Using molecular and morphometric data as operational criteria for the analysis of a threatened rainforest species complex shows interspecific variation, with implications for cryptic-species delimitation and conservation
Aaron J. Brunton
A
B
C
D
E
F
Abstract
Resolution of systematic associations and species boundaries is vital for developing conservation priorities for threatened taxa. A complex of Fontainea (Euphorbiaceae) populations, endemic to rainforest communities in central-eastern Australia, comprises several taxonomically challenging species. Fontainea oraria is Critically Endangered, with only one natural population of 10 mature individuals, with the closely related species F. australis being listed as Vulnerable. A recently discovered Fontainea population (currently nominated as F. sp. Coffs Harbour) with taxonomic similarities to F. oraria has been provisionally listed as Critically Endangered, with fewer than 40 individuals. This study employed an integrative approach, combining genetic data and morphometrics to determine species boundaries for three threatened Fontainea population groups as a model system for an integrative approach to delimiting cryptic species. Although our results suggest the potential subdivision of the population groups into three taxa, caution is warranted because this hypothesis remains inconclusive. Taxonomic challenges demand a careful approach, acknowledging the possibility of alternative interpretations. Contrary to supporting distinct species, our morphological and genetic data may also be interpreted as indicative of a single species with geographic variation, a phenomenon observed in genetically linked populations experiencing isolation by distance. Overall, we highlight the need for further research to establish species limits to guide conservation actions.
Keywords: conservation genetics, digital morphometrics, genome-wide markers, geographic variation, non-destructive sampling, rare species, species delineation, taxonomic resolution.
Introduction
Species delimitation has important consequences for biodiversity conservation (De Queiroz 2007; Carstens et al. 2013), and the extensive number of different species concepts (>25) continues to generate confusion and debate (Zachos 2018). As such, there remains no universally accepted definition of what is a ‘species’ (Frankham et al. 2012). Clearly, there are no species concepts without inherent flaws in relation to biological reality and, therefore, selecting an appropriate concept can have major conservation consequences (Frankham et al. 2012; Bradbury et al. 2023). Whatever the favoured approach, using a suitable definition and application of a species concept for delineating species is critical for evaluating conservation priorities for threatened taxa. Therefore, employing a unifying species concept to delineate interspecific boundaries is crucial for understanding these fundamental units of biology (De Queiroz 2007). Central to this concept is an understanding of the range of evidence, often termed ‘operational criteria’, that are suitable for identifying species-level diversity enabling evaluation of these evolutionary lineages to inform conservation initiatives (Sites and Marshall 2004; De Queiroz 2007). Although significant progress has been made for species delimitation on the basis of molecular criteria (Fujita et al. 2012), defining boundaries among closely related plant taxa remains challenging. Integrating a combination of approaches, such as, for example, DNA-based methods and morphometrics, has become more widely utilised to resolve boundaries of especially difficult-to-define congeners (Zheng et al. 2017). By combining multiple lines of evidence (operational criteria), a broader array of information can be assessed, and where largely concordant, results can be used to better delineate boundaries between species and, in turn, identify distinct groups for conservation priority.
Within the Euphorbiaceae family, subfamily Crotonoideae, subtribe Baloghinae G.L.Webster, includes several small, cryptic lineages, such as Alphandia Baill., Baloghia Endl., and Fontainea Heckel that are found in isolated populations across several Western Pacific islands (New Caledonia, Vanuatu, Norfolk Island), Papua New Guinea and eastern Australia (van Welzen et al. 2021). Fontainea is a small plant genus of dioecious shrubs and trees confined to rainforest communities, with nine recognised, dioecious species that are patchily distributed in rainforest habitats of eastern Australia, Papua New Guinea, New Caledonia, and Vanuatu (Forster 1997). The recent discovery of a new Fontainea population in Coffs Harbour, New South Wales (NSW), Australia, has sparked interest in its taxonomic and genetic associations, together with its position within the broader genus. This novel population is geographically significant, representing a southern range extension for the genus, thus raising questions as to putative relationships to geographically close relatives, which were previously known to be at the southern limit of Fontainea distribution.
