Life-history characteristics of the eastern shovelnose ray, Aptychotrema rostrata (Shaw, 1794), from southern Queensland, Australia
Matthew J. Campbell
A Queensland Department of Agriculture and Fisheries, Agri-Science Queensland, Ecosciences Precinct, GPO Box 267, Brisbane, Qld 4001, Australia.
B Centre for Sustainable Tropical Fisheries and Aquaculture and College of Science and Engineering, James Cook University, 1 James Cook Drive, Townsville, Qld 4811, Australia.
C Corresponding author. Email: matthew.campbell@daf.qld.gov.au
Marine and Freshwater Research 72(9) 1280-1289 https://doi.org/10.1071/MF20347
Submitted: 4 December 2020 Accepted: 26 March 2021 Published: 7 May 2021
Journal Compilation © CSIRO 2021 Open Access CC BY ©The State of Queensland (through the Department of Agriculture and Fisheries Queensland) [2021] and The Authors 2021
Abstract
The eastern shovelnose ray (Aptychotrema rostrata) is a medium-sized coastal batoid endemic to the eastern coast of Australia. It is the most common elasmobranch incidentally caught in the Queensland east coast otter trawl fishery, Australia’s largest penaeid-trawl fishery. Despite this, age and growth studies on this species are lacking. The present study estimated the growth parameters and age-at-maturity for A. rostrata on the basis of sampling conducted in southern Queensland, Australia. This study showed that A. rostrata exhibits slow growth and late maturity, which are common life-history strategies among elasmobranchs. Length-at-age data were analysed within a Bayesian framework and the von Bertalanffy growth function (VBGF) best described these data. The growth parameters were estimated as L0 = 193 mm TL, k = 0.08 year–1 and L∞ = 924 mm TL. Age-at-maturity was found to be 13.3 years and 10.0 years for females and males respectively. The under-sampling of larger, older individuals was overcome by using informative priors, reducing bias in the growth and maturity estimates. As such, the results can be used to derive estimates of natural mortality for this species.
Keywords: life history, growth, age-at-maturity, elasmobranch, Aptychotrema rostrata.
Introduction
The Queensland east coast otter trawl fishery (QECOTF) is the largest penaeid-trawl fishery in Australia. This fishery targets shrimps (Penaeidae: Melicertus spp., Penaeus spp., Metapenaeus spp.), sea scallops (Pectinidae: Ylistrum balloti), bugs (Scyllaridae: Thenus spp. and Ibacus spp.) and squid (Teuthoidea) with demersal otter trawl gear. In 2019, logbook data indicated that 299 vessels fished 35 950 days and landed ~5986 t of product for sale at both domestic and international markets. Further, two vessels target stout whiting (Sillago robusta), using Danish seine and fish trawl gear, in southern Queensland and are subject to an annual total allowable catch (TAC) of ~1100 t.
It has been estimated that 55% of the global catch from penaeid trawls is discarded (Gilman et al. 2020). The discard rate from the QECOTF is higher at 70%, resulting in >25 000 t being discarded annually (Wang et al. 2020), representing 28.5% of Australia’s total annual discards (Kennelly 2020). Consequently, quantifying and mitigating discards have been the subjects of significant research efforts in Queensland since the mid-1990s (e.g. Robins-Troeger 1994; Robins and McGilvray 1999). Hundreds of species comprise the discarded potion of the QECTOF catch (Courtney et al. 2006; Courtney et al. 2008), some of which are of conservation concern, such as sea turtles (McGilvray et al. 1999).
Elasmobranchs (i.e. sharks and rays) are one component of penaeid-trawl discards that have received increasing attention in the past two decades (Dulvy et al. 2017). Elasmobranch life-history strategies, including late maturity, few offspring, long life spans and slow growth (Dulvy et al. 2008), make this group vulnerable to over-exploitation (Stevens et al. 2000). Twenty-five per cent of elasmobranchs have an elevated risk of extinction as a result of capture in fisheries (Dulvy et al. 2017; Simpfendorfer and Dulvy 2017) and the species of Rhinopristiformes (wedgefishes and guitarfishes) are of a particular concern (Kyne et al. 2020). The introduction of turtle excluder devices (TEDs) has gone some way to reduce this risk in penaeid-trawl fisheries, particularly for larger species: however, TEDs remain ineffective for smaller elasmobranchs (Campbell et al. 2020).
The TEDs used in the QECOTF have no effect on the catch rate of the eastern shovelnose ray (Trygonorrhinidae: Aptychotrema rostrata, Shaw 1794; Courtney et al. 2008). This is the most common elasmobranch in the discarded portion of the penaeid-trawl (Kyne et al. 2002) and S. robusta (Rowsell and Davies 2012) catches in southern Queensland (>22°S). Aptychotrema rostrata is endemic to the eastern coast of Australia between Halifax Bay in northern Queensland (18°30′S) and Merimbula in southern New South Wales (36°53′S). The species rarely exceeds 1 m total length (TL), generally in depths of <100 m (Last and Stevens 2009), and feeds on crustaceans, teleost fish and squid (Kyne and Bennett 2002a). In southern Queensland, parturition occurs in November and December after a gestation period of 3–5 months, with litter sizes of 4–18 pups (Last et al. 2016).
In Queensland, the incidental capture of A. rostrata in the QECOTF is the main source of fishing mortality, although post-release survival is high (Campbell et al. 2018). In 2010, the two vessels targeting S. robusta caught 3075 A. rostrata individuals, of which ~22% were released alive (Rowsell and Davies 2012). Recreational anglers land A. rostrata (Kyne and Stevens 2015); however, the catch is negligible in Queensland (J. Webley, Fisheries Queensland, pers. comm.).
Despite its frequent occurrence in trawl catches, age and growth studies on A. rostrata are lacking. Diet (Kyne and Bennett 2002a), dentition (Gutteridge and Bennett 2014), sensory characteristics (Hart et al. 2004; Wueringer et al. 2009) and post-trawl survival (Campbell et al. 2018) have been the subject of recent research. Although reproductive strategies were described in two studies (Kyne and Bennett 2002b; Kyne et al. 2016), no previous study has quantified growth and age-at-maturity.
The lack of these data and the absence of information regarding the number of A. rostrata individuals caught annually are the main impediments for the assessment of population status. Currently, the IUCN Red List of Threatened Species categorises A. rostrata as ‘Least Concern’ (Kyne and Stevens 2015). In Australia, all fisheries are subject to environmental assessment, whereby jurisdictions are required to demonstrate that the impacts on individual species, both target and non-target, are sustainable in the long-term. Failure to do so can result in the revocation of export privileges, prohibiting access to lucrative international markets.
Previous qualitative ecological risk assessments (ERAs) have indicated that trawling in Queensland (Pears et al. 2012; Jacobsen et al. 2018) and New South Wales (Astles et al. 2009) poses a high ecological risk to A. rostrata in the respective jurisdictions. These ERAs rely on qualitative assessments of a species’ exposure and resilience to trawling, rather than empirical data, to assess risk. Generally, qualitative ERAs overestimate the ecological risk posed by fishing than the quantitative ERAs when compared with results from formal stock assessments (Zhou et al. 2016). As such, life-history data are fundamental to assessing stock status and form the basis of quantitative ERAs, an improved method for assessing data-poor species such as A. rostrata. The aim of the present study, therefore, was to estimate the growth parameters and age-at-maturity of A. rostrata.
