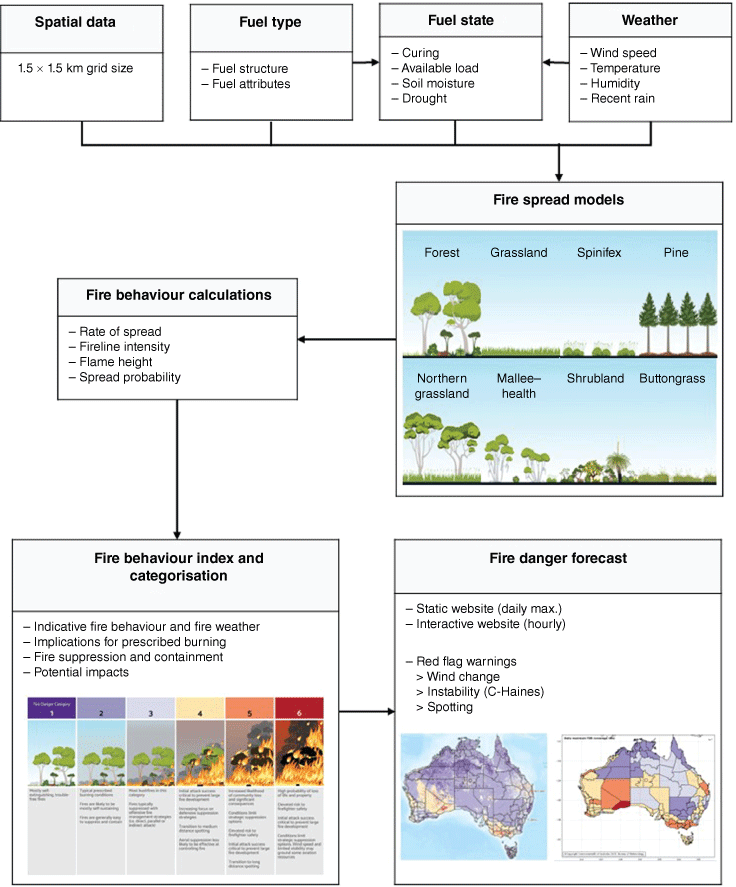
The static website consisted of a national map of daily maximum fire danger rating by Fire Weather District (Fig. 4), as well as state-based maps of daily maximum fire danger rating displayed at the Fire Weather District scale (Fig. 5). An interactive website displayed spatial hourly forecasts of fire danger rating, with options to view the time series (up to 3 days’ forecast) at any location as well as additional layers showing input values (e.g. weather parameters, fuel type and time since fire), incident markers, topography and calculated outputs at the 1.5 km grid scale (Fig. 6). The use of numbers and colours to label rating categories was chosen to allow evaluation of the AFDRSRP.
Welcome page for the AFDRS showing the national daily ratings for each Fire Weather District.
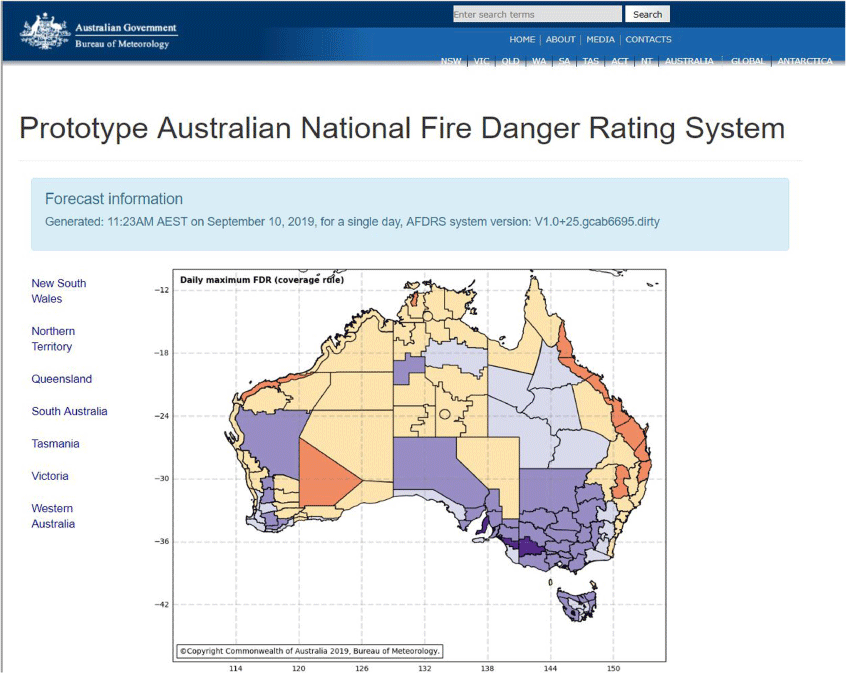
