Non-additive effects on plant mixtures flammability in a tropical mountain ecosystem
Helena Roland
A
B
C
D
E
F
Abstract
Consumability, a component of flammability, describes how well plants burn and may be influenced by species traits and climate change. However, knowledge gaps remain regarding how species mixtures interact and whether non-additive effects are mediated by functional traits and diversity.
This study examined the consumability of species mixtures in Brazil’s tropical montane grasslands (campos de altitude), focusing on traits and species interactions.
Laboratory tests measured remaining biomass (a proxy for consumability) in monospecific and three-species mixtures for seven species. Traits like specific leaf area (SLA), leaf dry matter content (LDMC), fuel moisture at ignition (FMCig) and maximum combustion rate (MCR) were assessed, along with functional diversity, to understand their influence on consumability.
Consumability in species mixtures differed from predictions based on individual species values, indicating non-additive effects. Leptostelma maximum, Pleroma hospita and Chusquea pinifolia, despite low or medium individual consumability, contributed most to mixture consumability. Higher SLA reduced consumability, whereas higher MCR, LDMC and functional index FRich increased it.
Our results suggest that low-consumability species like L. maximum may reduce fire severity, offering opportunities for fire management in the campos de altitude.
These findings highlight the importance of considering species interactions in mixtures and identifying traits that shape plant community flammability.
Keywords: Brazil, campos de altitude, climate change, consumability, ecosystem process, fire management, fire regimes, fire severity, species interactions, trait-based ecology.
Introduction
The maintenance of many ecosystems around the world depends on fire events (Bond and Keeley 2005; Bowman et al. 2009). Fire shapes plant communities by favoring species capable of surviving or regenerating after fire (Oliveras and Malhi 2016; Pausas et al. 2017). Additionally, plant biomass combustion by fire is a significant ecological process, impacting the carbon balance of terrestrial ecosystems, nutrient cycling and global climate (Oliveras et al. 2013; Scott 2014).
Grasslands support most of the fires on Earth, contributing to the majority of burned areas and fire-related emissions (van der Werf et al. 2017; Stevens et al. 2022). Although fire effects in savannas are well studied (Oliveras and Malhi 2016), little is known about fire regimes in tropical montane grasslands, especially under the pressures of climate change. In the campos de altitude, a tropical grassland vegetation type located above 1800 m in southeastern Brazil (Safford 1999), fire acts as selective pressure (Instituto Chico Mendes de Conservação da Biodiversidade (ICMBio) 2023), spreading through the litter and herbaceous layers during the dry seasons (Aximoff and Rodrigues 2011). However, future climate with rising temperatures and increased aridity (Lyra et al. 2018) may result in drier vegetation and more severe fire regimes. Fires are already a major concern for conservation managers, highlighting the need for further research on fire severity in montane grasslands (Safford 2001). One of the key factors determining fire severity is fire behavior, which refers to how a fire spreads and interacts with the environment during a fire event (Rego et al. 2021). In a context where most wildfires in campos de altitude are ignited by human activity (Instituto Chico Mendes de Conservação da Biodiversidade (ICMBio) 2023) and human-ignited fires tend to exhibit more extreme behavior (Hantson et al. 2022), understanding fire behavior is crucial for developing strategies to manage extreme fires amid growing human and climate pressures.
As plants serve as the primary fuel for sustaining fires, variations in their traits can result in considerable changes in fire behavior within a plant community (Cardoso et al. 2018). Those plant traits that describe a plant’s propensity to ignite and spread fire are called flammability traits (Pérez-Harguindeguy et al. 2013). Flammability can be divided into four components: (1) ignitability (i.e. how easily a plant ignites), (2) combustibility (i.e. the intensity of plant combustion), (3) sustainability (i.e. the duration of fire within plant tissue), and (4) consumability (i.e. the proportion of plant material consumed) (Anderson 1970; Martin et al. 1993). These different components of flammability have distinct implications for fire behavior and ecological responses (Pausas et al. 2017). For example, ignitability can be related to the rate of fire spread, whereas consumability is more connected to fire severity, often measured as the above- and below-ground organic matter consumed by fire (Gill and Zylstra 2005; Keeley 2009). Even though these four components are considered separate aspects of flammability, they can influence each other, but the exact nature of these relationships remains poorly understood. For instance, ignitability may be positively related to combustibility (Pausas et al. 2017; Boving et al. 2023), or these variables may not be related at all (Krix et al. 2019). Given the complexity and interrelated nature of these components, it becomes particularly important to focus on specific materials that serve as primary fuels in natural fires. With this perspective, measurements of the flammability of leaf material have additional importance because leaves are the main fuel for surface fires in grasslands (Murray et al. 2013; Rego et al. 2021).
Interspecific differences in leaf flammability may be related to leaf chemical (e.g. volatile compounds) and morphological (e.g. specific leaf area – SLA, and leaf dry matter content – LDMC) leaf traits (Pausas et al. 2017). For example, SLA is known to be an important determinant of the time to ignition of leaves (Murray et al. 2013; Grootemaat et al. 2015). Higher SLA combined with lower LDMC is linked to lower leaf density, making the leaves more permeable and allowing heat and oxygen to penetrate more easily, thus speeding up ignition (Grootemaat et al. 2015). LDMC can also be a good predictor of whole shoot flammability (Alam et al. 2020). Leaf traits can also affect flammability at the community level, influencing, for instance, the flammability of litter fuels (Parsons et al. 2015). Litter fuel, i.e. the layer of dead organic material that falls on the soil surface, usually includes a combination of leaves from various species. In an experiment using leaf mixtures of different plant species, Parsons et al. (2015) showed that SLA of individual species could be scaled up to predict combustibility at the community level. Sometimes, however, antagonistic or synergistic interactions among species can make it difficult to estimate community-level responses from trait measurements taken from individual species (de Magalhães and Schwilk 2012; van Altena et al. 2012; Della Rocca et al. 2018). In those cases, non-additive effects (i.e. effects not predicted from the sum of the effect of single species) may be promoted by functional diversity, such as functional richness and divergence between the traits of coexisting species (Tilman et al. 1997; Finerty et al. 2016). Higher functional diversity increases the probability of complementary use of resources, facilitation, or interference (Petchey et al. 2004; Hooper et al. 2005; Spehn et al. 2005). Therefore, when burned together, plants with different levels of flammability may exhibit two alternative types of effects: (1) additive effects, where the flammability of the mixture is the simple averaged flammability of the individual species; or (2) non-additive effects, where species with high or low flammability dominate the burning, so that the flammability of the mixture is higher or lower than that expected based only on the average flammability of each individual species (de Magalhães and Schwilk 2012; van Altena et al. 2012; Della Rocca et al. 2018). To the best of our knowledge, our study is the first to analyze flammability in monospecific versus mixed-species leaf mixtures in tropical mountainous environments. Consequently, it sheds light on the mechanisms that control ecosystem processes related to biomass burning in these ecosystems, such as the role of trait distribution and functional diversity in flammability ecosystem processes.