Materials and methods
Specimens of A. rostrata were primarily obtained from the operator of a Danish seine vessel, the FV San Antone II, targeting S. robusta in southern Queensland on an ad hoc basis in the period between April 2016 and November 2017. The San Antone II is a 17 m steel twin-hulled vessel powered by two 148 kW diesel engines. The Danish seine gear consisted of two 2500 m sweeps separated by a single net with a headline length of 34.75 m, with a mesh size of 85 mm in the wings and 55 mm in the codend. Samples were collected in southern Queensland waters between Sandy Cape (24°42.043′S, 153°16.027′E) and Coolangatta (28°09.844′S, 153°32.942′E) in depths between 35 and 50 m. During commercial operations, A. rostrata individuals were removed from the catch and stored whole in the vessel’s freezer for processing in the laboratory.
Sample collection on the San Antone II was supplemented by specimens obtained during the post-trawl survival (PTS) experiments conducted by Campbell et al. (2018).
Laboratory processing
All A. rostrata individuals were thawed, sexed, weighed (±0.01 g) and measured (total length, TL, ±0.1 cm). In accordance with Pierce and Bennett (2009), a segment of four or five vertebrae, located at the posterior of the abdominal cavity, was excised. Each segment was cleaned following Goldman et al. (2004) and air dried. The neural and haemal arches were removed, along with any remnant connective tissue. After drying, each segment was embedded in polyester resin and sectioned with a Buehler IsoMet Low Speed cutting saw (www.buehler.com/isoMet-low-speed-cutter.php), at a width of ~200 µm, and mounted on a microscope slide. The vertebral sections were examined with a Leica M60 stereo microscope (www.leica-microsystems.com/products/stereo-microscopes-macroscopes/p/leica-m80/) under reflected light on a matt black background, and photographed with a Leica IC90 E digital camera (www.leica-microsystems.com/products/microscope-cameras/p/leica-ic90-e/).
The maturity of each individual was assessed according to Kyne et al. (2016). Maturity in males depended on the calcification of the claspers, categorised as immature (possessing short, flexible, uncalcified claspers) or mature (rigid, calcified and elongated claspers). A mature female A. rostrata possessed one or more of the following: developed ovaries with yellow vitellogenic follicles of ≥5 mm diameter, fully developed oviducal glands and uteri, uterine eggs, and embryos in situ. Immature females were categorised by undifferentiated ovaries, undeveloped oviducal glands and thin uteri.
Ageing
Nominal age was estimated by two readers on the basis of the number of band pairs. A band pair was defined following Fig. 1c from Rolim et al. (2020) as one (narrow) translucent band and one wide (opaque) band, combined. Initially, the birth mark was defined as an angle change along the corpus calcareum (White et al. 2014), associated with the first distinct opaque band after the focus (called the ‘birth mark’, Campana 2014). However, preliminary investigation showed that the birth mark and the change of angle was absent or difficult to identify in a high proportion of individuals. As such, the first growth band (i.e. 1 year of age) was identified using a method described by Campana (2014). The mean distance between the waist and distal edge of the first growth band was calculated by measuring this distance for those 1-year-old animals (<~25 cm TL) where the birthmark was visible. This distance was measured with the Leica Application Suite software associated with the camera used to view centrum images. A line of this length was superimposed on the image of each sectioned centrum to determine the expected location of the first complete opaque band after the birth mark.
Counts were made without knowledge of the size or sex of the animal and the readability of each section was qualitatively assessed in accord with Officer et al. (1996). Where counts differed between readers, the count by the experienced reader was accepted. The following three measures of precision were calculated to assess consistency between readers: (1) percentage agreement (PA); (2) average percentage error (APE, Beamish and Fournier 1981); and (3) average coefficient of variation (ACV, Chang 1982). Further, Bowker’s test of symmetry was used to assess bias among readers.
Marginal increment ratio (MIR)
To determine the periodicity of band formation, monthly MIR was calculated following Natanson et al. (1995), who defined MIR as MIR = (CR – CRn) / (CRn – CRn–1), where CR is the centrum radius, CRn is the radius of the final complete band pair and CRn–1 is the radius of the next to last complete band pair. Given this method, MIR was calculated only for animals aged ≥2 years. Following (Simpfendorfer et al. 2000), MIR was compared among months using the Kruskal–Wallis one-way analysis of variance on ranks.
Edge type was qualitatively assessed to provide further evidence of band formation periodicity (Cailliet et al. 2006) and was classified into three levels, namely, ‘new’, ‘intermediate’ and ‘wide’. A ‘new’ edge was one where an opaque zone occurred at the distal edge of the centrum irrespective of the width of the opaque band. An edge of a centrum with any translucence visible beyond the last complete band pair was categorised as ‘intermediate’ and an edge was classified as ‘wide’ if the width of the translucent band beyond the last complete band pair was ≥2/3 the width of the previous translucent band. A chi-square test was used to compare the observed frequency of each edge type, as a function of month, with the expected frequencies. In this case, the null hypothesis of the test was that the frequency of edge type was not dependent on month of capture.
Growth
Band pair counts (i.e. nominal age) were adjusted for growth beyond the last complete band pair on the basis of edge type (Pierce and Bennett 2009). Nominal age was increased by 0.33 year for intermediate edges and by 0.66 year for wide edges.
Initial analysis indicated that younger individuals were under-sampled. As such, back-calculation techniques were used to increase the sample size of smaller size classes. The linear-modified Dahl–Lea method (Francis 1990) was used to estimate the total length (La) of each individual at age a, as follows:
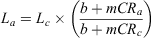
where Lc is the length at capture; CRa is the centrum radius at age a; CRc is the centrum radius at capture; and b and m are the coefficients of the linear regression between CRc and Lc. This method was preferred to the Dahl–Lea direct proportions method because the CRc – Lc relationship did not pass through the origin (Goldman 2005). Following Goldman (2005), the quadratic-modified Dahl–Lea method (Francis 1990) was used for comparison with the linear-modified Dahl–Lea method to determine the most appropriate approach for estimating La as a function of CRa. Francis (1990) defined the quadratic-modified Dahl–Lea equation as

where d, e and f are the quadratic regression estimates. The mean observed lengths and the mean back-calculated lengths, as a function of age, were compared using two-sample Student’s t-tests where sample size permitted. In this case, the observed lengths were restricted to those animals where new or intermediate edges occurred at the distal edge of the centrum.
In accord with Smart et al. (2016), the following three growth functions were used to estimate mean length-at-age: von Bertalanffy growth function (VBGF), logistic function and Gompertz function (Table 1). In all instances, the biologically relevant length-at-birth (L0) was estimated, rather than the age when length is zero (i.e. t0), as recommended for elasmobranchs by Cailliet et al. (2006). Relevant parameters were estimated via non-linear least-squares regression: however, the under-sampling of larger individuals resulted in an under-estimate of L∞. As such, a Bayesian approach using Markov-chain Monte Carlo (MCMC) was used to estimate biologically appropriate growth parameters (Emmons et al. 2021).