Screen shot of daily ratings for each Fire Weather District within Queensland showing (a) the daily maximum AFDRS rating on the 1.5 km spatial grid (as shown in Fig. 6); (b) the daily maximum Fire Danger Index (FDI) on the 1.5 km spatial grid; and (c) the daily maximum AFDRS rating for each Fire Weather District. For each Fire Weather District, the table provides the location identifier (AAC), forecast daily maximum AFDRS rating and red flag warnings for C-Haines (yes/no), spotting distance and wind change (yes/no) based on the Wind Change Danger Index (WCDI).
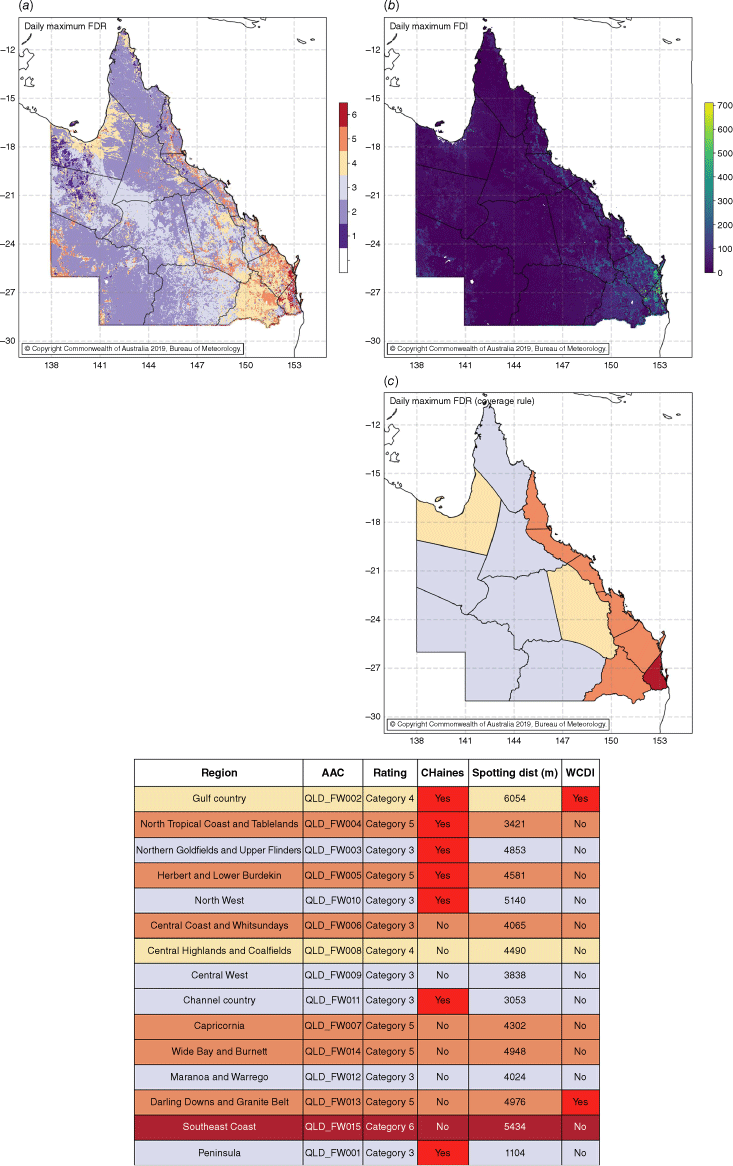
Screen shot of the welcome page for the interactive AFDRS showing hourly fire danger at the 1.5 km grid scale across Australia. Diamond icons indicate live, fire incidents based on the Australian Warning System (https://www.australianwarningsystem.com.au/) where white: no warning level, blue: Advice, yellow: Watch and Act, and red: Emergency Warning.