Here, we measured two leaf morphological traits (SLA and LDMC) and two flammability traits (Live Fuel Moisture Content at Ignition – LFMCig and Maximum Combustion Rate – MCR; Table 1) in seven species to assess how these traits and their functional diversity influence biomass consumability in species mixtures in a laboratory-based experiment. Consumability was chosen because it reflects the amount of biomass consumed during a fire, which is linked to fire severity. We examined the contribution of different species to leaf mixture consumability and predicted how changes in plant community composition might affect fire behavior in the campos de altitude. Specifically, we hypothesized: (H1) non-additive effects in community flammability, with species of greater consumability dominating mixture flammability (de Magalhães and Schwilk 2012; van Altena et al. 2012); (H2) consumability is explained by morphological (SLA, LDMC), ignitability (LFMCig) and combustibility (MCR) traits: (H2a) SLA positively correlates with consumability as higher SLA facilitates heat and oxygen penetration within the leaf; (H2b) LDMC positively correlates with consumability, as higher LDMC indicates more biomass available for combustion; (H2c) LFMCig has a positive relationship with consumability, because the higher the LFMCig, the greater the ignitability and, consequently, there is more time for the biomass to be consumed; (H2d) MCR positively correlates with consumability owing to higher intensity of biomass loss. Alternatively, we could find (H3) no direct relationship between individual traits and consumability, but instead a positive relationship between overall functional diversity and consumability, suggesting that non-additive effects are driven by functional diversity between these four traits.
Full name | Abbr. | Unit | Meaning | |
---|---|---|---|---|
Specific leaf area | SLA | mm2 mg−1 | One-sided leaf area divided by its dry mass. Higher values (i.e. lower mass per unit area) can facilitate heat and oxygen penetration within the leaf, which can lead to greater biomass consumption (Grootemaat et al. 2015) | |
Leaf dry matter content | LDMC | % | Leaf dry mass divided by its water-saturated fresh mass; higher values indicate more biomass available for combustion, which can lead to greater biomass consumption (Alam et al. 2020) | |
Live fuel moisture content at ignition | LFMCig | % | Live moisture content of the fuel at the ignition point. Higher values indicate that ignition occurs with higher moisture content, which means higher ignitability. Higher ignitability can mean more time available for the biomass to be consumed (Cardoso et al. 2018) | |
Maximum combustion rate | MCR | g s−1 | Rate of biomass loss during combustion, determined by fitting a linear model to mass variation over time. It reflects how quickly fuel is consumed. Higher values indicate that biomass is lost more rapidly during burning, potentially leading to greater biomass consumption (Simpson et al. 2016; Cardoso et al. 2018) | |
Remaining biomass | – | g | Refers to the biomass left after combustion, here used as a proxy for consumability. By burning leaf species biomass, we observed the final biomass remaining post-combustion. Lower remaining biomass indicates higher consumability, as more biomass was consumed during the fire | |
Functional richness | FRic | – | Functional diversity index representing the range of different functional traits within a community. Greater functional richness could positively affect consumability by promoting trait complementarity, increasing the likelihood of mixtures containing species with varying levels of ignitability and combustibility (Petchey et al. 2004; Hooper et al. 2005; Spehn et al. 2005) | |
Functional divergence | FDiv | – | Functional diversity index representing the dissimilarity among species in trait space. It could positively affect consumability by increasing trait variation within the community, leading to a greater range of ignitability and combustibility (Petchey et al. 2004; Hooper et al. 2005; Spehn et al. 2005) |
Methodology
Study area
We conducted plant community sampling and collected plant material for trait measurements in the campos de altitude of Itatiaia National Park, Rio de Janeiro, Brazil (22°22′37″S, 44°42′28″W; 2400 m above sea level). This area features a mean annual temperature of 14.4°C, annual rainfall of 2200 mm and a distinct seasonal climate (Segadas-Vianna and Dau 1965; Safford 1999). It experiences wet summers (December to February) and dry winters (June to August), with winter precipitation under 50 mm per month and temperatures often below freezing. The region has ~200 fog events and 56 frost days annually (Segadas-Vianna and Dau 1965). Campos de altitude is a fire-influenced ecosystem (Hardesty et al. 2005; Instituto Chico Mendes de Conservação da Biodiversidade (ICMBio) 2023), with predominantly anthropogenic fires, although lightning-induced fires can occur in the rainy season (Instituto Chico Mendes de Conservação da Biodiversidade (ICMBio) 2023). Fires, which range in intensity but are mostly surface fires, occur every 2–15 years, mainly during the dry season (July to mid-October) (Aximoff and Rodrigues 2011; Instituto Chico Mendes de Conservação da Biodiversidade (ICMBio) 2023). The vegetation is a mosaic of various life forms, including grasses, sedges, bamboos and small trees, with dominant species such as Cortaderia modesta, Machaerina ensifolia and Chusquea sp. (Instituto Chico Mendes de Conservação da Biodiversidade (ICMBio) 2023). These species resprout quickly after fires, and the first two also exhibit fire-induced flowering (Brade 1956).
Field sampling and leaf morphological traits
We selected seven different species (Table 2), including the most frequent: Cortaderia modesta, Machaerina ensifolia and Chusquea pinifolia, as well as other species representing a variety of relative frequencies and life forms. The relative frequency of the species in the study area was measured as described in Matos et al. (2021). In short, we established 12 transects of 100 min at the field site, and for each transect, we laid out 1-m2 quadrats at 3 m intervals (300 m2 area sampled). Then, we counted all angiosperms rooted inside each plot, plus overhanging leaves or shoots. Relative frequency was then calculated as the number of sampling units where a certain species occurred divided by the total number of sampling units (N = 300). For simplicity, we refer to each species by its generic name (Table 3).