Initial taxonomic assessment has indicated the Coffs Harbour population of Fontainea as a potential new species, which has an accepted phrase name Fontainea sp. Coffs Harbour A.S. Benwell 341, NSW1102027 (National Herbarium of New South Wales 2021). Although yet to be formally described, this new Fontainea entity shares taxonomic affinities with F. oraria, but is distinguished by features of its fruit, indicating that it could be a distinct species (PlantNET, Royal Botanic Gardens and Domain Trust, Sydney, NSW, Australia, see https://plantnet.rbgsyd.nsw.gov.au, accessed 23 March 2023). Comprising fewer than 40 individuals (Brunton et al. 2024), and only three mature fruiting plants, this new entity is currently threatened by the construction footprint of a major road bypass, contributing to a provisional listing as Critically Endangered (PlantNET, see https://plantnet.rbgsyd.nsw.gov.au). Its closest relatives from a geographic perspective, the sister-subclade of F. australis Jessup & Guymer (southern Fontainea) and F. oraria Jessup & Guymer (coastal Fontainea), are located ~220 km north of Coffs Harbour, and are also both of major conservation concern. The Critically Endangered F. oraria comprises only 10 mature plants at Lennox Head, New South Wales (NSW), which has triggered in situ and ex situ conservation strategies to boost the number of individuals and improve genetic resources (Brown et al. 2017). Wild plants of F. oraria face an elevated risk of extinction because of low genetic diversity, habitat degradation (Rossetto et al. 2000), restricted formal protection, and projections of future habitat decline (Brunton et al. 2023). Although F. australis has a broader distribution across the Queensland (Qld)–New South Wales (NSW) border ranges and the Wollumbin erosion caldera, there are a limited number of small, isolated populations, including sites with only single specimens (Brunton et al. 2022). As such, it is listed as Vulnerable (Environment Protection and Biodiversity Conservation Act 1999), and because of a range of threatening processes, including ongoing habitat degradation, this species was identified as a conservation priority in the region (Department of Environment, Climate Change and Water NSW 2010).
Genetic studies have highlighted that the taxonomic delimitation of F. australis and F. oraria requires further attention (Rossetto et al. 2000; Brunton et al. 2022). Molecular evidence has indicated that some geographically discrete populations of F. australis are also genetically distinct, whereas some F. australis populations share genotypes with F. oraria (Brunton et al. 2022). However, species delimitation based on genetic makers alone has served to highlight the challenges in applying only molecular evidence for resolution of this southern lineage of Fontainea. Recent genotype–environment analyses of southern Fontainea and coastal Fontainea also indicated that there are several geographically and genetically distinct clusters within this group. Yet, species delimitation continues to be a challenge, even when using a range-wide sampling approach (Brunton et al. 2024). Considering the potential for high levels of intraspecific morphological variation to conceal species boundaries (Jones et al. 2013), it is clear that deploying a unifying species concept to examine the relationships among the established groups, as well as any potential novel Fontainea taxa, is warranted and has important conservation implications.
Since the publication of earlier taxonomic revisions of Fontainea (Forster 1997; Forster and Van Welzen 1999), a more recent, broader resource of herbarium specimens has been established for F. australis and other congeneric species in the course of collecting material for contemporary genetic and ecological research (Brunton et al. 2024). This expanded collection of specimens has yielded morphometric data, which combined with a renewed molecular analysis, have enabled examination of the cryptic species boundaries of this threatened species complex that, in turn, will benefit conservation management efforts, and their long-term survival.
Therefore, in the present study, we aimed to determine whether using operational criteria that include phylogeny, genetic diversity, and morphological variation in an analysis could identify species boundaries and the evolutionary lineages within F. australis and F. oraria, and their association with F. sp. Coffs Harbour. With this new addition, there is an opportunity to update the genetic associations of these three taxa and apply an integrated approach by using morphometrics for an improved definition of the species boundaries to enhance conservation activities for this group. In this study, we used a reduced-representation genome-sequencing platform (diversity arrays technology sequencing, DArTseq) to generate single-nucleotide polymorphism (SNP) markers for molecular analysis and conducted morphological character analysis of leaf traits (because of the absence of comprehensive reproductive material) from representative populations from a Fontainea group with two previously recognised species and the newly found entity. Foremost, we aimed to (1) clarify how many species there are within this complex of F. australis, F. oraria and F. sp. Coffs Harbour, and (2) highlight the evolutionary history of this regionally significant rainforest lineage.
Materials and methods
Study taxa
Current taxonomic classifications of Fontainea are based on traditional morphologically focussed techniques. Several taxa display unique identifying features that allow for simple delineation between the species following this approach (Jessup and Guymer 1985; Forster 1997; Forster and Van Welzen 1999). However, morphological plasticity within Fontainea from the southern clade (Rossetto et al. 2000) suggests that a clearer resolution of the genus could be achieved by combining analysis of molecular markers and morphological characters to evaluate phenotypic variation among putative taxa. Recent evidence from a set of reduced-representation markers (DArTseq) showed F. oraria to be closely aligned with several F. australis populations, and that F. oraria potentially represents an ecotype that forms part of a broader species continuum within F. australis (Brunton et al. 2024). To provide a more definitive characterisation of the species boundaries, a rigorous analysis with phenotype data was recommended for future studies of this challenging complex.
Sampling
Our combined analysis of silica-dried leaf material to generate reduced-representation genomic data for molecular analysis, along with a separate set of oven-dried material from field-collected specimens for the morphometric component. Leaf material from populations of F. australis, F. oraria and the Coffs Harbour Fontainea was collected throughout their geographical distributions in central-eastern Australia (Fig. 1).
Sampling locations of an Australian rainforest complex, including Fontainea australis (see middle inset map for population detail), F. oraria and F. sp. Coffs Harbour.