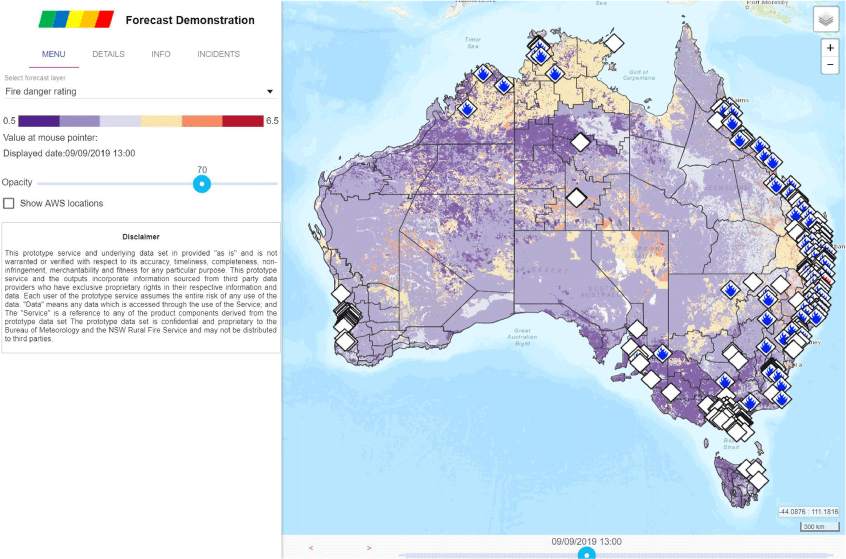
During the 2017–2018 southern fire season in Australia, a live trial was completed to assess the performance and reliability of the AFDRSRP. Seventy-one participants representing fire and land management agencies from all Australian jurisdictions participated in the live trial, completing a total of 265 observation reports (Grootemaat et al. 2024). The dataset was supplemented by 72 case study reports to expand conditions to also represent fires of significant consequence. Participants documented information from live incidents including descriptions of the fire behaviour, suppression or containment response and fire impacts. Each incident was then assigned to an observed rating category based on expert judgement using definitions for each fire danger rating category. Observed ratings were then compared with forecast ratings as calculated by the AFDRSRP, with participants identifying potential reasons for any inconsistencies in the forecast. The performance of the AFDRSRP was subsequently compared with that of the McArthur system. The AFDRSRP was found to accurately forecast fire danger 56% of the time compared with 43% by the McArthur system. The live trial highlighted a tendency for the AFDRSRP to overpredict rather than underpredict fire danger (Grootemaat et al. 2024).
The system architecture for the AFDRS is based on four modules: (i) a fuel state editor (an interactive tool for capture, upload and approval of the fuel inputs); (ii) fire danger calculations (implementation of the fire behaviour models and forecast calculations); (iii) a fire danger viewer (interactive visualisation of the weather and fire danger forecasts, including Red Flags); and (iv) seasonal outlooks (visualisation products of seasonal forecasts of fire danger). Work has also been under way to develop and evaluate additional risk products that complement the Fire Behaviour Index in the form of indices for (Fire) Ignition, Suppression and Impacts (FISI Indices) (Jenkins et al. 2021, 2022).
Categories of Fire Danger Rating for the public-facing system were also developed to include lessons from research findings for improved communication of fire danger to the community (Tippett et al. 2018), integration of findings from a recent nationwide survey (Metrix 2019) and consultation with stakeholders that make use of the fire danger rating system to inform decision-making. During implementation of the AFDRS, categories for the Operational Decision-making Framework were linked to the Public Facing Framework based on the Fire Behaviour Index to enable forecasting suitable for the general public.