![]() |
Bayesian models were fit using the ‘BayesGrowth’ package (Smart 2020; accessed 18 February 2021), by using R statistical software (ver. 3.6.1, R Foundation for Statistical Computing, Vienna, Austria, see https://www.R-project.org/, accessed 18 February 2021), in accord with methods described by Smart and Grammer (2021) and Emmons et al. (2021). The ‘BayesGrowth’ package uses the ‘Stan’ computer program (Carpenter et al. 2017), via the ‘Rstan’ package (Stan Development Team 2020) to perform MCMC using no U-turn sampling (NUTS). Four MCMC chains with 10 000 simulations, with a burn-in period of 5000 simulations, were used to determine parameter posterior distributions. Model convergence was assessed using the Gelman–Rubin test and diagnostic plots generated using the ‘Bayesplot’ package (Gabry 2020; accessed 18 February 2021) in R.
The models were fit with a normal residual error structure (σ). Prior distributions for the L0 and L∞ estimates were informed by data published by Last et al. (2016). These authors reported the maximum size of A. rostrata as 1200 mm TL and with a length-at-birth (L0) of 130–150 mm TL. Given this information, priors were set at L∞ ~N(1200, 50) and L0 ~N(140, 10). A non-informative prior was used for σ and a common non-informative prior was used for the growth coefficients of candidate models (k, g1 and g2, Table 1). An upper bound was nominated for the uniform distributions of σ and k of 100 and 0.3 year–1 respectively. The common non-informative prior for the growth coefficients allowed for comparison of the three candidate growth functions, each with identical priors. Leave-one-out-information-criterion weights (LOOICw), calculated within the ‘BayesGrowth’ package using the ‘loo’ R package (Vehtari et al. 2020), were used to determine the most appropriate candidate model. As with the Akaike weights in the frequentist approach, the candidate model with the highest LOOICw was considered the most appropriate.
Maturity
To overcome the under-sampling of larger, older animals, Beverton–Holt life-history invariants (BH–LHI) were used to estimate of age-at-maturity (t50) and length-at-maturity (L50). Life-history ratios described by Jensen (1996) and Frisk et al. (2001) were used to estimate t50 and L50 by using natural mortality (M) and the previously defined k and L∞ (L50/L∞ = 0.66, ln(M) = 0.42 × ln(k) – 0.83 and M × t50 = 1.65).
Results
Overall, 214 A. rostrata individuals were collected to assess growth; 142 were collected by the crew of the San Antone II and 72 were collected as part of the PTS experiments conducted by Campbell et al. (2018). The animals caught during the PTS experiments had significantly smaller TL than those caught on the San Antone II (t = –4.180, d.f. = 166.7, P < 0.001). Two animals were excluded from the analysis, because age could not be determined from the respective vertebral centra. Of the 212 animals assessed for growth, 102 were female with a mean TL of 403 mm (s.e. = 11.00, range = 192–753) and 110 were male with a mean TL of 413 mm (s.e. = 11.16, range = 193–671). No significant difference in size was detected between sexes (t = –0.670, d.f. = 209.9, P = 0.504; Fig. 1).
![]() |
Ageing
Generally, ageing between readers was consistent (PA = 82.67%, ACV = 4.21, APE = 2.97), with the age bias plot showing little variation from the 1:1 line of equivalence (Supplementary material Fig. S1, available at the journal’s website). Further, Bowker’s test of symmetry showed no between-reader bias (χ2 = 13.93, d.f. = 12, P = 0.305). The nominal age (i.e. the number of complete band pairs) range of males and females was 0–15 years and 0–17 years respectively. The oldest female was 750 mm TL and the two males assigned the nominal age of 15 years were 624 and 648 mm TL.
Marginal increment ratio
Marginal increment ratio was lowest during August and September (Fig. S2). New edges were also most likely to occur during these months. The Kruskal–Wallis test on ranks indicated that MIR varied significantly among months (χ2 = 23.927, d.f. = 4, P < 0.001). Mean MIR decreased from March through to August, before increasing in September. The highest mean MIR occurred in November, at the end of the austral spring.
Wide edges were also most likely to occur in November; however, the frequency of each edge type was not dependent on the month (χ2 = 12.67, d.f. = 8, P = 0.124).
Growth
The relationship between TL and CR was best described by the quadratic-modified Dahl–Lea method (TL = –0.038CR2 + 12.312CR – 17.01, R2 = 0.964). Back-calculated and observed lengths-at-age were not significantly different (Supplementary material Table S1). As such, the observed and back-calculated data were combined, resulting in a dataset containing 1112 measures of length-at-age.
The VBGF was found to best fit the length-at-age data (Table 2, LOOICw = 1). There was no support for either the Gompertz (LOOICw = 0) or the Logistic (LOOICw = 0) growth functions. With the sexes combined, the estimated VBGF parameters were L∞ = 923 mm TL, L0 = 193 mm TL and k = 0.08 year–1 (Table 2, Fig. 2, Fig. S3). Estimates of L∞ and L0 were higher for females (1141 and 193 mm respectively) than males (813 and 187 mm respectively; Fig. 3). The growth coefficient for females (k = 0.05 year–1) was half of that for males (k = 0.10 year–1).
![]() |
![]() |
Maturity
Of the 212 A. rostrata individuals used to assess growth, only nine females and nine males were sexually mature. The oldest immature animals (female and male) were >10 years of age, whereas the youngest mature animals were 6 years. Using the BH–LHI described by Jensen (1996) and Frisk et al. (2001), age-at-maturity for both sexes combined was 10.9 years, and 13.3 and 10.0 years for females and males respectively. Further, length-at-maturity was 609 mm TL for both sexes combined, and 753 and 555 mm TL for females and males respectively.
Discussion
The results from the current study represent the first estimates of growth and age-at-maturity published in the primary literature for A. rostrata. Slow growth and late maturity are common among elasmobranchs (Dulvy et al. 2008), making this group vulnerable to over-exploitation (Stevens et al. 2000). These characteristics, combined with intense fishing pressure, have resulted in an increasing concern for Rhinopristiformes, many of which are at an extremely high risk of extinction (Kyne et al. 2020). The landings and catch rates of Rhinopristiformes species have declined by up to 80% throughout most of their ranges (D’Alberto et al. 2019); however, a combination of reduced fishing pressure, prohibiting the retention of shark products and networks of marine protected areas have been shown to mitigate risk for this group (Kyne et al. 2020). This is especially the case for A. rostrata, which is considered abundant because of its diverse habitat use and the extent of refuges across its range (Kyne and Stevens 2015). Significant reduction in shrimp trawl effort since 2000 (Wang et al. 2020) is also likely to have had a positive effect on the species’ abundance in Queensland.
These factors ensure the continued high levels of abundance in Queensland despite this species’ low productivity. Delayed maturity and small maximum size imply a low maximum intrinsic population growth rate (rmax) in Rhinopristiformes (D’Alberto et al. 2019). These authors evaluated population productivity in nine Rhinopristiformes and concluded that the trygonorrhinids exhibit low rmax values compared with larger species such as the giant shovelnose ray (Glaucostegus typus) and bottlenose wedgefish (Rhynchobatus australiae), both of which co-occur with A. rostrata. This is due to the ability of these larger species to produce numerous and large offspring. Age-at-maturity was also found to be negatively correlated with productivity and the t50 derived for A. rostrata using the BH–LHI is higher than the estimates for all nine species assessed by D’Alberto et al. (2019).