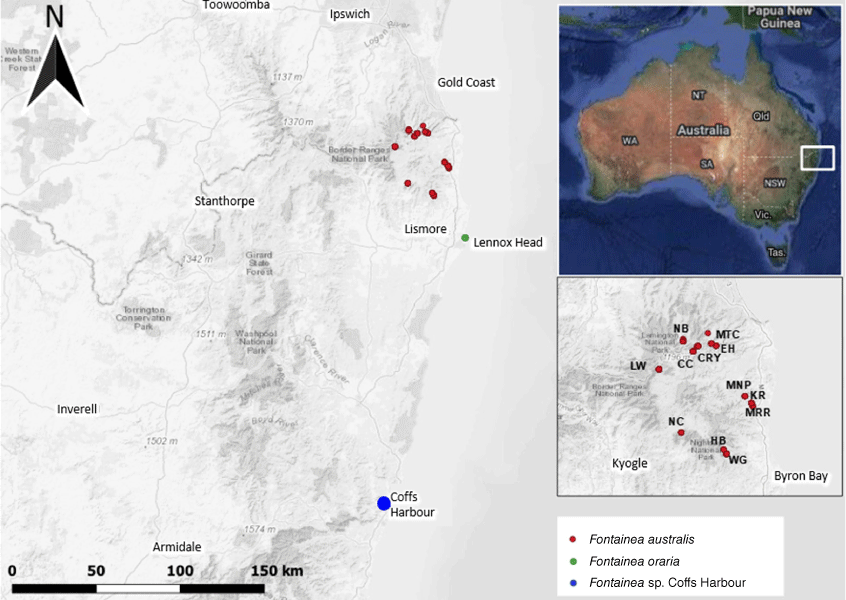
In total, 186 genetic accessions and 152 leaf accessions from 14 populations of the 3 Fontainea taxa were collected for genetic and morphological analyses (Table 1). In addition, representatives from two F. rostrata populations from the Gympie region in Queensland (Qld; Goomboorian and Greens Creek) were used as an outgroup to provide a measure of support for species delineation for genetic analyses. Fontainea rostrata was selected because it was previously recovered as a distinct species in a sister subclade to F. australis and F. oraria. (Brunton et al. 2022). A voucher representative for each population was lodged with the Queensland Herbarium (BRI), or where samples were supplied by an external source (F. australis – CC, CRY), we refer to the NSW Herbarium (NSW) voucher details (Table 1).
Taxon | Population code – location | Genetic | Leaf | Voucher representative | |
---|---|---|---|---|---|
F. australis | NB – Queensland | 14 | 17 | BRI AQ1038753 | |
F. australis | MTC – Queensland | 7 | 7 | BRI AQ1038756 | |
F. australis | EH – Queensland A | 10 | 10 | BRI AQ0670081 | |
F. australis | CC – New South Wales A | 12 | 13 | 135R (NSW) | |
F. australis | CRY – New South Wales A | 14 | 13 | SJJSI0389966 | |
F. australis | MNP – New South Wales | 7 | 4 | BRI AQ1038759 | |
F. australis | KR – New South Wales A | 13 | 13 | BRI AQ1038757 | |
F. australis | MRR – New South Wales | 7 | 7 | BRI AQ1038758 | |
F. australis | LW – New South Wales | 25 | 25 | BRI AQ1038765 | |
F. australis | NC – New South Wales | 7 | 7 | BRI AQ1038766 | |
F. australis | HB – New South Wales A | 3 | 3 | BRI AQ1038761 | |
F. australis | WG – New South Wales | 20 | 14 | BRI AQ1038763 | |
F. oraria | FO – Lennox Head, New South Wales A | 10 | 7 | BRI AQ1038767 | |
Coffs Harbour Fontainea | FCH – Coffs Harbour, New South Wales | 37 | 12 | BRI AQ1038768 | |
F. rostrata | FR – Goomboorian, Queensland | 3 | 10 | BRI AQ0450396 | |
F. rostrata | FR – Gympie, Queensland | 7 | 10 | BRI AQ0561492 |
Voucher representatives from all the taxa are deposited in BRI (Queensland Herbarium) or NSW (New South Wales Herbarium).
SNP genotyping and quality filtering
Genotype data were generated from silica-dried leaf samples (10–15 mg per individual) sent to Diversity Arrays Technology Pty Ltd (DArT, Canberra, ACT, Australia) who performed all DNA extractions and raw sequencing by using proprietary analytical pipelines, specifically employing DArTseq analysis. DArTseq utilises a combination of complexity reduction through restriction enzymes, implicit fragmentation size selection, and next-generation sequencing (NGS) (Kilian et al. 2012), similar to double-digest restriction-associated DNA sequencing (ddRAD; Peterson et al. 2012). As a result, DArTseq produces a collection of co-dominant SNPs accompanied by relevant metadata, providing insights into the quality of each SNP. The metadata include a reproducibility score for each locus, indicating the consistency of genotype calls across replicates.