Concluding remarks
The AFDRS builds on the previous fire danger systems used in Australia and has been shown to lead to significant improvements in the way fire danger is calculated, forecast and interpreted. By using forecast weather and spatially explicit fuel layers to yield projections of fire behaviour and fire danger, the approach taken enables categorisation with clearly defined thresholds that signal important changes in operational response and potential for impacts. Through a nationally consistent fuel layer linked to eight fire spread models, the AFDRS also encodes a climatology that reflects regional variations occurring where vegetation types are finely adapted to the climate of a particular location. The AFDRS has a modular framework, which allows for continuous and incremental improvement of its components in response to new science without needing to rebuild the entire system. Using standardised definitions of fire danger categories, the framework also enables the performance of the AFDRS to be measured beyond general or anecdotal observations.
Through the live trial evaluation of the AFDRSRP, the system has demonstrated capacity to result in significant improvements in forecasting fire danger, specifically in areas of fire weather prediction and fire behaviour potential, and in understanding potential impacts on human life, assets and the environment. The AFDRS will strengthen the ability of fire authorities to accurately communicate bushfire risk to the community, enhance operational readiness and preparedness, and contribute to risk management prevention (including input into building standards and planning controls).
It is important to note that any improvements to fire danger rating in Australia must be considered in the context of intended applications. The AFDRS will equip fire managers with access to improved fire danger forecasting and related information but it cannot replace the need to also have a depth of experience and judgement (McArthur 1977a). The ability of the AFDRS to ensure operational preparedness and public awareness of potential fire threats and impacts is highly dependent on adequate training of fire practitioners as well as suitable resourcing and tools. Likewise, a national campaign to inform and equip the Australian community is also essential for the successful implementation of the AFDRS.
At the time of writing, the AFDRS has been functioning operationally for over 1 year, which is the most significant change to fire danger forecasting in Australia in more than 50 years. The AFDRS modular framework enables continuous improvement and so the AFDRS Project Team are currently focused on reviewing performance and coordinating issue resolution and improvements (Australian Fire Danger Rating System 2023). During this time, models used to forecast the probabilities of suppression success at initial attack, and the probability of a fire impacting houses and fire ignitions have also been developed and their performance evaluated (Jenkins et al. 2021). These indices will provide additional indicators and measures to support fire management decision making.
Data availability
Data sharing is not applicable as no new data were generated or analysed during this study.
Declaration of funding
The AFDRS was funded by grants from the Australian Government’s National Emergency Management Projects programme and the NSW Rural Fire Service’s Bush Fire Risk Mitigation and Resilience Programme. The project also received considerable in-kind support from the Bureau of Meteorology.
Acknowledgements
The development of the AFDRS relied on the expertise and participation of many fire managers and researchers and we thank those who were part of early project planning, development and support, particularly from the AFAC (Australasian Fire and Emergency Service Authorities Council) Project Office. We want to thank in particular the many operational staff and researchers who contributed to the design and testing of the AFDRSRP. We also thank the Associate Editor for the Special Section and two anonymous reviewers for their contributions towards improving our manuscript.
References
Anderson WR, Cruz MG, Fernandes PM, McCaw L, Vega JA, Bradstock RA, Fogarty L, Gould J, McCarthy G, Marsden-Smedley JB, Matthews S, Mattingley G, Pearce HG, van Wilgen BW (2015) A generic, empirical-based model for predicting rate of fire spread in shrublands. International Journal of Wildland Fire 24, 443-460.
| Crossref | Google Scholar |
Beck J (1995) Equations for the forest fire behaviour tables for Western Australia. CALMScience 1, 325-348.
| Google Scholar |
Blanchi R, Lucas C, Leonard J, Finkele K (2010) Meteorological conditions and wildfire-related house loss in Australia. International Journal of Wildland Fire 19, 914-926.