Maximum intrinsic population growth rate was calculated for only nine species of Rhinopristiformes because of the lack of reliable life-history information. Only three of the eight species that comprise Trygonorrhinidae have published growth information, namely, southern fiddler ray (Trygonorrhina dumerilii), shortnose guitarfish (Zapteryx brevirostris) and banded guitarfish (Zapteryx exasperata). The VBGF growth coefficient derived in the current study, k = 0.08 year–1, is lower than any of those published for Trygonorrhinidae. Values of k have been published for T. dumerilii and Z. brevirostris at 0.13 year–1 (Izzo and Gillanders 2008) and 0.12 year–1 (Carmo et al. 2018) respectively. Cervantes-Gutiérrez et al. (2018) reported a higher growth coefficient for male and female Z. exasperata of k = 0.174 year–1 and k = 0.144 year–1 respectively. Additionally, Caltabellotta et al. (2019) reported faster growth in the smaller Z. brevirostris of k = 0.24 year–1.
Only T. dumerilii has a higher published estimate of L∞ than that presented here. Izzo and Gillanders (2008) reported an L∞ for T. dumerilii of 1129 mm TL for females and males combined. The L∞ (1157 mm TL) for female T. dumerilii is similar to that derived in the current study for female A. rostrata (1141 mm TL), despite T. dumerilii reaching a higher maximum size (1460 mm TL, Last et al. 2016). Cervantes-Gutiérrez et al. (2018) reported estimates of L∞ for female and male Z. exasperata of 1007 mm and 898 mm respectively. In contrast, Caltabellotta et al. (2019) reported an L∞ of 624 mm and 602 mm for female and male Z. brevirostris, whereas Carmo et al. (2018) derived smaller values of 56.0 cm and 50.4 cm respectively. In accord with other elasmobranchs, the published estimates of L∞ for the trygonorrhinids were higher for females; Cortés (2000) found that the maximum size of males was, on average, ~10% smaller than that of females in 164 shark species.
Kyne et al. (2016) reported an L50 for male A. rostrata of 597.3 mm. This is comparable to the present study: however, their L50 for females (639.5 mm) is lower than the L50 reported here. This difference in L50 may be a result of using the BH–LHIs calculated using the estimates of k and L∞ derived here. The under-sampling of large, mature females resulted in biased estimates of female L50, necessitating the use of the BH–LHI. This under-sampling may have been due to the selectivity of the sampling gears used in the respective studies. Kyne et al. (2016) conducted sampling using shrimp (Melicertus plebejus) trawls in water depths to 100 m. In contrast, samples in the present study were predominantly (67%) collected on the San Antone II, which deploys Danish seine gear to target S. robusta in water of <50 m. The Danish seine used in this fishery is characterised by slow haul speeds and short haul times (Rowsell and Davies 2012), which may allow larger A. rostrata to escape capture.
The difference in water depth is unlikely to be the cause of the under-sampling of large animals. Kyne and Bennett (2002b) collected A. rostrata individuals from Moreton Bay, adjacent to the grounds in the current study, and reported that 41 of 48 (~85%) females sampled were mature. These authors used rod-and-reel in water depths of 3–10 m and reported a female L50 similar to that in Kyne et al. (2016). The number of mature females was higher than from the current study; only 9 of the 102 females caught aboard the San Antone II were mature and no mature animals were collected during the PTS experiments conducted by Campbell et al. (2018).
Sexual bimaturism is a common life-history strategy among viviparous elasmobranchs (Colonello et al. 2020) because of less investment by females in growth to compensate for attaining a larger size to support pups (Cortés 2000). The higher L50 and t50 derived here for female A. rostrata are consistent with Kyne et al. (2016), who estimated a higher L50 for female A. rostrata. Similarly, Jones et al. (2010) reported a higher L50 for females for the congeneric A. vincentiana caught in southern Western Australia. Delayed female maturity has been reported for the confamilial banded guitarfish (Zapteryx exasperate) caught in Mexico (Cervantes-Gutiérrez et al. 2018).
The MIR analysis suggested that band pair formation occurs annually. Ideally, sampling should have occurred throughout the year to ensure a complete analysis of the periodicity of band pair formation; however, the seasonal nature of fisheries that catch A. rostrata as by-catch resulted in irregular access to samples during the study period. Similarly, Cervantes-Gutiérrez et al. (2018) reported that fishery closures hampered year-round sampling of Z. exasperata in Mexico and published incomplete measures of marginal increments; however, these authors assumed annual band pair formation when quantifying growth. Caltabellotta et al. (2019) suggested annual band formation for Z. brevirostris by using MIR and, like in the previous studies on the growth of trygonorrhinids (Izzo and Gillanders 2008; Carmo et al. 2018), this assumption is also reasonable for A. rostrata. The minimum MIR occurred in August, indicating a period of slow somatic growth coinciding with minimum monthly mean sea-surface temperature (Meynecke and Lee 2011) and high reproductive activity (Kyne et al. 2016). The chi-square test conducted on the edge frequency data somewhat contradicted the results from the MIR analysis and, as such, further sampling should be undertaken throughout the year to confirm that band pair formation occurs annually.
The back-calculated lengths-at-age were not significantly different from the observed values. However, the mean back-calculated lengths were higher than the observed mean lengths for ages 0–6 years because of the inclusion of those vertebral centra where intermediate edges were observed. The low number of vertebral centra with new edges at each age necessitated the inclusion of the centra with intermediate edges for robust comparison between back-calculated and observed lengths-at-age.
The under-sampling of older animals resulted in biased growth parameter estimates. However, estimating the VBGF parameters in a Bayesian framework allowed for the use of informed priors to estimate L∞, overcoming the lack of larger animals sampled. Similarly, back-calculation increases the number of measures of length-at-age for smaller size classes, resulting in improved growth parameter estimates for elasmobranchs where age data are sparse for smaller individuals (e.g. Smart et al. 2013; D’Alberto et al. 2017; Carmo et al. 2018). These two techniques allowed for the estimation of reasonable growth parameters for use in assessing the population status of A. rostrata.
Various methods correlate k and L∞ to natural mortality (M, e.g. Pauly 1980; Frisk et al. 2001; Then et al. 2015). As such, biased estimates of growth result in biased estimates of M (D’Alberto et al. 2019), leading to inaccurate assessments of stock status (Pardo et al. 2013). Estimating growth parameters in a Bayesian framework overcomes this bias (Smart and Grammer 2021). Campbell et al. (2017) quantified the ecological risk posed to A. rostrata by the ECOTF, by using the sustainability assessment for fishing effects (SAFE) quantitative ERA developed by (Zhou et al. 2009). In this instance, risk was quantified via comparison between the level of fishing mortality (F) and the maximum sustainable fishing mortality (Fmsm), where Fmsm = 0.41M (Zhou et al. 2012). Hence, the growth parameter estimates derived here allow for the calculation of unbiased estimates M and Fmsm, which enable the accurate assessment of the population status.