DArTseq has been successfully applied to define a range of taxonomically challenging plant groups (Sansaloni et al. 2011; Steane et al. 2011; O’Donnell et al. 2023), including Fontainea (Brunton et al. 2022). Also, notably, the chromosome count and ploidy status of Fontainea are currently unknown and have not been investigated in the study species.
For this study, we used a dataset similar to that of Brunton et al. (2024); however, this new SNP data included samples of the novel Fontainea entity from Coffs Harbour. The quality of the SNP dataset was assessed using filtering scripts from the custom R package ‘RRtools’ from the Royal Botanical Garden, Sydney (Rossetto et al. 2019). To ensure the reliability of the analyses, only high-quality markers meeting specific criteria were retained. SNPs with a reproducibility of ≥96% and less than 20% missing data were included. In cases where a marker had multiple SNPs, one SNP was randomly chosen to avoid linkage bias. The proportion of missing data for the samples was 3.74% after quality checking. After these steps, the dataset was reduced to 18,314 SNPs that were used for subsequent genetic analyses.
Genomic-data analysis
Genetic trees constructed for F. australis, F. oraria and the Coffs Harbour Fontainea were rooted using Fontainea rostrata because this species is related to, but distinct from, the three focal groups within this southern complex (Brunton et al. 2022). Although this phylogeny-reconstruction approach considers the numerous independently inherited SNPs as sharing a single gene tree, which may not fully account for their independent evolution and inheritance, it still holds potential to offer insights into the phylogenetic relationships among individuals within the Fontainea complex, provided the interpretation is approached with caution.
To construct the genetic trees, a NEXUS file was generated and exported from RStudio (ver. 4.3.3, Posit Software, PBC, Boston, MA, USA, see https://posit.co/products/open‐source/rstudio/) by using a down-sample approach (SVD quartets) to randomly sample the filtered SNP dataset so that all classes have the same frequency as the minority class (Chifman and Kubatko 2014). Phylogenetic relationships among the three species were then reconstructed using FASTA alignments generated using Decipher (Wright 2016). To identify the best-fit model to implement for the phylogenetic analysis, we used the modelTest function from the R package Phangorn (ver. 2.11.1, see https://cran.r-project.org/package=phangorn; Schliep 2011) to highlight the substitution model with the greatest statistical support (GTR + G) on the basis of the lowest values of Akaike information criterion (AIC), AIC adjusted for small sample sizes (AICc) and Bayesian information criterion (BIC). Maximum-likelihood (ML) topologies were then constructed using Phangorn (ver. 2.11.1; Schliep 2011) and Phytools (ver. 2.1-1, see https://cran.r-project.org/package=phytools/; Revell 2012). To infer confidence values on the ML trees, we applied 100 replicates for the DArTseq dataset, then plotted bootstrap support values on tree edges for the GTR + G. Last, an optimised, GTR + G tree was run to allow for stochastic rearrangements. Optimised trees were then exported in Newick format for additional editing and visualised using FigTree (ver. 1.4.4, A. Rambaut, see http://tree.bio.ed.ac.uk/software/figtree/).
SplitsTree (ver. 4.41.6, see https://github.com/husonlab/splitstree4; Huson 1998) was used to conduct a network analysis with a NeighbourNet tree by using the same NEXUS file that was used for the phylogenetic analysis. Default settings on uncorrected characters with ambiguous states were applied to show a visual summary of genetic relationships and to identify areas that could reflect reticulation, incomplete lineage sorting or other processes that are represented through dense networks or ‘webs’ among branches.
To explore patterns of genetic structure among Fontainea taxa, we conducted a principal-component analysis (PCA) by using adegenet (ver. 2.1.10, see https://cran.r-project.org/package=adegenet; Jombart 2008) and generated a pairwise population genetic differentiation (FST) matrix with the diveRsity package (ver. 1.9.90, see https://cran.r-project.org/package=diveRsity; Keenan et al. 2013). To determine the correlation between genomic distribution and geographic distance (isolation by distance, IBD), we performed a Mantel test in the Vegan package (ver. 2.6-4, J. Oksanen et al., see https://cran.r-project.org/package=vegan/) by using pairwise Euclidian-distance matrices.
Finally, we assessed genomic diversity within the Fontainea complex by using the Hierfstat package (ver. 0.5-11, see https://cran.r-project.org/package=hierfstat; Goudet 2005) to estimate allelic richness (AR) with a rarefaction method, number of alleles per locus (size), observed heterozygosity (HO), expected heterozygosity (HE), inbreeding coefficient (FIS) and number of private alleles (npa).
Leaf morphometric characters and multivariate analysis
To examine the congruence of morphological traits with genetic divergence, we evaluated a set of seven leaf characters. Leaf-sampling protocols are not always clear in the literature; however, we followed similar published methods for morphometric (Silva et al. 2012) and functional analyses (Pérez-Harguindeguy et al. 2013), whereby medium-aged to young, fully expanded leaves were collected from the most sunlit areas of the canopy of each individual plant (>1.5 m in height). Morphological characters analysed include those reported in taxonomic treatments (Jessup and Guymer 1985; Forster 1997), as well as characters that represent key ecological strategies among plants and that appeared variable on the basis of our field observations (Fig. 2).