Specie | Life form | Relative frequency (%) | |
---|---|---|---|
Chusquea pinifolia (Nees) Nees | Bamboo | 19.33 | |
Cortaderia modesta (Döll.) Hack | Graminoid | 80.00 | |
Eryngium glaziovianum Urb. | Herbaceous (rosette) | 49.00 | |
Hypochaeris lutea (Vell.) Britton | Herbaceous | 1.67 | |
Leptostelma maximum D.Don | Herbaceous | 8.33 | |
Machaerina ensifolia (Boeckeler) T.Koyama | Graminoid | 83.67 | |
Pleroma hospita Cogn. | Shrub | 47.33 |
Species | SLA (mm2 mg−1) | LDMC (%) | LFMCig (%) | MCR (g s−1) | Remaining biomass (g) | Average consumability contribution | |
---|---|---|---|---|---|---|---|
Chusquea | 11.32 ± 0.96B | 53.88 ± 3.74A | 101.10 ± 13.60B | 0.23 ± 0.13A | 0.15 ± 0.05AB | 0.02 ± 0.01C | |
Cortaderia | 6.65 ± 1.97D | 42.49 ± 2.81B | 122.00 ± 53.80AB | 1.08 ± 0.24B | 0.10 ± 0.03AC | 0.05 ± 0.03AB | |
Eryngium | 7.08 ± 1.18CD | 32.17 ± 2.22C | 158.50 ± 28.50A | 0.24 ± 0.13A | 0.10 ± 0.01AC | 0.05 ± 0.02B | |
Hypochaeris | 34.8 ± 9.63A | 16.17 ± 3.52D | – | 0.06 ± 0.04C | 0.22 ± 0.04B | 0.04 ± 0.02B | |
Leptostelma | 21.36 ± 16.57AB | 15.96 ± 6.56D | 190.90 ± 77.50A | 0.13 ± 0.11AC | 0.19 ± 0.06B | 0.02 ± 0.02C | |
Machaerina | 3.67 ± 0.29E | 34.57 ± 3.56C | 42.09 ± 12.80C | 0.97 ± 0.28B | 0.07 ± 0.02C | 0.09 ± 0.03A | |
Pleroma | 8.64 ± 1.34C | 39.46 ± 1.60B | 141.00 ± 13.80A | 0.55 ± 0.19D | 0.15 ± 0.04AB | 0.02 ± 0.02C |
Values of the mean ± s.d. per species for Specific leaf area (SLA), Leaf dry matter content (LDMC), Live fuel moisture content at the point of ignition (LFMCig), Maximum combustion rate (MCR), Remaining biomass and the Average consumability contribution for each species. Values followed by different letters indicate significant differences between means (P < 0.05).
To measure SLA and LDMC, we collected branches (or whole individuals for herbaceous species) of 10 individuals per species and rehydrated them for 2 h (Matos et al. 2021). Next, we sampled five mature leaves (excluding the petiole) per individual to determine the water-saturated fresh mass with a precision scale (0.01 g). Leaf samples were then scanned (at 300 dpi resolution) to obtain leaf area (LA) using Image J software version 1.48. Finally, leaves were oven-dried at 60°C for 72 h to obtain leaf dry mass. We then used LA, fresh and dry mass to obtain SLA and LDMC .
Flammability laboratory experiments
We conducted different tests to measure LFMCig, MCR and remaining biomass, using separate samples for each one, allowing us to meet the specific objectives of each test. To determine LFMCig, we conducted an ignition test following the methodology described by Cardoso et al. (2018). We could not test Hypochaeris owing to a lack of sufficient individuals in the study area. We collected leaf samples from 100 individuals of each species and rehydrated them for at least 2 h. We then selected subsets of 10 leaf samples from different individuals and weighed them daily. The ignition test involved holding a lighted match to the center of each leaf for 5 s until half of the samples (five) ignited. We manually held the leaves and conducted the ignition in a closed environment within the research station at Itatiaia National Park, ensuring no air currents interfered with the process. After ignition, we placed the samples in an oven to determine their dry mass, and calculated LFMCig .
To determine the MCR, we followed the methodology of Simpson et al. (2016) and Cardoso et al. (2018). We collected branch samples from 10 individuals per species and standardized their initial mass to 9.95–10.5 g, as larger biomass facilitated the measurement of the mass loss rate over time. However, for smaller species (Eryngium, Hypochaeris, Leptostelma), standardization at 10 g was not feasible, resulting in masses ranging from 0.61 to 8.33 g. We dried all samples at 60°C for 3 days before testing. Each sample was then burned on a precision balance, ignited directly with a match and mass loss recorded via video. We conducted the experiment in a fume hood to ensure constant conditions. We recorded mass variation every 0.2 s using Free Video to JPG Converter. We plotted mass variation over time and fitted the resulting sigmoidal curve using the drm function in the drc package (Ritz et al. 2015). The inflection point of the curve indicates when mass loss occurred at the highest rate. Using this reference, we fitted a linear model to 3 s of data on either side of this point, with the slope representing the plant MCR.
We conducted a third test to determine the biomass remaining after combustion (proxy for consumability) of monospecific and mixed-species leaf mixtures. We conducted the burn in a JUNG 1000°C muffle furnace (model 0910). Conducting the experiment inside the furnace provided controlled and consistent conditions for biomass consumption. We dried all samples at 60°C for 3 days before testing. As consumability depends on the biomass ultimately burned, we used dry material to standardize conditions and minimize variability in fuel moisture content over the course of the experiment. To optimize temperature and burning time, we performed a pilot test (Supplementary Fig. S1) with 1 g of leaf material from two species at 300, 400 and 500°C for 30, 60, 120 and 180 min. Our goal was to find a time-temperature combination that distinguished species differences without excessive burning. One of the species used in the pilot study, Baccharis, was not included in the main experiment owing to limited material. The decision to include Baccharis in the pilot does not affect the validity of the results, as the focus of the pilot was on testing the experimental conditions rather than the specific species. We found that 400°C for 30 min best highlighted species differences, whereas 300°C also showed differences but was less realistic for surface fires. Thus, we selected 400 and 30 min as our experimental parameters (see Supplementary Material S1). We assessed consumability with five replicates per species, using 0.9995–1.0005 g of initial biomass. For three-species mixtures, we maintained total biomass within this range, allocating ~0.3332–0.3335 g per species. The decision to use 0.9995–1.0005 g allowed us to include rare species with limited biomass. To ensure consistency, we cut leaves into smaller pieces before burning, as graminoid leaves often exceed 1.0 g. This process was uniformly applied to both single-species and mixed-species samples to standardize biomass, minimize structural bias and ensure comparability between treatments. We placed each replicate in identical aluminium containers (Ø 9 cm, height 2.5 cm, volume 80 mL). We chose three-species combinations to include the three most frequent species (Cortaderia, Machaerina and Chusquea) identified in the Integrated Fire Management Plan of Itatiaia National Park (Instituto Chico Mendes de Conservação da Biodiversidade (ICMBio) 2023). In total, we performed 35 three-species combinations with five replicates each.
We also calculated the average contribution for each species in a mixture, following the method of de Magalhães and Schwilk (2012). This calculation represents how closely the consumability of a mixture matches the consumability of each species. We first determined the difference in consumability between a species and all mixtures containing that species. We then calculated the average of these differences to obtain a representative value for each species. This value indicates the influence of each species on the overall consumability of the mixtures. The smaller the absolute value for a given species, the more that species dominates the consumability of the mixtures in which it occurs.
Data analysis
To assess variability in species consumability, we first conducted the Shapiro–Wilk and Levene tests to check for normality and homogeneity of the data. We then performed ANOVA to determine if there were significant differences among species. When significant differences were found, we used the Tukey test for multiple comparisons. For traits that did not meet the assumptions of normality and homogeneity even after Box-Cox transformation, we used the Kruskal–Wallis and Dunn’s tests for multiple comparisons to determine species differences in SLA, LDMC, LFMC, MCR, remaining biomass and Average Consumability Contribution.