Campbell et al. (2017) assessed the risk posed to 47 elasmobranchs, only 18 of which had published growth estimates. Of these 18, growth was quantified for seven species on the basis of samples collected within the study area. This reinforces the need for basic life-history data to inform fishery impacts in batoids (Kyne 2016) and elasmobranchs in general. In the absence of life-history information, previous studies (Zhou et al. 2013; Zhou et al. 2015) have used the ‘Life History Tool’ on the Fishbase website (www.fishbase.se) to determine values for M. There is a need, therefore, to increase knowledge of life-history information to ensure the accurate assessment of fishery impacts on elasmobranchs with sparse catch data.
In conclusion, the current study has contributed to the scientific knowledge of A. rostrata, and Rhinopristiformes more broadly. Consistent with other elasmobranchs, A. rostrata exhibits slow growth, late maturity and a long lifespan. Despite this, the species is abundant in Queensland owing to its diverse habitat use and the extent of refuges throughout its range. This result contrasts with other Rhinopristiformes, many of which are at a high risk of extinction. The life-history characteristics derived from this research can be used in future studies to determine population status and inform management decisions.
Conflicts of interest
Colin Simpfendorfer is an Associate Editor for Marine and Freshwater Research. Despite this relationship, he did not at any stage have Associate Editor-level access to this manuscript while in peer review, as is the standard practice when handling manuscripts submitted by an editor to this journal. Marine and Freshwater Research encourages its editors to publish in the journal and they are kept totally separate from the decision-making process for their manuscripts. The authors declare that they have no further conflicts of interest.
Declaration of funding
The Fisheries Research and Development Corporation (FRDC Project Number 2015/014) and the Queensland Department of Agriculture and Fisheries provided the funding for this work. Our thanks go to these institutions for their continued support for fisheries research.
Acknowledgements
The authors extend sincerest thanks to Matt Wills, Master of the C-Rainger, for his patience and enthusiasm for this research. We are grateful to Michael Pinzone, Master of the San Antone II, and his crew for collecting samples. The authors thank Sean Maberly, Master of the FRV Tom Marshall, for his contribution during the PTS experiments. Jonathan Smart provided advice on the analysis of the length-at-age data in a Bayesian framework and his assistance is gratefully acknowledged. Sophie Eammons provided an earlier version of her tiger shark growth study, which helped in the analysis of the length-at age data here. We sincerely thank Zalee Bates and Pat Abbott for their continued diligence in sourcing and supplying relevant literature. We thank two reviewers whose comments and suggestions improved this paper. This work was authorised under the Queensland Department of Agriculture and Fisheries General Fisheries Permit number 186281, The Queensland National Parks and Wildlife Marine Parks permit QS2015/MAN322, and the Queensland Animal Ethics Approval Number CA2015/06/867.
References
Astles, K. L., Gibbs, P. J., Steffe, A. S., and Green, M. (2009). A qualitative risk-based assessment of impacts on marine habitats and harvested species for a data deficient wild capture fishery. Biological Conservation 142, 2759–2773.| A qualitative risk-based assessment of impacts on marine habitats and harvested species for a data deficient wild capture fishery.Crossref | GoogleScholarGoogle Scholar |
Beamish, R., and Fournier, D. (1981). A method for comparing the precision of a set of age determinations. Canadian Journal of Fisheries and Aquatic Sciences 38, 982–983.
| A method for comparing the precision of a set of age determinations.Crossref | GoogleScholarGoogle Scholar |
Cailliet, G. M., Smith, W. D., Mollet, H. F., and Goldman, K. J. (2006). Age and growth studies of chondrichthyan fishes: the need for consistency in terminology, verification, validation, and growth function fitting. Environmental Biology of Fishes 77, 211–228.
| Age and growth studies of chondrichthyan fishes: the need for consistency in terminology, verification, validation, and growth function fitting.Crossref | GoogleScholarGoogle Scholar |
Caltabellotta, F. P., Siders, Z. A., Murie, D. J., Motta, F. S., Cailliet, G. M., and Gadig, O. B. F. (2019). Age and growth of three endemic threatened guitarfishes Pseudobatos horkelii, P. percellens and Zapteryx brevirostris in the western South Atlantic Ocean. Journal of Fish Biology 95, 1236–1248.
| Age and growth of three endemic threatened guitarfishes Pseudobatos horkelii, P. percellens and Zapteryx brevirostris in the western South Atlantic Ocean.Crossref | GoogleScholarGoogle Scholar | 31429078PubMed |
Campana, S. E. (2014). ‘Age determination of elasmobranchs, with special reference to Mediterranean species: a technical manual. Studies and Reviews.’ (General Fisheries Commission for the Mediterranean: Rome, Italy.)
Campbell, M., Courtney, T., Wang, N., McLennan, M., and Zhou, S. (2017). Estimating the impacts of management changes on bycatch reduction and sustainability of high-risk bycatch species in the Queensland East Coast Otter Trawl Fishery: final report for FRDC Project no. 2015/014. Queensland Department of Agriculture and Fisheries, Brisbane, Qld, Australia. Available at http://frdc.com.au/Archived-Reports/FRDC%20Projects/2015-014-DLD.pdf
Campbell, M. J., McLennan, M. F., Courtney, A. J., and Simpfendorfer, C. A. (2018). Post-release survival of two elasmobranchs, the eastern shovelnose ray (Aptychotrema rostrata) and the common stingaree (Trygonoptera testacea), discarded from a prawn trawl fishery in southern Queensland, Australia. Marine and Freshwater Research 69, 551–561.
| Post-release survival of two elasmobranchs, the eastern shovelnose ray (Aptychotrema rostrata) and the common stingaree (Trygonoptera testacea), discarded from a prawn trawl fishery in southern Queensland, Australia.Crossref | GoogleScholarGoogle Scholar |
Campbell, M. J., Tonks, M. L., Miller, M., Brewer, D. T., Courtney, A. J., and Simpfendorfer, C. A. (2020). Factors affecting elasmobranch escape from turtle excluder devices (TEDs) in a tropical penaeid-trawl fishery. Fisheries Research 224, 105456.
| Factors affecting elasmobranch escape from turtle excluder devices (TEDs) in a tropical penaeid-trawl fishery.Crossref | GoogleScholarGoogle Scholar |
Carmo, W. P., Fávaro, L. F., and Coelho, R. (2018). Age and growth of Zapteryx brevirostris (Elasmobranchii: Rhinobatidae) in southern Brazil. Neotropical Ichthyology 16, e170005.
| Age and growth of Zapteryx brevirostris (Elasmobranchii: Rhinobatidae) in southern Brazil.Crossref | GoogleScholarGoogle Scholar |
Carpenter, B., Gelman, A., Hoffman, M. D., Lee, D., Goodrich, B., Betancourt, M., Brubaker, M., Guo, J., Li, P., and Riddell, A. (2017). Stan: A Probabilistic Programming Language. 2017-01-11. Available at https://www.jstatsoft.org/v076/i01
Cervantes-Gutiérrez, F., Tovar-Ávila, J., and Galván-Magaña, F. (2018). Age and growth of the banded guitarfish Zapteryx exasperata (Chondrichthyes: Trygonorrhinidae). Marine and Freshwater Research 69, 66–73.
| Age and growth of the banded guitarfish Zapteryx exasperata (Chondrichthyes: Trygonorrhinidae).Crossref | GoogleScholarGoogle Scholar |
Chang, W. Y. B. (1982). A statistical method for evaluating the reproducibility of age determination. Canadian Journal of Fisheries and Aquatic Sciences 39, 1208–1210.