Leaf morphological diversity of the Fontainea complex from central eastern Australia. Each panel (a–h) displays a group of three oven-dried, medium-aged to young, fully expanded leaves collected from the canopy of a representative individual from various populations across the following three Fontainea taxa: (a) F. australis from private property (population – EHR) adjoining Springbrook National Park, Qld, (b) F. australis from private property in Mooball, NSW (population – KR), (c) F. australis from Limpinwood Nature Reserve (population – LW), NSW, (d) F. australis from road reserve in Mooball, NSW (population – MRR), (e) F. oraria from private property in Lennox Head, NSW, (f) F. oraria from private property in Lennox Head, NSW, (g) F. sp. Coffs Harbour from Korora, NSW, (h) F. sp. Coffs Harbour from wesern Korora, NSW. Scale bar: 20 mm.
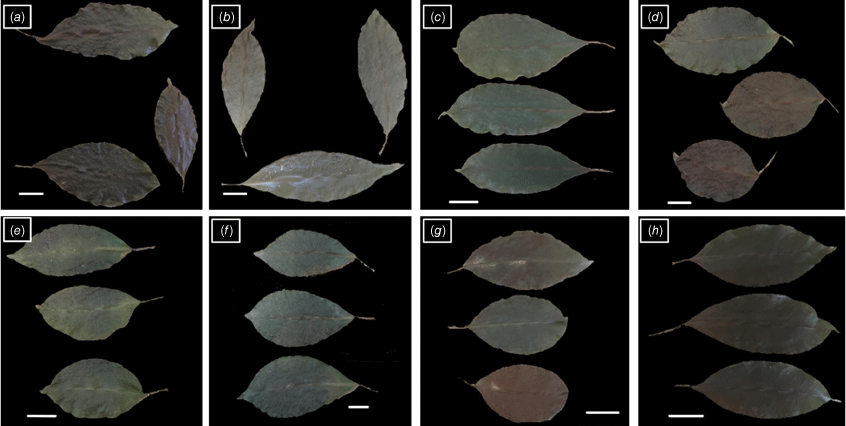
All characters were measured on the abaxial surface, with the average over three leaves collected for each specimen after being pressed flat while oven-dried in paper bags at 70°C for 72 h. This average-estimate approach follows the methods of a number of similar leaf morphometric studies (Rumpunen and Bartish 2002; Viscosi et al. 2009; Viscosi and Cardini 2011). Leaf length (LL) was measured at the midrib from the leaf base to apex, and leaf width (LW) at the widest point. Petiole length (PL) was measured as the distance from the apex to the base where the leaf was removed from the plant. For a quantitative leaf-shape surrogate, we used leaf ratio (LRTO), which was calculated as LL:LW. Averages of these measurements were calculated using ImageJ (Schneider et al. 2012), which was also used to calculate leaf area (LA).
Dry leaf mass (DLM) was measured from the oven-dried weight (g); this measure has been closely linked to absolute water content and leaf form and structure, which contribute to the carbon-integration cycle for leaf expansion and leaf-mass accumulation (Chaves et al. 2002; Ullah et al. 2013). Leaf water content state is strongly associated with leaf growth, turgor, transpiration, photosynthesis and stomatal conductance (Kramer and Boyer 1995). Typically, larger leaves are related to wet, hot and intense-light environments, whereas plants with small leaves are mostly found at high elevations or latitudes or in arid, hot systems (Wright et al. 2017). Specific leaf area (SLA; leaf area:dry leaf mass) was included as a key functional trait that represents the available area for capture of solar irradiance (Milla and Reich 2007) and has also been linked to important biological traits, including leaf nitrogen concentration, plant growth rate and lifespan (Lambers and Poorter 1992; Wright et al. 2004). In addition, interspecific differences of SLA have been strongly associated with resource partitioning among species, with low-SLA species related to nutrient-poor soils and a slow-growth strategy (Poorter and De Jong 1999; Baraloto et al. 2006).
Although floral and fruit features are an important diagnostic feature for taxonomic treatments of Fontainea (Jessup and Guymer 1985; Forster 1997), the asynchronous and irregular phenology, both within and among species, limited collection to only a few populations and individuals. We therefore could not include such data in the morphometric analysis.
As such, we applied a multivariate approach to assess leaf morphological differentiation among the three Fontainea taxa using the R package MorphoTools2 (ver. 1.0.1.1, see https://cran.r-project.org/package=MorphoTools2; Šlenker et al. 2022). Morphometric data were evaluated, first, by using a PCA based on the seven leaf traits, because this identifies potential group structure without assigning a priori groups for the samples (Šlenker et al. 2022). Furthermore, a canonical discriminant analysis (CDA) and classificatory discriminant analysis were performed at multiple levels (population and taxon) by using a linear discriminant analysis (LDA), with a stepwise selection to assess the marginal effect of certain characters with a leave-one-out cross-validation. We also performed the non-parametric, k-nearest neighbours’ (KNN) classification analysis to assess classifications at the individual level.