To test for the presence of non-additive effects, we employed a paired t-test to compare the expected values for the remaining biomass of the mixtures (i.e. based on the remaining biomass values of their constituent species) with the observed values of the remaining biomass obtained from burning the mixtures (see Fig. 1).
Scheme of the consumability experiment for determining expected and observed values of remaining biomass.
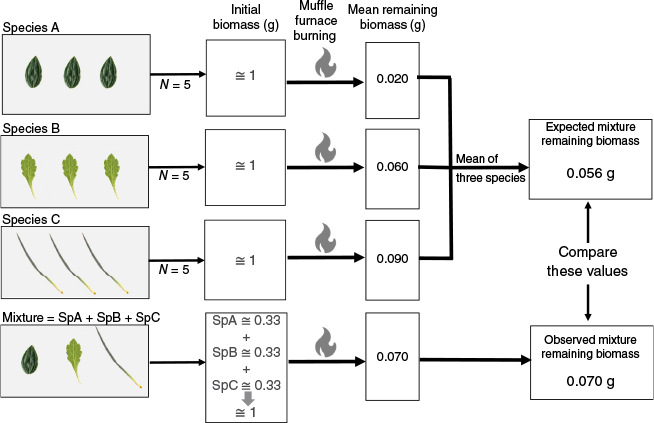
We estimated functional diversity for each of the 35 mixtures using functional diversity indices. Specifically, we measured functional richness (FRic), which indicates the volume of trait space occupied and functional divergence (FDiv), which quantifies how trait values diverge within this space. They were calculated using the FRic and FDiv functions from the FD R package (Laliberté and Legendre 2010). To link functional traits with consumability, we ran linear regressions between each trait’s mean value per mixture and the remaining biomass. We also assessed the relationship between functional diversity indices (functional richness, functional divergence) and consumability using linear regressions between these indices and the remaining biomass for each mixture. All analyses were conducted in the R environment (R version 4.3.1, R Core Team 2023).
Results
Leaf morphological and flammability traits
We observed significant interspecific differences in the leaf morphological (SLA, LDMC) and flammability (LFMCig, MCR) traits, remaining biomass and average consumability contribution across the seven studied species (Table 2).
Remaining biomass of monospecific leaf mixtures also varied across species (Table 2, Fig. 2a). For example, Leptostelma and Hypochaeris showed the highest values, whereas Machaerina had the lowest amount of remaining biomass.
Results of the consumability experiments and comparison between the observed remaining biomass. Results of the consumability experiments. (a) Remaining biomass (g) of monospecific leaf mixtures for each of the seven species evaluated: Chusquea pinifolia, Cortaderia modesta, Eryngium glaziovianum, Hypochaeris lutea, Leptostelma maximum, Machaerina ensifolia, Pleroma hospita. The green dots in the first boxplot represent the individual data points. (b) Comparison between the observed remaining biomass of the 35 three-species leaf mixtures and the expected values based on the individual constituent species remaining biomass.
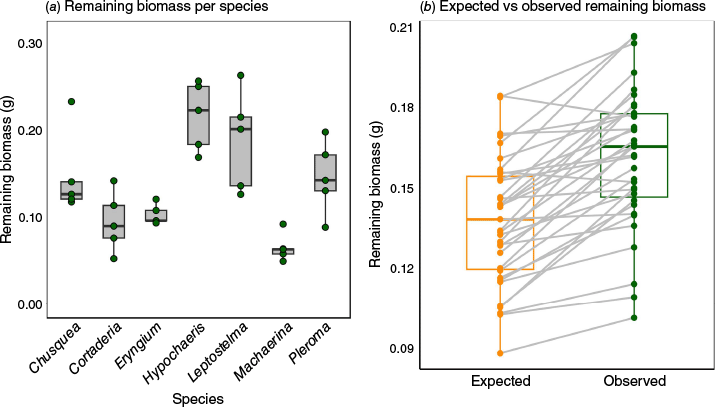
Expected vs observed biomass and average species contribution
Overall, as hypothesized (H1), the observed remaining biomass of the three-species mixtures differed from the expected values calculated based on the individual remaining biomass species values (Fig. 2b). The results of the paired t-test indicated a mean difference of 0.0235 g (t = 8.0717, P = 2.70 × 10−9) between observed and expected values. Additionally, contrary to our hypothesis (H1), in 33 (out of 35) mixtures, the observed mixture remaining biomass was higher than the predictions based on the individual constituent species values and species with high consumability – M. ensifolia (0.0882) and Cortaderia (0.544) – had the lowest average contributions to mixtures’ consumability. The species that exhibited the largest contributions to mixtures’ consumability were those with low and medium consumability – Leptostelma (0.0208), Pleroma (0.0224) and Chusquea (0.0231).
Relationship between functional diversity and consumability
As hypothesized (H2), leaf morphological and flammability traits showed significant relationships with consumability (Fig. 3). Contrary to our expectations in H2a, SLA exhibited the strongest positive relationship with remaining biomass (R2 = 0.48, P = 0.02; Fig. 3a), indicating a negative relationship with consumability. According to our hypotheses (H2b and H2d), both LDMC (R2 = 0.19, P = 0.03; Fig. 3b) and MCR (R2 = 0.33, P = 0.002; Fig. 3d) demonstrated negative relationships with remaining biomass, which translates to a positive relationship with consumability. Regarding functional diversity indices, only FRic displayed a significant negative relationship with remaining biomass, indicating a positive relationship with consumability (Fig. 3e), thus partially supporting our H3.
Relationships between mean leaf functional traits per mixture and functional diversity with remaining mixture biomass (g). The statistically significant linear regressions are in green: (a) Specific leaf area (SLA); (b) Leaf dry matter content (LDMC); (c) Live fuel moisture in the point of ignition (LFMCig); (d) Maximum combustion rate (MCR); (e) Functional Richness (FRic); and (f) Functional divergence (FDiv). See supplementary material for the complete statistical results (Supplementary Table S1: intercept, slope, residual s.e., degrees of freedom).