| A statistical method for evaluating the reproducibility of age determination.Crossref | GoogleScholarGoogle Scholar |
Colonello, J. H., Cortés, F., and Belleggia, M. (2020). Male-biased sexual size dimorphism in sharks: the narrowmouth catshark Schroederichthys bivius as case study. Hydrobiologia 847, 1873–1886.
| Male-biased sexual size dimorphism in sharks: the narrowmouth catshark Schroederichthys bivius as case study.Crossref | GoogleScholarGoogle Scholar |
Cortés, E. (2000). Life history patterns and correlations in sharks. Reviews in Fisheries Science 8, 299–344.
| Life history patterns and correlations in sharks.Crossref | GoogleScholarGoogle Scholar |
Courtney, A. J., Tonks, M. L., Campbell, M. J., Roy, D. P., Gaddes, S. W., Kyne, P. M., and O’Neill, M. F. (2006). Quantifying the effects of bycatch reduction devices in Queensland’s (Australia) shallow water eastern king prawn (Penaeus plebejus) trawl fishery. Fisheries Research 80, 136–147.
| Quantifying the effects of bycatch reduction devices in Queensland’s (Australia) shallow water eastern king prawn (Penaeus plebejus) trawl fishery.Crossref | GoogleScholarGoogle Scholar |
Courtney, A. J., Campbell, M. J., Roy, D. P., Tonks, M. L., Chilcott, K. E., and Kyne, P. J. (2008). Round scallops and square-meshes: a comparison of four codend types on the catch rates of target species and bycatch in the Queensland (Australia) saucer scallop (Amusium balloti) trawl fishery. Marine and Freshwater Research 59, 849–864.
| Round scallops and square-meshes: a comparison of four codend types on the catch rates of target species and bycatch in the Queensland (Australia) saucer scallop (Amusium balloti) trawl fishery.Crossref | GoogleScholarGoogle Scholar |
D’Alberto, B. M., Chin, A., Smart, J. J., Baje, L., White, W. T., and Simpfendorfer, C. A. (2017). Age, growth and maturity of oceanic whitetip shark (Carcharhinus longimanus) from Papua New Guinea. Marine and Freshwater Research 68, 1118–1129.
| Age, growth and maturity of oceanic whitetip shark (Carcharhinus longimanus) from Papua New Guinea.Crossref | GoogleScholarGoogle Scholar |
D’Alberto, B. M., Carlson, J. K., Pardo, S. A., and Simpfendorfer, C. A. (2019). Population productivity of shovelnose rays: inferring the potential for recovery. PLoS One 14, e0225183.
| Population productivity of shovelnose rays: inferring the potential for recovery.Crossref | GoogleScholarGoogle Scholar | 31751369PubMed |
Dulvy, N. K., Baum, J. K., Clarke, S., Compagno, L. J. V., Cortés, E., Domingo, A., Fordham, S., Fowler, S., Francis, M. P., Gibson, C., Martínez, J., Musick, J. A., Soldo, A., Stevens, J. D., and Valenti, S. (2008). You can swim but you can’t hide: the global status and conservation of oceanic pelagic sharks and rays. Aquatic Conservation 18, 459–482.
| You can swim but you can’t hide: the global status and conservation of oceanic pelagic sharks and rays.Crossref | GoogleScholarGoogle Scholar |
Dulvy, N. K., Simpfendorfer, C. A., Davidson, L. N. K., Fordham, S. V., Bräutigam, A., Sant, G., and Welch, D. J. (2017). Challenges and priorities in shark and ray conservation. Current Biology 27, R565–R572.
| Challenges and priorities in shark and ray conservation.Crossref | GoogleScholarGoogle Scholar | 28586694PubMed |
Emmons, S. M., D’Alberto, B. M., Smart, J. J., and Simpfendorfer, C. A. (2021). Age and growth of tiger shark (Galeocerdo cuvier) from Western Australia. Marine and Freshwater Research , .
| Age and growth of tiger shark (Galeocerdo cuvier) from Western Australia.Crossref | GoogleScholarGoogle Scholar |
Francis, R. I. C. C. (1990). Back-calculation of fish length: a critical review. Journal of Fish Biology 36, 883–902.
| Back-calculation of fish length: a critical review.Crossref | GoogleScholarGoogle Scholar |
Frisk, M. G., Miller, T. J., and Fogarty, M. J. (2001). Estimation and analysis of biological parameters in elasmobranch fishes: a comparative life history study. Canadian Journal of Fisheries and Aquatic Sciences 58, 969–981.
| Estimation and analysis of biological parameters in elasmobranch fishes: a comparative life history study.Crossref | GoogleScholarGoogle Scholar |
Gabry, J. T. M. (2020). bayesplot: Plotting for Bayesian Models. R package version 1.7.2. Available at https://mc-stan.org/bayesplot.
Gilman, E., Perez Roda, A., Huntington, T., Kennelly, S. J., Suuronen, P., Chaloupka, M., and Medley, P. A. H. (2020). Benchmarking global fisheries discards. Scientific Reports 10, 14017.
| Benchmarking global fisheries discards.Crossref | GoogleScholarGoogle Scholar | 32820238PubMed |
Goldman, K. J. (2005). Age and growth of elasmobranch fishes. FAO Fisheries Technical Paper 474, 76–102.
Goldman, K. J., Cailliet, G. M., Andrews, A. H., and Natanson, L. J. (2004). Age determination and validation in chondrichthyan fishes. In ‘Biology of Sharks and their Relatives’. 2nd edn. (Eds J. C. Carrier, J. A. Musick, and M. R. Heithaus.) pp. 423–450. (CRC Press: Boca Raton, FL, USA.)
Gutteridge, A. N., and Bennett, M. B. (2014). Functional implications of ontogenetically and sexually dimorphic dentition in the eastern shovelnose ray, Aptychotrema rostrata. The Journal of Experimental Biology 217, 192–200.
| Functional implications of ontogenetically and sexually dimorphic dentition in the eastern shovelnose ray, Aptychotrema rostrata.Crossref | GoogleScholarGoogle Scholar | 24072800PubMed |
Hart, N. S., Lisney, T. J., Marshall, N. J., and Collin, S. P. (2004). Multiple cone visual pigments and the potential for trichromatic colour vision in two species of elasmobranch. The Journal of Experimental Biology 207, 4587–4594.
| Multiple cone visual pigments and the potential for trichromatic colour vision in two species of elasmobranch.Crossref | GoogleScholarGoogle Scholar | 15579554PubMed |
Izzo, C., and Gillanders, B. M. (2008). Initial assessment of age, growth and reproductive parameters of the southern fiddler ray Trygonorrhina fasciata (Müller & Henle, 1841) from South Australia. Pan-American Journal of Aquatic Sciences 3, 321–327.
Jacobsen, I., Zeller, B., Dunning, M., Garland, A., Courtney, T., and Jebreen, E. J. (2018). An ecological risk Assessment of the southern Queensland east coast otter trawl fishery and river and inshore beam trawl fishery. Department of Agriculture and Fisheries, Brisbane, Qld. Available at https://www.daf.qld.gov.au/__data/assets/pdf_file/0004/1402672/Sth-QLD-Trawl-ERA-Final.pdf
Jensen, A. L. (1996). Beverton and Holt life history invariants result from optimal trade-off of reproduction and survival. Canadian Journal of Fisheries and Aquatic Sciences 53, 820–822.
| Beverton and Holt life history invariants result from optimal trade-off of reproduction and survival.Crossref | GoogleScholarGoogle Scholar |
Jones, A. A., Hall, N. G., and Potter, I. C. (2010). Species compositions of elasmobranchs caught by three different commercial fishing methods off southwestern Australia, and biological data for four abundant bycatch species. Fishery Bulletin 108, 365–381.