Last, to determine whether discrete leaf characters could discriminate among taxa, we generated conditional-inference trees with the R package partykit (ver. 1.2-20, see https://cran.r-project.org/package=partykit; Hothorn and Zeileis 2015) by using a classification tree analysis (CART). As an additional comparison to which leaf traits could be used to classify the three species, we also fit ANOVA models for the three taxa with the base R function, aov. Individual differences among taxa were determined by running Tukey HSD post hoc tests on the ANOVA model fit, with multiple comparisons of means at a 95% family-wise confidence level.
Results
Phylogenetic associations, genetic structure and diversity
The concatenation of the SNPs detected using DArTseq resulted in an alignment length of 18,457 nucleotides by using the quality-filtered data that also included F. rostrata. Analysis of the best-fit substitution model for phylogenetic reconstruction by using the DArTseq SNPs identified the GTR + G model as the best-fit model (Supplementary Table S1), which produced an ML tree with strong support for the delineation of the major clades. The model-based ML tree recovered a topology to support F. australis populations as divergent from F. oraria and the Coffs Harbour Fontainea entity (Fig. 3a). For F. australis, the ML tree was congruent with previous phylogenetic associations (Brunton et al. 2024); however, our revised analysis showed that three geographically proximate populations of MNP, KR and MRR as well as WG and HB were strongly supported as a well-differentiated genetic subgroup that is distinct from the remaining nine F. australis populations in a branch that was sister to F. oraria (Fig. 3a). There was also strong support (bootstrap value = 100), indicating that the Coffs Harbour entity is a distinct genetic cluster. These genetic associations were also reflected in the consensus network tree (Fig. 3b), which supported the novel Fontainea from Coffs Harbour as a genetically distinct group.
(a) Phylogenetic tree and (b) a consensus network of a Fontainea complex from central-eastern Australia constructed from individually aligned and concatenated DArTseq SNP sequence markers (18,457) by using a maximum-likelihood analysis. Fontainea rostrata was used as an outgroup representative to root the tree. The values on the phylogenetic tree at the branch nodes represent bootstrap support on the basis of the best-fit GTR + G model. Fontainea lineages recovered are represented outside the tip labels of the tree and tips and branches are coloured by species.
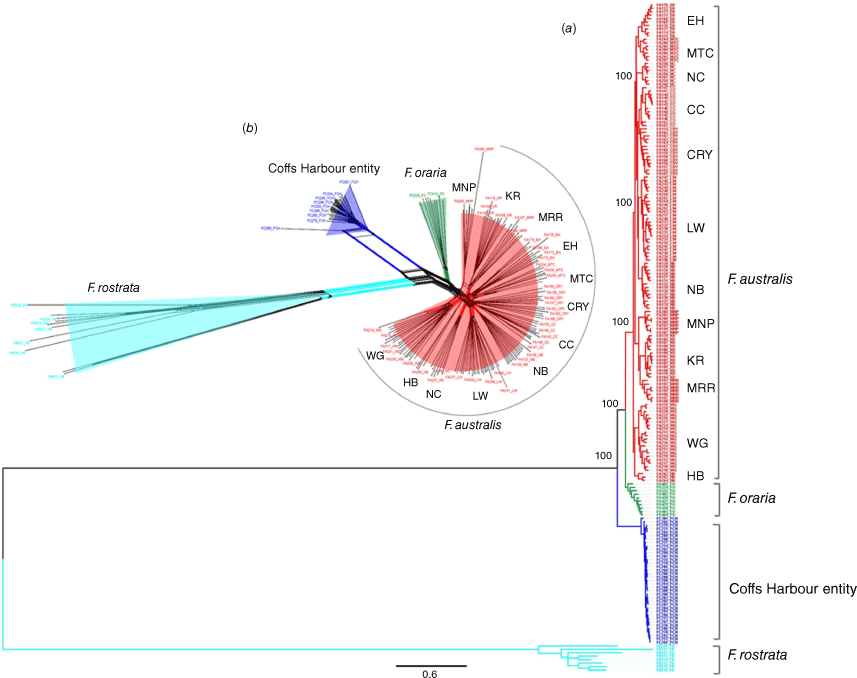
Network analysis concentrated on the three focal Fontainea groups recovered relationships similar to the phylogenetic topology and broader network with F. rostrata, with three main clusters (Fig. 4a), namely, one with Coffs Harbour Fontainea, a second with F. oraria, and a third, dense clade of all the F. australis samples comprising a range of subclusters that mostly represent the geographically clustered populations. PCA ordination (Supplementary Fig. S1) demonstrated similar patterns of genetic clustering that also identified Coffs Harbour Fontainea as a distinct group and F. oraria as closely aligned with some F. australis populations (MRR, MNP, KR, WG). IBD analysis showed that genetic divergence was strongly associated with geographic distance (Fig. 4b). This pattern was also reflected in the pairwise population estimates of genetic differentiation (FST) for the three Fontainea groups (Table S2). FST values among F. australis ranged from a level of minimal differentiation of FST = 0.232 (CRY) to a maximum of FST = 0.352 (MNP), with an overall average FST value of 0.292 (Table S2). Fontainea oraria had an average pairwise population FST value of 0.376 compared with the F. australis populations. Coffs Harbour Fontainea had an average pairwise population FST value of 0.677 compared with F. australis, and 0.685 with F. oraria (Table S2).