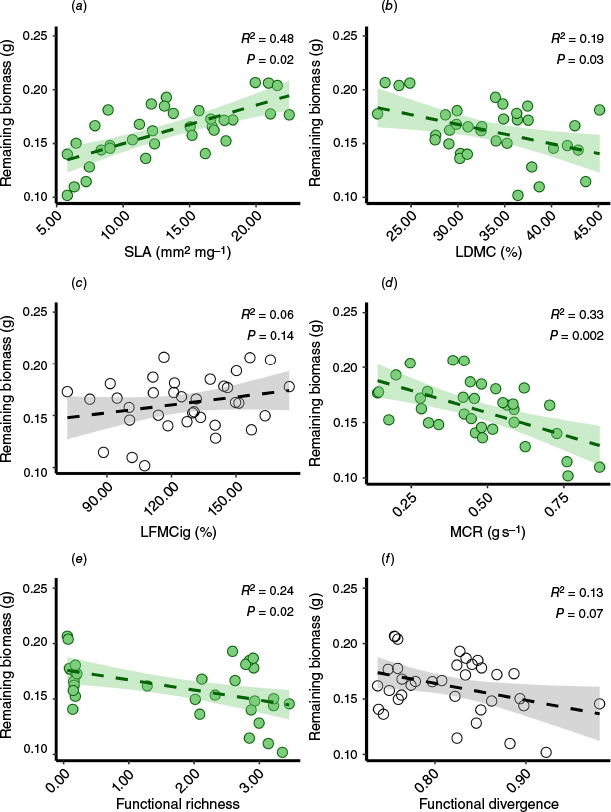
Discussion
With the increased risk of fire events with higher severity worldwide, and in the campos de altitude in particular (Assis and Mattos 2016), it is essential to determine how fire severity is influenced by species traits and their functional diversity. Our results show a non-additive effect of species traits on leaf consumability in mixtures, meaning community consumability cannot be predicted from the consumability of individual species. Species with higher consumability did not dominate the burning process as expected. Additionally, traits such as SLA, LDMC and MCR, as well as the functional index FRic, were important to explain the consumability of the leaf mixtures. We discuss these findings in the context of community assembly, ecosystem processes and potential implications for fire severity in the campos de altitude under climate change.
Non-additivity and the influence of low and high-consumability species
As hypothesized (H1), our results indicate non-additivity in consumability when species are mixed, showing that species interactions significantly affect consumability differently from individual species’ properties. Contrary to H1, high-consumability species contributed less to total mixture consumability, contradicting findings from previous studies (de Magalhães and Schwilk 2012; van Altena et al. 2012). Despite the local biodiversity managers’ belief that more abundant species with higher flammability drive fire behavior (Instituto Chico Mendes de Conservação da Biodiversidade (ICMBio) 2023), their dominant effect on fire severity may be related to their higher relative abundance rather than non-additive effects. Instead, species with low to medium consumability, such as Leptostelma, Pleroma and Chusquea, played a significant role in the amount of biomass burned. Consequently, the observed consumability of the leaf mixtures was lower than that predicted by the sum of the consumability of each individual species. This result suggests that, although not dominant, these species may play a crucial role in modulating fire severity in the campos de altitude.
The role of traits and functional diversity in biomass consumability
Contrary to our hypothesis, SLA showed a negative relationship with consumability, whereas LDMC and MCR showed a positive relationship. The combination of low SLA and high LDMC means higher leaf densities, suggesting that leaf density is positively related to consumability in our study. This could have happened because leaf density is associated with the number of plant cells and the thickness of their cell walls (Roderick et al. 1999; Grootemaat et al. 2017), and denser tissues likely store a greater calorific content and can reach higher combustion temperatures (Kataki and Konwer 2001). Although several studies have observed a positive relationship between SLA and ignitability (Murray et al. 2013; Grootemaat et al. 2015), it appears that higher combustion temperatures have a stronger impact on consumability than ignition speed.
Another important aspect to consider when examining combinations of leaves mixtures is the formation of the litter layer. In the litter layer, fuel flammability is often influenced by bulk density (the mass of dry fuel per unit volume) or packing ratio (the proportion of fuel volume relative to air volume within the fuel layer; Grootemaat et al. 2017). At very high bulk densities or packing ratios, fire can become oxygen-limited. In these poorly ventilated litter beds, flammability can increase linearly with oxygen availability (Drysdale 1999). Conversely, in well-ventilated litter beds, other factors such as surface area available for combustion and leaf flammability become more critical (Drysdale 1999). As we mentioned earlier, leaf traits play a role in shaping the litter layer. In this way, the negative relationship between SLA and consumability may be explained by how SLA affects bulk density. Lower SLA values seem to increase bulk density (Burton et al. 2021), which then increases the fuel’s consumability. This likely occurs because oxygen was not a limiting factor in these litter beds. In this scenario, similarly to leaf density, higher bulk densities indicate higher caloric content per volume and higher temperatures reached during burning (Kataki and Konwer 2001). As a result, this enhances consumability.
As expected in H3, we found a positive relationship between FRic and consumability. FRic, which measures species functional diversity, reflects the complementarity of species niches in relation to resource use (Hooper et al. 2005; Mason et al. 2005). In the context of fire, higher FRic indicates complementarity in traits such as ignitability and combustibility. Traits like LFMCig and MCR, which influence ignition ease and combustion rate (Pausas et al. 2017), contribute to functional diversity, which promotes more complete biomass consumption. For instance, species that ignite quickly but burn slowly, such as Eryngium, and those that ignite more slowly but burn intensely, like Machaerina, complement each other when burned together, enhancing overall consumability. Species with fast ignition can cause the entire mixture to catch fire quickly, whereas species with intense burning can increase the combustibility of the mixture, leading to higher consumability. Therefore, greater FRic results in greater complementarity of species niches in relation to these traits, making it more likely that mixtures will contain species with different levels of ignitability and combustibility, ultimately increasing their consumability.
However, functional divergence, which measures the dissimilarity among species in trait space, did not show a significant relationship with consumability in our study. This suggests that although species within the community may differ in certain traits, the degree of dissimilarity among species within the trait space did not consistently contribute to variations in how biomass was consumed by fire. This result underscores that it is the complementarity rather than dissimilarity of traits among species that primarily influences consumability in this context.
Integrating species sensitivity to climate change with future fire severity
Campos de altitude are particularly vulnerable to climate change (Assis and Mattos 2016) and increasing numbers of anthropogenic fires, which tend to be more intense as they occur during the dry season (Aximoff and Rodrigues 2011). Therefore, understanding the environmental changes and plant functional traits that influence fire behavior is essential for the long-term conservation of these ecosystems. In a previous study, Matos et al. (2021) analyzed the response of campos de altitude species to drought, integrating the ecological strategies proposed by Grime (1977) (i.e. stress tolerance S, competitors C, and ruderals R) with the physiological strategies of Levitt (1972) (i.e. tolerance, escape, avoidance). They found that Leptostelma, one of the species with a major contribution to leaf mixtures’ consumability, follows a CR-escape/avoidance strategy, which may make it more vulnerable to future climate changes because it lacks mechanisms to tolerate drought. Consequently, as drought intensifies in mountainous areas, the loss of Leptostelma could lead to more intense and severe fires in the campos de altitude. The changes in fire severity due to a decline in Leptostelma abundance are likely to be non-linear and difficult to predict because of the non-additive effects its presence has on the community’s consumability. Other species with high contributions to reducing leaf consumability, such as Pleroma and Chusquea follow a more drought-resistant strategy (S-tolerance/avoidance). Although these three species showed a high contribution to consumability, Pleroma and Chusquea exhibited similar consumability values compared with C. modesta, a species with high consumability. Therefore, despite Leptostelma’s high sensitivity to drought, we recommend conserving this species as a management strategy to modulate fire severity in campos de altitude. This is because Leptostelma was the only species that both significantly contributed to the consumability of mixtures and showed lower consumability compared with high-consumability species like Cortaderia.