Kennelly, S. J. (2020). Bycatch Beknown: Methodology for jurisdictional reporting of fisheries discards–Using Australia as a case study. Fish and Fisheries 21, 1046–1066.
| Bycatch Beknown: Methodology for jurisdictional reporting of fisheries discards–Using Australia as a case study.Crossref | GoogleScholarGoogle Scholar |
Kyne, P. M. (2016). Ray conservation. In ‘Rays of the World’. Vol. 1. (Eds P. R. Last, W. T. White, M. R. de Carvalho, B. Séret, M. F. W. Stehmann, and G. J. P. Naylor.) pp. 21–24. (CSIRO Publishing: Melbourne, Vic., Australia.)
Kyne, P. M., and Bennett, M. B. (2002a). Diet of the eastern shovelnose ray, Aptychotrema rostrata (Shaw & Nodder, 1794), from Moreton Bay, Queensland, Australia. Marine and Freshwater Research 53, 679–686.
| Diet of the eastern shovelnose ray, Aptychotrema rostrata (Shaw & Nodder, 1794), from Moreton Bay, Queensland, Australia.Crossref | GoogleScholarGoogle Scholar |
Kyne, P. M., and Bennett, M. B. (2002b). Reproductive biology of the eastern shovelnose ray, Aptychotrema rostrata (Shaw Nodder, 1794), from Moreton Bay, Queensland, Australia. Marine and Freshwater Research 53, 583–589.
| Reproductive biology of the eastern shovelnose ray, Aptychotrema rostrata (Shaw Nodder, 1794), from Moreton Bay, Queensland, Australia.Crossref | GoogleScholarGoogle Scholar |
Kyne, P. M., and Stevens, J. D. (2015). Aptychotrema rostrata. The IUCN Red List of Threatened Species 2015: e.T161596A68609037. Available at
Kyne, P., Courtney, A., Campbell, M., Chilcott, K., Gaddes, S., Turnbull, C., Van Der Geest, C., and Bennett, M. (2002). An overview of the elasmobranch bycatch of the Queensland East coast trawl fishery (Australia). Northwest Atlantic Fisheries Organisation NAFO SCR Document 2, 1–11.
Kyne, P. M., Courtney, A. J., Jacobsen, I. P., and Bennett, M. B. (2016). Reproductive parameters of rhinobatid and urolophid batoids taken as by-catch in the Queensland (Australia) east coast otter-trawl fishery. Journal of Fish Biology 89, 1208–1226.
| Reproductive parameters of rhinobatid and urolophid batoids taken as by-catch in the Queensland (Australia) east coast otter-trawl fishery.Crossref | GoogleScholarGoogle Scholar | 27238204PubMed |
Kyne, P. M., Jabado, R. W., Rigby, C. L., Gore, M. A., Pollock, C. M., Herman, K. B., Cheok, J., Ebert, D. A., Simpfendorfer, C. A., and Dulvy, N. K. (2020). The thin edge of the wedge: extremely high extinction risk in wedgefishes and giant guitarfishes. Aquatic Conservation 30, 1337–1361.
| The thin edge of the wedge: extremely high extinction risk in wedgefishes and giant guitarfishes.Crossref | GoogleScholarGoogle Scholar |
Last, P. R., and Stevens, J. D. (2009). ‘Sharks and Rays of Australia.’ (CSIRO Publishing: Melbourne, Vic., Australia)
Last, P., Naylor, G., Séret, B., White, W., de Carvalho, M., and Stehmann, M. (2016). ‘Rays of the World.’ (CSIRO Publishing: Melbourne, Vic., Australia)
McGilvray, J. G., Mounsey, R. P., and MacCartie, J. (1999). The AusTED II, an improved trawl efficiency device 1. Design theories. Fisheries Research 40, 17–27.
| The AusTED II, an improved trawl efficiency device 1. Design theories.Crossref | GoogleScholarGoogle Scholar |
Meynecke, J.-O., and Lee, S. Y. (2011). Climate-coastal fisheries relationships and their spatial variation in Queensland, Australia. Fisheries Research 110, 365–376.
| Climate-coastal fisheries relationships and their spatial variation in Queensland, Australia.Crossref | GoogleScholarGoogle Scholar |
Natanson, L. J., Casey, J. G., and Kohler, N. E. (1995). Age and growth estimates for the dusky shark, Carcharhinus obscurus, in the western North Atlantic Ocean. Fishery Bulletin 93, 116–126.
Officer, R. A., Gason, A. S., Walker, T. I., and Clement, J. G. (1996). Sources of variation in counts of growth increments in vertebrae from gummy shark (Mustelus antarcticus, and school shark, Galeorhinus galeus): implications for age determination. Canadian Journal of Fisheries and Aquatic Sciences 53, 1765–1777.
| Sources of variation in counts of growth increments in vertebrae from gummy shark (Mustelus antarcticus, and school shark, Galeorhinus galeus): implications for age determination.Crossref | GoogleScholarGoogle Scholar |
Pardo, S. A., Cooper, A. B., and Dulvy, N. K. (2013). Avoiding fishy growth curves. Methods in Ecology and Evolution 4, 353–360.
| Avoiding fishy growth curves.Crossref | GoogleScholarGoogle Scholar |
Pauly, D. (1980). On the interrelationships between natural mortality, growth parameters, and mean environmental temperature in 175 fish stocks. ICES Journal of Marine Science 39, 175–192.
| On the interrelationships between natural mortality, growth parameters, and mean environmental temperature in 175 fish stocks.Crossref | GoogleScholarGoogle Scholar |
Pears, R. J., Morison, A. K., Jebreen, E. J., Dunning, M. C., Pitcher, C. R., Courtney, A. J., Houlden, B., and Jacobsen, I. P. (2012). Ecological risk assessment of the East Coast Otter Trawl Fishery in the Great Barrier Reef Marine Park: technical report. Great Barrier Reef Marine Park Authority, Townsville, Qld, Australia. Available at http://elibrary.gbrmpa.gov.au/jspui/bitstream/11017/1148/1/ECOTF_ERA_Technical_web.pdf
Pierce, S. J., and Bennett, M. B. (2009). Validated annual band-pair periodicity and growth parameters of blue-spotted maskray Neotrygon kuhlii from south-east Queensland, Australia. Journal of Fish Biology 75, 2490–2508.
| Validated annual band-pair periodicity and growth parameters of blue-spotted maskray Neotrygon kuhlii from south-east Queensland, Australia.Crossref | GoogleScholarGoogle Scholar | 20738504PubMed |
Robins, J. B., and McGilvray, J. G. (1999). The AusTED II, an improved trawl efficiency device 2. Commercial performance. Fisheries Research 40, 29–41.