| Crossref | Google Scholar |
Bureau of Meteorology (2011) Australian Weather Forecast Districts. Available at http://www.bom.gov.au/weather-services/maps/aus-weather-forecast-districts.shtml
Bureau of Meteorology (2015) Australian Digital Forecast Database (ADFD) User Guide. Available at http://www.bom.gov.au/catalogue/adfdUserGuide.pdf
Bureau of Meteorology (2023) Fire Weather Service Level Specification, Season 2022–2023, Severe Weather Environmental Prediction Services. Commonwealth of Australia. Available at http://www.bom.gov.au/weather-services/fire-weather-centre/fire-weather-services/documents/Fire_Weather_Service_Level_Specification.pdf
Burrows N, Gill M, Sharples J (2018) Development and validation of a model for predicting fire behaviour in spinifex grasslands of arid Australia. International Journal of Wildland Fire 27, 271-279.
| Crossref | Google Scholar |
Castellnou M, Guiomar N, Rego F, Fernandes P, Viegas DX (Ed.) (2018) Fire growth patterns in the 2017 mega fire episode of October 15, central Portugal. ‘Advances in Forest Fire Research 2018- Chapter 3 – Fire Management’ 447-453.
| Google Scholar |
Cheney NP (1968) Predicting fire behaviour with fire danger tables. Australian Forestry 32, 71-79.
| Crossref | Google Scholar |
Cheney NP, Gould JS, Catchpole WR (1998) Prediction of fire spread in grasslands. International Journal of Wildland Fire 8, 1-13.
| Crossref | Google Scholar |
Cheney NP, Gould JS, McCaw WL, Anderson WR (2012) Predicting fire behaviour in dry eucalypt forest in southern Australia. Forest Ecology and Management 280, 120-131.
| Crossref | Google Scholar |
Clarke H, Evans JP (2018) Exploring the future change space for fire weather in southeast Australia. Theoretical and Applied Climatology 136, 513-527.
| Crossref | Google Scholar |
Countryman CM (1966) Rating Fire Danger by the Multiple Basic Index System. Journal of Forestry 64, 531-536.
| Crossref | Google Scholar |
Cromer DAN (1946) Hygrothermographic fire danger rating and forecasting. Australian Forestry 10, 52-71.
| Crossref | Google Scholar |
Cruz MG, Alexander ME (2013) Uncertainty associated with model predictions of surface and crown fire rates of spread. Environmental Modelling & Software 47, 16-28.
| Crossref | Google Scholar |
Cruz MG, Alexander ME, Fernandes PM (2008) Development of a model system to predict wildfire behaviour in pine plantations. Australian Forestry 71, 113-121.
| Crossref | Google Scholar |
Cruz MG, Sullivan AL, Gould JS, Sims NC, Bannister AJ, Hollis JJ, Hurley RJ (2012) Anatomy of a catastrophic wildfire: the Black Saturday Kilmore East fire in Victoria, Australia. Forest Ecology and Management 284, 269-285.
| Crossref | Google Scholar |
Cruz MG, McCaw WL, Anderson WR, Gould JS (2013) Fire behaviour modelling in semi-arid mallee–heath shrublands of southern Australia. Environmental Modelling & Software 40, 21-34.
| Google Scholar |
Di Giuseppe F, Pappenberger F, Wetterhall F, Krzeminski B, Camia A, Libertá G, San Miguel J (2016) The potential predictability of fire danger provided by numerical weather prediction. Journal of Applied Meteorology and Climatology 55, 2469-2491.
| Crossref | Google Scholar |
Dimitrakopoulos AP, Bemmerzouk AM, Mitsopoulos ID (2011) Evaluation of the Canadian Fire Weather Index system in an eastern Mediterranean environment. Meteorological Applications 18, 83-93.
| Crossref | Google Scholar |
Dowdy AJ (2018) Climatological variability of fire weather in Australia. Journal of Applied Meteorology and Climatology 57, 221-234.
| Crossref | Google Scholar |
Dowdy AJ, Pepler A (2018) Pyroconvection risk in Australia: Climatological changes in atmospheric stability and surface fire weather conditions. Geophysical Research Letters 45, 2005-2013.