Contrary to our expectations (H1), the co-dominant species Cortaderia and Machaerina showed the lowest contribution to consumability. These species, classified as CS-escape/tolerance (Matos et al. 2021), have high consumability, low SLA, high LDMC and high MCR. They can benefit from increased fire frequency, as fire stimulates their flowering (Brade 1956), and their dense clumps may offer protection against high temperatures during fires (Safford 2001). As both drought and fire frequency intensifies, these species may become more abundant, increasing fire severity and reducing the presence of less frequent species, including Leptostelma.
Mechanisms of non-additive effects in ecosystems process
Non-additive effects have been widely studied in leaf litter decomposition experiments, where deviations from expected outcomes are attributed to interactions among species traits (Dias et al. 2013; Finerty et al. 2016). One possible explanation is the improved microenvironmental condition theory (Makkonen et al. 2013), which suggests that certain species, depending on their traits, create microclimatic conditions that change the decomposition of others. For example, plant litter traits that improve conditions for decomposers can promote the breakdown of co-occurring litter species (Makkonen et al. 2013). In fire ecology, non-additive effects may arise from species traits that alter the microenvironment within the litter layer. In our study, species with high SLA and low LDMC – traits associated with lower leaf density and possibly lower bulk density (Burton et al. 2021) – and low MCR decreased consumability of mixtures. This was likely because oxygen was not limiting, and the less dense litter reduced heat retention and calorific content per unit volume, creating unfavorable conditions for whole biomass consumption by fire. As a result, these interactions led to consumability values in mixtures lower than expected based on individual species values.
Our experimental set-up followed a trait-based approach, one focus being to assess how species’ traits influence ecological processes (Rosado et al. 2013). Controlled experiments are crucial for reliably comparing species while minimizing external variability (Pérez-Harguindeguy et al. 2013). In our study, heat exposure was controlled by burning leaf material in a muffle furnace, and small cut leaf samples were used to standardize species proportions, reflecting aspects of litter fuels. Although the role of leaf litter in campos de altitude fire dynamics remains unclear, its influence cannot be disregarded. However, this approach does not fully replicate natural fuel conditions, as the herbaceous layer, which often burns as whole individuals, is another key fuel component (Instituto Chico Mendes de Conservação da Biodiversidade (ICMBio) 2023). Leaf traits and flammability measurements have been criticized for poorly predicting field fire behavior, as they often neglect plant architecture, a critical factor influencing ecosystem flammability (Fernandes and Cruz 2012). Nonetheless, leaf traits like LDMC have been shown to exert stronger effects on shoot flammability than architectural traits (Alam et al. 2024). This underscores the relevance of trait-based approaches based on plant organs, where leaf traits can serve as robust proxies to predict fire behavior. By assessing non-additive effects, our study sheds light on species interactions and their role in fire severity, a critical but understudied aspect of campos de altitude.
Conclusion
Our findings highlight the importance of evaluating the interactions among burning species in mixtures and of identifying key species and traits that contribute most to the overall community flammability. Although functional diversity was shown to be important, average values of community traits had the most significant impact on mixtures consumability. Specifically, SLA was the most influential trait on consumability, followed by MCR and LDMC. We also found evidence of non-additive effects on the consumability of mixtures, where the species Leptostelma, Pleroma and Chusquea with low and medium consumability may play a key role in modulating fire severity. Leptostelma was the only species with a high contribution to the consumability of mixtures and significantly lower consumability values compared with species with high consumability. Given the increasing risk of extreme fires due to climate change, conserving Leptostelma could be a key strategy for mitigating fire severity, with it potentially serving as natural firebreaks around infrastructure. Although our lab-based findings indicate specific effects of species and traits on community consumability, we encourage their validation through further field studies. Future research should consider factors like fuel structure/arrangement, and environmental variables to better understand their implications for fire severity. Nonetheless, we believe these results can guide management strategies in campos de altitude and improve the effectiveness of conservation efforts in facing future environmental challenges.
Declaration of funding
This research was supported by LabEx CeMEB, an ANR "Investissements d'avenir" program (ANR-10-LABX-04-01), funded through the I-SITE Excellence Program of the University of Montpellier, under the Investissements France 2030. H.R. was funded by the Coordenação de Aperfeiçoamento de Pessoal de Nível Superior – Brazil (CAPES) – Finance Code 001 and by FAPERJ – Finance code E-26/200.273/2020. B.H.P.R. was supported by FAPERJ, Programa cientistas do nosso estado – Finance code E-26/200.446/2023. I.S.M. was funded by RUFFORD 18749-1 and CAPES 88882.182435/2018-01.
Acknowledgements
This paper is derived from H.R.’s PhD thesis at the Research Institute of Botanical Garden of Rio de Janeiro. We are thankful to all members of the Plant Ecology Lab at State University of Rio de Janeiro for their assistance in both field and laboratory work, to the Itatiaia National Park staff for all logistical support and to the University of Montpellier, CNRS, CIRAD, INRAE, IRD and Labex CeMEB, Montpellier, France, for the support and infrastructure provided. This project was developed under license SISBIO 67669-7.
Author contributions
H.R., B.H.P.R. and I.O. conceived the ideas and designed methodology. I.S.M. and H.R. collected and curated the data. H.R. analyzed the data and led the writing of the manuscript. All the authors contributed critically to the drafts and gave final approval for publication.