(a) Relationship between genetic and geographic distribution (isolation by distance, IBD) for three Fontainea taxa, F. australis, F. oraria and Coffs Harbour Fontainea (see inset legend), by using a Mantel test and, (b) NeighbourNet analysis of the three Fontainea taxa (see inset legend) from the rainforests of central-eastern Australia, generated using SplitsTree.
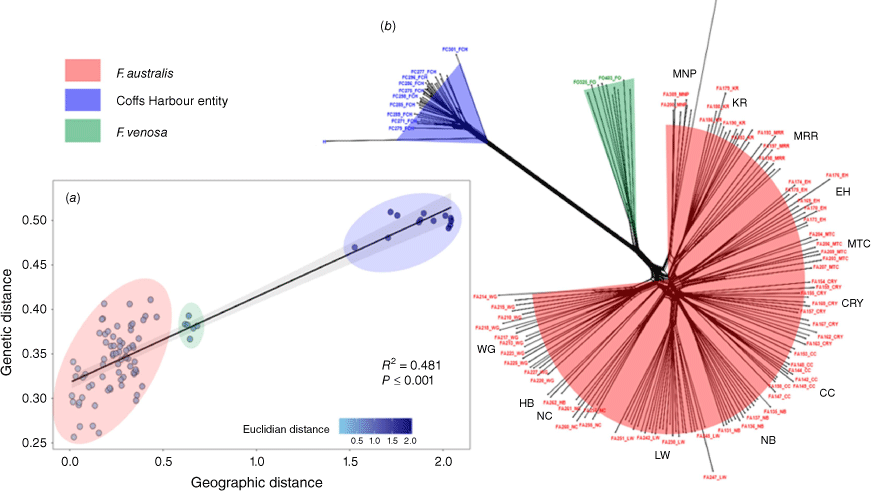
Genomic-diversity data showed similar results across most parameters for the F. australis populations; however, the inbreeding coefficient (FIS) was elevated among some populations, such as CRY and WG (Table 2), indicating high kinship levels, even though it has a relatively high number of private alleles compared to the other Fontainea populations. Despite its small population size, and isolated locality, F. oraria showed relatively high diversity values (AR, HO, HE) and a negligible inbreeding coefficient (Table 2). Coffs Harbour Fontainea showed considerably lower values of genomic variation (AR, HO, HE) than did F. australis and F. oraria, with a relatively large number of private alleles (npa), especially when compared with most F. australis populations (Table 2).
Site | Taxon | AR | Size | HO | HE | FIS | npa | |
---|---|---|---|---|---|---|---|---|
CC | F. australis | 1.359 | 11.59 | 0.169 | 0.175 | 0.039 | 182 | |
CRY | F. australis | 1.424 | 13.43 | 0.183 | 0.209 | 0.106 | 376 | |
EH | F. australis | 1.366 | 9.48 | 0.173 | 0.183 | 0.043 | 224 | |
HB | F. australis | 1.319 | 2.87 | 0.170 | 0.158 | –0.094 | 121 | |
KR | F. australis | 1.386 | 12.45 | 0.199 | 0.188 | –0.043 | 110 | |
LW | F. australis | 1.391 | 24.19 | 0.175 | 0.188 | 0.064 | 561 | |
MNP | F. australis | 1.329 | 6.55 | 0.177 | 0.166 | –0.060 | 79 | |
MRR | F. australis | 1.378 | 6.67 | 0.186 | 0.189 | 0.012 | 144 | |
MTC | F. australis | 1.379 | 6.67 | 0.181 | 0.190 | 0.032 | 157 | |
NB | F. australis | 1.375 | 13.52 | 0.167 | 0.183 | 0.069 | 325 | |
NC | F. australis | 1.346 | 6.69 | 0.175 | 0.170 | –0.037 | 259 | |
WG | F. australis | 1.413 | 19.08 | 0.180 | 0.206 | 0.114 | 442 | |
FCH | F. sp. Coffs Harbour A | 1.147 | 35.81 | 0.077 | 0.073 | 0.014 | 468 | |
FO | F. oraria | 1.434 | 9.59 | 0.217 | 0.214 | –0.006 | 509 |
Morphometric analysis
PCA analysis of leaf traits without predefined group assignments did not show any clear group structure among the three Fontainea groups on the basis of the seven leaf traits (Fig. 5a). By contrast, the CDA of leaf characters showed distinct clustering of this Fontainea complex (Fig. 5b). There was clear separation of F. australis and the putative new entity, Coffs Harbour Fontainea, along the first axis, and the CDA also illustrated some overlap in morphometric characters between F. australis and F. oraria (Fig. 5b).