| Crossref | Google Scholar |
Fogarty LG, Pearce HG, Catchpole WR, Alexander ME (1998) ‘Adoption vs. adaption: Lessons from applying the Canadian Forest Fire Danger Rating System in New Zealand, Third International Conference on Forest Fire Research. In ‘14th Conference on Fire and Forest Meteorology. Vol. 1.’ Luso, Portugal, 16–20 November 1998. (Ed DX Viegas) pp. 631–645. (ADAI, University of Coimbra: Coimbra, Portugal)
Fujioka FM, Gill AM, Viegas DX, Wotton BM (2009) Fire Danger and Fire Behavior Modeling Systems in Australia, Europe, and North America. In ‘Chapter 21. Wildland Fires and Air Pollution. Developments in Environmental Science. Vol. 8’. (Eds A Bytnerowicz, MJ Arbaugh, AR Riebau, C Andersen) pp. 471–498. (Elsevier: Amsterdam, Netherlands)
Gisborne HT (1933) The wood cylinder method of measuring forest inflammability. Journal of Forestry 31, 683-689.
| Crossref | Google Scholar |
Gómez-González S, Ojeda F, Fernandes P (2018) Portugal and Chile: Longing for sustainable forestry while rising from the ashes. Environmental Science & Policy 81, 104-107.
| Crossref | Google Scholar |
Griffiths D (1999) Improved formula for the drought Factor in McArthur’s Forest Fire Danger Meter. Australian Forestry 62, 202-206.
| Crossref | Google Scholar |
Grootemaat S, Matthews S, Kenny BJ, Runcie J, Hollis JJ, Sauvage S, Fox-Hughes P, Holmes A (2024) Live trial performance of the Australian Fire Danger Rating System – Research Prototype. International Journal of Wildland Fire In press.
| Crossref | Google Scholar |
Hardy CC, Hardy CE (2007) Fire danger rating in the United States of America: an evolution since 1916. International Journal of Wildland Fire 16, 217-231.
| Crossref | Google Scholar |
Harris S, Anderson W, Kilinc M, Fogarty L (2012) The relationship between fire behaviour measures and community loss: an exploratory analysis for developing a bushfire severity scale. Natural Hazards 63, 391-415.
| Crossref | Google Scholar |
Hollis JJ, Matthews S, Anderson WR, Cruz MG, Fox-Hughes P, Grootemaat S, Kenny BJ, Sauvage S (2024) A framework for defining fire danger rating to support fire management operations in Australia. International Journal of Wildland Fire In press.
| Crossref | Google Scholar |
Hungerford RD, Harrington MG, Frandsen WH, Ryan KC, Niehoff GJ (1991) Influence of fire on factors that affect site productivity. In ‘Proceedings- of the symposium on Management and productivity of western-montane forest soils. General Technical Report INT-280’ Boise, ID, April 10-12, 1990. pp. 32–50 (US Department of Agriculture Forest Service Intermountain Research Station: Ogden, UT)
Kenny BJ, Matthews S, Sauvage S, Grootemaat S, Hollis JJ, Fox-Hughes P, Runcie J, Holmes A (2024) Australian Fire Danger Rating System: Implementing fire behaviour calculations to forecast fire danger. International Journal of Wildland Fire In press.
| Crossref | Google Scholar |
Kepert JD, Wain A, Tory KJ (2012) A comprehensive, nationally consistent climatology of fire weather parameters. In ‘Extended abstracts, Annual Conference of the Australasian Fire and Emergency Services Council and the Bushfire Cooperative Research Centre.Perth, Australia’ (Eds RP Thornton and LJ Wright) pp. 82–98. (Bushfire Co-operative Research Centre: East Melbourne, Australia)
Marsden-Smedley J, Catchpole WR (1995) Fire behaviour modelling in Tasmanian buttongrass moorlands. II. Fire behaviour. International Journal of Wildland Fire 5, 215-228.
| Crossref | Google Scholar |
Mills G, Salkin O, Fearon M, Harris S, Brown T, Reinbold H (2022) Meteorological drivers of the eastern Victorian Black Summer (2019–2020) fires. Journal of Southern Hemisphere Earth Systems Science 72, 139-163.