References
Alam MA, Wyse SV, Buckley HL, Perry GLW, Sullivan JJ, Mason NWH, Buxton R, Richardson SJ, Curran TJ (2020) Shoot flammability is decoupled from leaf flammability, but controlled by leaf functional traits. Journal of Ecology 108, 641-653.
| Crossref | Google Scholar |
Alam MA, Wyse SV, Buckley HL, Perry GLW, Cui X, Sullivan JJ, Schwilk DW, Curran TJ (2024) Fuel architecture influences interspecific variation in shoot flammability, but not as much as leaf traits. Journal of Ecology 113, 322-338.
| Crossref | Google Scholar |
Anderson HE (1970) Forest fuel ignitibility. Fire Technology 6, 312-319.
| Crossref | Google Scholar |
Assis MV, Mattos EAD (2016) Vulnerabilidade da vegetação de campos de altitude às mudanças climáticas. Oecologia Australis 20, 162-174.
| Crossref | Google Scholar |
Aximoff I, Rodrigues RDC (2011) Histórico dos incêndios florestais no Parque Nacional do Itatiaia. Ciência Florestal 21, 83-92.
| Crossref | Google Scholar |
Bond W, Keeley J (2005) Fire as a global ‘herbivore’: the ecology and evolution of flammable ecosystems. Trends in Ecology & Evolution 20, 387-394.
| Crossref | Google Scholar | PubMed |
Boving I, Celebrezze J, Salladay R, Ramirez A, Anderegg LDL, Moritz M (2023) Live fuel moisture and water potential exhibit differing relationships with leaf‐level flammability thresholds. Functional Ecology 37(11), 2770-2785.
| Crossref | Google Scholar |
Bowman DMJS, Balch JK, Artaxo P, Bond WJ, Carlson JM, Cochrane MA, D’Antonio CM, DeFries RS, Doyle JC, Harrison SP, Johnston FH, Keeley JE, Krawchuk MA, Kull CA, Marston JB, Moritz MA, Prentice IC, Roos CI, Scott AC, Swetnam TW, van der Werf GR, Pyne SJ (2009) Fire in the arth system. Science 324, 481-484.
| Crossref | Google Scholar | PubMed |
Brade AC (1956) A flora do Parque Nacional do Itatiaia. Boletim do Parque Nacional do Itatiaia 5, 1-112 [In Portuguese].
| Google Scholar |
Burton JE, Cawson JG, Filkov AI, Penman TD (2021) Leaf traits predict global patterns in the structure and flammability of forest litter beds. Journal of Ecology 109, 1344-1355.
| Crossref | Google Scholar |
Cardoso AW, Oliveras I, Abernethy KA, Jeffery KJ, Lehmann D, Edzang Ndong J, McGregor I, Belcher CM, Bond WJ, Malhi YS (2018) Grass species flammability, not biomass, drives changes in fire behavior at tropical forest-savanna transitions. Frontiers in Forests and Global Change 1, 6.
| Crossref | Google Scholar |
Della Rocca G, Danti R, Hernando C, Guijarro M, Madrigal J (2018) Flammability of two Mediterranean mixed forests: study of the non-additive effect of fuel mixtures in laboratory. Frontiers in Plant Science 9, 825.
| Crossref | Google Scholar | PubMed |
de Magalhães RMQ, Schwilk DW (2012) Leaf traits and litter flammability: evidence for non‐additive mixture effects in a temperate forest. Journal of Ecology 100, 1153-1163.
| Crossref | Google Scholar |
Dias ATC, Berg MP, De Bello F, van Oosten AR, Bílá K, Moretti M (2013) An experimental framework to identify community functional components driving ecosystem processes and services delivery. Journal of Ecology 101, 29-37.
| Crossref | Google Scholar |
Fernandes PM, Cruz MG (2012) Plant flammability experiments offer limited insight into vegetation–fire dynamics interactions. New Phytologist 194, 606-609.
| Crossref | Google Scholar | PubMed |
Finerty GE, De Bello F, Bílá K, Berg MP, Dias ATC, Pezzatti GB, Moretti M (2016) Exotic or not, leaf trait dissimilarity modulates the effect of dominant species on mixed litter decomposition. Journal of Ecology 104, 1400-1409.
| Crossref | Google Scholar |
Gill AM, Zylstra P (2005) Flammability of Australian forests. Australian Forestry 68, 87-93.
| Crossref | Google Scholar |
Grime JP (1977) Evidence for the existence of three primary strategies in plants and its relevance to ecological and evolutionary theory. The American Naturalist 111, 1169-1194.
| Crossref | Google Scholar |
Grootemaat S, Wright IJ, van Bodegom PM, Cornelissen JHC, Cornwell WK (2015) Burn or rot: leaf traits explain why flammability and decomposability are decoupled across species. Functional Ecology 29, 1486-1497.
| Crossref | Google Scholar |
Grootemaat S, Wright IJ, van Bodegom PM, Cornelissen JHC (2017) Scaling up flammability from individual leaves to fuel beds. Oikos 126, 1428-1438.
| Crossref | Google Scholar |
Hantson S, Andela N, Goulden ML, Randerson JT (2022) Human-ignited fires result in more extreme fire behavior and ecosystem impacts. Nature Communications 13, 2717.
| Crossref | Google Scholar | PubMed |
Hardesty J, Myers R, Fulks W (2005) Fire, ecosystems, and people: a preliminary assessment of fire as a global conservation issue. The George Wright Forum 22(4), 78-87.
| Google Scholar |
Hooper DU, Chapin FS, Ewel JJ, Hector A, Inchausti P, Lavorel S, Lawton JH, Lodge DM, Loreau M, Naeem S, Schmid B, Setälä H, Symstad AJ, Vandermeer J, Wardle DA (2005) Effects of biodiversity on ecosystem functioning: a consensus of current knowledge. Ecological Monographs 75, 3-35.
| Crossref | Google Scholar |
Instituto Chico Mendes de Conservação da Biodiversidade (ICMBio) (2023) ‘Integrated Fire Management Plan of Itatiaia National Park.’ (Ministry of the Environment) Available at https://www.gov.br/icmbio/pt-br/centrais-de-conteudo/publicacoes/planos-de-manejo-integrado-do-fogo/PMIFParnadoItatiaia_compressed.pdf [verified 25 September 2024]
Kataki R, Konwer D (2001) Fuelwood characteristics of some indigenous woody species of north-east India. Biomass and Bioenergy 20, 17-23.
| Crossref | Google Scholar |
Keeley JE (2009) Fire intensity, fire severity and burn severity: a brief review and suggested usage. International Journal of Wildland Fire 18, 116.
| Crossref | Google Scholar |
Krix DW, Phillips ML, Murray BR (2019) Relationships among leaf flammability attributes and identifying low-leaf-flammability species at the wildland–urban interface. International Journal of Wildland Fire 28(4), 295.
| Crossref | Google Scholar |
Laliberté E, Legendre P (2010) A distance‐based framework for measuring functional diversity from multiple traits. Ecology 91, 299-305.
| Crossref | Google Scholar | PubMed |
Lyra A, Tavares P, Chou SC, Sueiro G, Dereczynski C, Sondermann M, Silva A, Marengo J, Giarolla A (2018) Climate change projections over three metropolitan regions in southeast Brazil using the non-hydrostatic ETA regional climate model at 5-km resolution. Theoretical and Applied Climatology 132, 663-682.
| Crossref | Google Scholar |
Makkonen M, Berg MP, van Logtestijn RS, van Hal JR, Aerts R (2013) Do physical plant litter traits explain non‐additivity in litter mixtures? A test of the improved microenvironmental conditions theory. Oikos 122, 987-997.