| The AusTED II, an improved trawl efficiency device 2. Commercial performance.Crossref | GoogleScholarGoogle Scholar |
Robins-Troeger, J. B. (1994). Evaluation of the Morrison soft turtle excluder device: prawn and bycatch variation in Moreton Bay, Queensland. Fisheries Research 19, 205–217.
| Evaluation of the Morrison soft turtle excluder device: prawn and bycatch variation in Moreton Bay, Queensland.Crossref | GoogleScholarGoogle Scholar |
Rolim, F. A., Siders, Z. A., Caltabellotta, F. P., Rotundo, M. M., and Vaske-Júnior, T. (2020). Growth and derived life-history characteristics of the Brazilian electric ray Narcine brasiliensis. Journal of Fish Biology 97, 396–408.
| Growth and derived life-history characteristics of the Brazilian electric ray Narcine brasiliensis.Crossref | GoogleScholarGoogle Scholar | 32402101PubMed |
Rowsell, N., and Davies, J. (2012). At-sea observation of the stout whiting fishery 2009–10. Technical report. Department of Agriculture, Fisheries and Forestry, Brisbane, Qld, Australia. Available at http://era.daf.qld.gov.au/id/eprint/6552/1/FOP%20Stout%20Whiting%20Report%202012%20final.pdf
Simpfendorfer, C. A., and Dulvy, N. K. (2017). Bright spots of sustainable shark fishing. Current Biology 27, R97–R98.
| Bright spots of sustainable shark fishing.Crossref | GoogleScholarGoogle Scholar | 28171764PubMed |
Simpfendorfer, C. A., Chidlow, J., McAuley, R., and Unsworth, P. (2000). Age and growth of the whiskery shark, Furgaleus macki, from southwestern Australia. Environmental Biology of Fishes 58, 335–343.
| Age and growth of the whiskery shark, Furgaleus macki, from southwestern Australia.Crossref | GoogleScholarGoogle Scholar |
Smart, J. J. (2020) BayesGrowth: Estimate fish growth using MCMC analysis. R package version 0.3.0. Available at https://github.com/jonathansmart/BayesGrowth.
Smart, J. J., and Grammer, G. L. (2021). Modernising fish and shark growth curves with Bayesian length-at-age models. PLoS One 16, e0246734.
| Modernising fish and shark growth curves with Bayesian length-at-age models.Crossref | GoogleScholarGoogle Scholar | 33556124PubMed |
Smart, J. J., Harry, A. V., Tobin, A. J., and Simpfendorfer, C. A. (2013). Overcoming the constraints of low sample sizes to produce age and growth data for rare or threatened sharks. Aquatic Conservation 23, 124–134.
| Overcoming the constraints of low sample sizes to produce age and growth data for rare or threatened sharks.Crossref | GoogleScholarGoogle Scholar |
Smart, J. J., Chin, A., Tobin, A. J., and Simpfendorfer, C. A. (2016). Multimodel approaches in shark and ray growth studies: strengths, weaknesses and the future. Fish and Fisheries 17, 955–971.
| Multimodel approaches in shark and ray growth studies: strengths, weaknesses and the future.Crossref | GoogleScholarGoogle Scholar |
Stan Development Team (2020). RStan: the R interface to Stan. R package version 2.21.2. http://mc-stan.org/
Stevens, J. D., Bonfil, R., Dulvy, N. K., and Walker, P. A. (2000). The effects of fishing on sharks, rays, and chimaeras (chondrichthyans), and the implications for marine ecosystems. ICES Journal of Marine Science 57, 476–494.
| The effects of fishing on sharks, rays, and chimaeras (chondrichthyans), and the implications for marine ecosystems.Crossref | GoogleScholarGoogle Scholar |
Then, A. Y., Hoenig, J. M., Hall, N. G., and Hewitt, D. A. (2015). Evaluating the predictive performance of empirical estimators of natural mortality rate using information on over 200 fish species. ICES Journal of Marine Science 72, 82–92.
| Evaluating the predictive performance of empirical estimators of natural mortality rate using information on over 200 fish species.Crossref | GoogleScholarGoogle Scholar |
Vehtari, A., Gabry, J., Magnusson, M., Yao, Y., Bürkner, P.-C., Paananen, T., and Gelman, A. (2020) loo: Efficient leave-one-out cross-validation and WAIC for Bayesian models. R package version 2.4.1. https://mc-stan.org/loo/
Wang, N., Courtney, A. J., Campbell, M. J., and Yang, W.-H. (2020). Quantifying long-term discards from Queensland’s (Australia) east coast otter trawl fishery. ICES Journal of Marine Science 77, 680–691.
| Quantifying long-term discards from Queensland’s (Australia) east coast otter trawl fishery.Crossref | GoogleScholarGoogle Scholar |
White, J., Simpfendorfer, C. A., Tobin, A. J., and Heupel, M. R. (2014). Age and growth parameters of shark-like batoids. Journal of Fish Biology 84, 1340–1353.
| Age and growth parameters of shark-like batoids.Crossref | GoogleScholarGoogle Scholar | 24702252PubMed |
Wueringer, B. E., Tibbetts, I. R., and Whitehead, D. L. (2009). Ultrastructure of the ampullae of Lorenzini of Aptychotrema rostrata (Rhinobatidae). Zoomorphology 128, 45–52.
| Ultrastructure of the ampullae of Lorenzini of Aptychotrema rostrata (Rhinobatidae).Crossref | GoogleScholarGoogle Scholar |
Zhou, S., Griffiths, S. P., and Miller, M. (2009). Sustainability assessment for fishing effects (SAFE) on highly diverse and data-limited fish bycatch in a tropical prawn trawl fishery. Marine and Freshwater Research 60, 563–570.
| Sustainability assessment for fishing effects (SAFE) on highly diverse and data-limited fish bycatch in a tropical prawn trawl fishery.Crossref | GoogleScholarGoogle Scholar |
Zhou, S., Yin, S., Thorson, J. T., Smith, A. D. M., and Fuller, M. (2012). Linking fishing mortality reference points to life history traits: an empirical study. Canadian Journal of Fisheries and Aquatic Sciences 69, 1292–1301.
| Linking fishing mortality reference points to life history traits: an empirical study.Crossref | GoogleScholarGoogle Scholar |
Zhou, S., Daley, J., Fuller, M., Bulman, C., Hobday, A., Courtney, T., Ryan, P., and Ferrel, D. (2013). ERA extension to assess cumulative effects of fishing on species. Final report on FRDC Project 2011/029, Canberra, ACT, Australia. Available at http://frdc.com.au/research/Final_Reports/2011-029-DLD.pdf
Zhou, S., Buckworth, R. C., Miller, M., and Jarrett, A. (2015). A SAFE analysis of bycatch in the Joseph Boneparte Gulf fishery for red-legged banana prawns. CSIRO Oceans and Atmosphere Flagship, Brisbane, Qld, Australia. Available at http://www.afma.gov.au/wp-content/uploads/2014/02/JBG-SAFE-Report-2015_05_21-final.pdf
Zhou, S., Hobday, A. J., Dichmont, C. M., and Smith, A. D. M. (2016). Ecological risk assessments for the effects of fishing: a comparison and validation of PSA and SAFE. Fisheries Research 183, 518–529.
| Ecological risk assessments for the effects of fishing: a comparison and validation of PSA and SAFE.Crossref | GoogleScholarGoogle Scholar |