| Crossref | Google Scholar |
Ministerial Council for Police and Emergency Management (2018) ‘Communiqué. Ministerial Council for Police and Emergency Management 26 October 2018.’ (New Zealand). Available at https://www.homeaffairs.gov.au/how-to-engage-us-subsite/files/mcpem-communique-20181026.pdf
National Wildfire Coordinating Group (2019) Fire Behavior Field Reference Guide. No. PMS 437. Available at https://www.nwcg.gov/publications/pms437
Nauslar NJ, Abatzoglou JT, Marsh PT (2018) The 2017 North Bay and Southern California Fires: A Case Study. Fire 1, 18.
| Crossref | Google Scholar |
Noble IR, Gill AM, Bary GAV (1980) McArthur’s fire-danger meters expressed as equations. Australian Journal of Ecology 5, 201-203.
| Crossref | Google Scholar |
Nolan RH, Bowman DMJS, Clarke H, Haynes K, Ooi MKJ, Price OF, Williamson GJ, Whittaker J, Bedward M, Boer MM, Cavanagh VI, Collins L, Gibson RK, Griebel A, Jenkins ME, Keith DA, Mcilwee AP, Penman TD, Samson SA, Tozer MG, Bradstock RA (2021) What do the Australian Black Summer fires signify for the global fire crisis? Fire 4, 97.
| Crossref | Google Scholar |
Penman TD, Parkins KA, Mascaro S, Chong D, Bradstock RA (2015) ‘National Fire Danger Rating System probabilistic framework project. Final report year three. No. 2015.116.’ (Bushfire and Natural Hazards CRC: Melbourne, Australia) Available at https://www.bnhcrc.com.au/sites/default/files/managed/downloads/national_fdr_system_probabilistic_framework_year_3_report_final_for_publication.pdf
Pettinari M, Chuvieco E (2017) Fire behavior simulation from global fuel and climatic information. Forests 8, 179.
| Crossref | Google Scholar |
San-Miguel-Ayanz J, Carlson JD, Alexander M, Tolhurst KG, Morgan G, Sneeuwjagt RJ, Dudley M (2003) Current methods to assess fire danger potential. In ‘Wildland fire danger estimation and mapping: The role of remote sensing data. Vol. 4’. Series in remote sensing. (Ed. E Chuvieco) pp. 21–61. (World Scientific Publishing: Singapore)
Sauvage S, Fox-Hughes P, Matthews S, Kenny B, Hollis J, Grootemaat S, Runcie J, Holmes A, Harris R, Love P, Williamson G (2024) Australian Fire Danger Rating System Research Prototype: A climatology. International Journal of Wildland Fire In press.
| Crossref | Google Scholar |
Sharples JJ, McRae RHD, Weber RO, Gill AM (2009) A simple index for assessing fire danger rating. Environmental Modelling & Software 24, 764-774.
| Crossref | Google Scholar |
Sirakoff C (1985) A correction to the equations describing the McArthur forest fire danger meter. Australian Journal of Ecology 10, 481.
| Crossref | Google Scholar |
Stocks BJ, LynhamLawson TJ, Lawson BD, Alexander ME, Wagner CEV, McAlpine RS, Dubé DE (1989) The Canadian Forest Fire Danger Rating System: an overview. Forestry Chronicle 65, 450-457.
| Crossref | Google Scholar |
Taylor SW, Alexander ME (2006) Science, technology, and human factors in fire danger rating: the Canadian experience. International Journal of Wildland Fire 15, 121-135.
| Crossref | Google Scholar |
Viegas DX, Bovio G, Ferreira A, Nosenzo A, Sol B (1999) Comparative study of various methods of fire danger evaluation in southern Europe. International Journal of Wildland Fire 9, 235-246.
| Crossref | Google Scholar |
Wallace WR (1936) Forest fire weather research in Western Australia. Australian Forestry 1, 17-24.
| Crossref | Google Scholar |
Xanthopoulos G, Athanasiou M (2019) Attica Region, Greece July 2018: A tale of two fires and a seaside tragedy. Wildfire 28/2, 18-21.
| Google Scholar |
Zacharakis I, Tsihrintzis VA (2023) Integrated wildfire danger models and factors: a review. Science of the Total Environment 899, 165704.
| Crossref | Google Scholar | PubMed |