| Crossref | Google Scholar |
Martin R, Gordon R, Gutierrez, MA, Lee D, Molina-Terrén DM, Schroeder RA, Sapsis DB, Stephens SL (1993) Assessing the flammability of domestic and wildland vegetation. In ‘Proceedings of the 12th Conference on Fire and Forest Meteorology’. pp. 26-28. (Society of American Foresters) 10.13140/RG.2.1.3999.3680
Mason NWH, Mouillot D, Lee WG, Wilson JB (2005) Functional richness, functional evenness and functional divergence: the primary components of functional diversity. Oikos 111, 112-118.
| Crossref | Google Scholar |
Matos IS, Eller CB, Oliveras I, Mantuano D, Rosado BHP (2021) Three eco‐physiological strategies of response to drought maintain the form and function of a tropical montane grassland. Journal of Ecology 109, 327-341.
| Crossref | Google Scholar |
Murray BR, Hardstaff LK, Phillips ML (2013) Differences in leaf flammability, leaf traits and flammability-trait relationships between native and exotic plant species of dry sclerophyll forest. PLoS One 8, e79205.
| Crossref | Google Scholar | PubMed |
Oliveras I, Malhi Y (2016) Many shades of green: the dynamic tropical forest–savannah transition zones. Philosophical Transactions of the Royal Society B: Biological Sciences 371, 20150308.
| Crossref | Google Scholar | PubMed |
Oliveras I, Meirelles ST, Hirakuri VL, Freitas CR, Miranda HS, Pivello VR (2013) Effects of fire regimes on herbaceous biomass and nutrient dynamics in the Brazilian savanna. International Journal of Wildland Fire 22, 368.
| Crossref | Google Scholar |
Pausas JG, Keeley JE, Schwilk DW (2017) Flammability as an ecological and evolutionary driver. Journal of Ecology 105, 289-297.
| Crossref | Google Scholar |
Parsons AL, Balch JK, de Andrade RB, Brando PM (2015) The role of leaf traits in determining litter flammability of south-eastern Amazon tree species. International Journal of Wildland Fire 24(8), 1143.
| Crossref | Google Scholar |
Pérez-Harguindeguy N, Díaz S, Garnier E, Lavorel S, Poorter H, Jaureguiberry P, Bret-Harte MS, Cornwell WK, Craine JM, Gurvich DE , Urcelay C, Veneklaas EJ, Reich PB, Poorter L, Wright IJ, Ray P, Enrico L, Pausas JG, De Vos AC, Buchmann N, Funes G, Quétier F, Hodgson JG, Thompson K, Morgan HD, Ter Steege H, Sack L, Blonder B, Poschlod P, Vaieretti MV, Conti G, Staver AC, Aquino S, Cornelissen JHC (2013) New handbook for standardised measurement of plant functional traits worldwide. Australian Journal of Botany 61, 167.
| Crossref | Google Scholar |
Petchey OL, Hector A, Gaston KJ (2004) How do different measures of functional diversity perform? Ecology 85, 847-857.
| Crossref | Google Scholar |
R Core Team (2023) ‘R: a language and environment for statistical computing’. (R Foundation for Statistical Computing: Vienna, Austria) Available at https://www.R-project.org/ [accessed 10 September 2024]
Rego CF, Morgan P, Fernandes P, Hoffman C (2021) Fuel and Fire Behavior Description. In ‘Fire Science: From Chemistry to Landscape Management’. Springer Textbooks in Earth Sciences, Geography and Environment. (Eds CF Rego, P Morgan, P Fernandes, C Hoffman) pp. 101–114. (Springer International Publishing: Cham, Switzerland) 10.1007/978-3-030-69815-7_6
Ritz C, Baty F, Streibig JC, Gerhard D (2015) Dose–response analysis using R. PLoS One 10, e0146021.
| Crossref | Google Scholar | PubMed |
Roderick ML, Berry SL, Noble IR, Farquhar GD (1999) A theoretical approach to linking the composition and morphology with the function of leaves. Functional Ecology 13, 683-695.
| Crossref | Google Scholar |
Rosado BH, Dias AT, de , Mattos EA (2013) Going back to basics: importance of ecophysiology when choosing functional traits for studying communities and ecosystems. Natureza & Conservação 11, 15-22.
| Crossref | Google Scholar |
Safford HD (1999) Brazilian Páramos I. An introduction to the physical environment and vegetation of the campos de altitude. Journal of Biogeography 26, 693-712.
| Crossref | Google Scholar |
Safford HD (2001) Brazilian Päramos. III. Patterns and rates of postfire regeneration in the campos de altitude. Biotropica 33, 282-302.
| Crossref | Google Scholar |
Segadas-Vianna F, Dau L (1965) Ecology of the Itatiaia range, southeastern Brazil. II. Climates and Altitudinal Climatic Zonation. Arquivos do Museu Nacional 53, 31–53. https://biostor.org/reference/248948
Simpson KJ, Ripley BS, Christin P, Belcher CM, Lehmann CER, Thomas GH, Osborne CP (2016) Determinants of flammability in savanna grass species. Journal of Ecology 104, 138-148.
| Crossref | Google Scholar |
Spehn EM, Hector A, Joshi J, Scherer-Lorenzen M, Schmid B, Bazeley-White E, Beierkuhnlein C, Caldeira MC, Diemer M, Dimitrakopoulos PG, Finn JA, Freitas H, Giller PS, Good J, Harris R, Högberg P, Huss-Danell K, Jumpponen A, Koricheva J, Leadley PW, Loreau M, Minns A, Mulder CPH, O’Donovan G, Otway SJ, Palmborg C, Pereira JS, Pfisterer AB, Prinz A, Read DJ, Schulze E-D, Siamantziouras A-SD, Terry AC, Troumbis AY, Woodward FI, Yachi S, Lawton JH (2005) Ecosystem effects of biodiversity manipulations in European grasslands. Ecological Monographs 75, 37-63.
| Crossref | Google Scholar |
Stevens N, Bond W, Feurdean A, Lehmann CER (2022) Grassy ecosystems in the Anthropocene. Annual Review of Environment and Resources 47, 261-289.
| Crossref | Google Scholar |
Tilman D, Knops J, Wedin D, Reich P, Ritchie M, Siemann E (1997) The influence of functional diversity and composition on ecosystem processes. Science 277, 1300-1302.
| Crossref | Google Scholar |
van Altena C, van Logtestijn RSP, Cornwell WK, Cornelissen JHC (2012) Species composition and fire: non-additive mixture effects on ground fuel flammability. Frontiers in Plant Science 3, 63.
| Crossref | Google Scholar | PubMed |
van der Werf GR, Randerson JT, Giglio L, van Leeuwen TT, Chen Y, Rogers BM, Mu M, van Marle MJE, Morton DC, Collatz GJ, Yokelson RJ, Kasibhatla PS (2017) Global fire emissions estimates during 1997–2016. Earth System Science Data 9, 697-720.
| Crossref | Google Scholar |