Principal-component analysis (PCA) of two Fontainea species and the undescribed entity from Coffs Harbour performed using seven leaf traits and without prior group assignment (a) and a Canonical discriminant analysis (CDA) of three Fontainea taxa (aust, F. australis – red; coffs, Coffs Harbour Fontainea – green; or, F. oraria – blue) highlighting morphometric cluster groupings on the basis of seven leaf traits with a leave-one-out cross-validation (b).
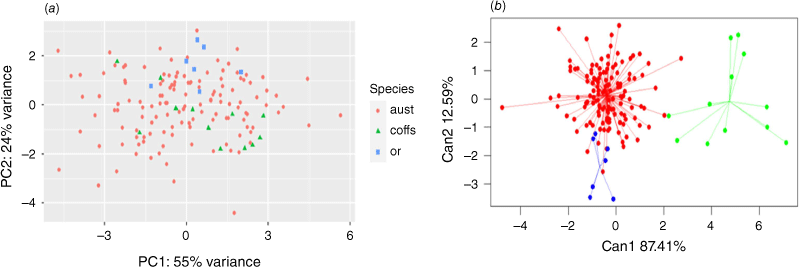
Generally, the series of classification analyses showed similar results and a good ability to classify the three groups within this Fontainea complex at multiple levels by using an LDA and KNN approach. At the population level (Table 3), several F. australis samples were classified with F. oraria, including some individuals from the Mooball region (KR, MRR) and the WG population. LDA at the taxon level (Table S3) classified ~9% of F. australis as F. oraria, and one sample each of Coffs Harbour Fontainea and F. oraria as F. australis.
Population | Taxon | N | as.australis | as.coffs | as.oraria | n correct | Percentage correct | |
---|---|---|---|---|---|---|---|---|
c1 | F. sp. Coffs Harbour | 11 | 1 | 10 | 0 | 10 | 90.91 | |
c2 | F. sp. Coffs Harbour | 1 | 0 | 1 | 0 | 1 | 100 | |
CC | F. australis | 13 | 13 | 0 | 0 | 13 | 100 | |
CRY | F. australis | 13 | 13 | 0 | 0 | 13 | 100 | |
EH | F. australis | 10 | 10 | 0 | 0 | 10 | 100 | |
HB | F. australis | 3 | 3 | 0 | 0 | 3 | 100 | |
KR | F. australis | 13 | 10 | 0 | 3 | 10 | 76.92 | |
LW | F. australis | 25 | 25 | 0 | 0 | 25 | 100 | |
MNP | F. australis | 4 | 4 | 0 | 0 | 4 | 100 | |
MRR | F. australis | 7 | 5 | 0 | 2 | 5 | 71.43 | |
MTC | F. australis | 7 | 7 | 0 | 0 | 7 | 100 | |
NB | F. australis | 17 | 14 | 0 | 3 | 13 | 82.35 | |
NC | F. australis | 7 | 6 | 1 | 0 | 6 | 85.71 | |
WNG | F. australis | 14 | 11 | 0 | 3 | 11 | 78.57 | |
FO | F. oraria | 7 | 1 | 0 | 6 | 6 | 85.71 | |
Total | 152 | 123 | 12 | 17 | 138 | 90.79 |
LDA results at the taxon level also showed a high proportion (>90%) of accurate classifications for F. australis and F. sp. Coffs Harbour and classified one of the seven F. oraria samples with F. australis (Table S3). KNN analysis showed that the highest number of correct classifications was at k = 4 (Fig. S2) and reflected the LDA results, with some F. australis individuals classified as F. oraria, several F. sp. Coffs Harbour individuals classified with F. australis, and >85% of F. oraria classified as F. australis (Table 4).
Taxon | N | as.australis | as.coffs | as.oraria | n correct | Percentage correct | |
---|---|---|---|---|---|---|---|
F. australis | 133 | 131 | 0 | 2 | 131 | 98.50 | |
F. sp. Coffs Harbour | 12 | 3 | 9 | 0 | 9 | 75.00 | |
F. oraria | 7 | 6 | 0 | 1 | 1 | 14.29 | |
Total | 152 | 140 | 9 | 3 | 141 | 92.76 |
Conditional-inference trees showed the three Fontainea groups were best characterised by two (SLA, DLM) of the seven leaf traits used in the analysis. Fontainea sp. Coffs Harbour was best characterised by low SLA, and at low SLA, is distinguished from some F. australis classifications by high DLM (>0.351, Fig. 6). For the larger SLA (>0.027–0.065), all samples were classified as F. australis, but with a 3.4% error, with a small number of samples each from F. oraria and Coffs Harbour Fontainea being misclassified (Fig. 6). For SLA >0.065, there was a roughly equal classification between F. australis and F. oraria, albeit with a small number of samples (n = 9).
Conditional-inference tree examining three Fontainea taxa (aust, F. australis; coffs, F. sp. Coffs Harbour; or, F. oraria), showing specific leaf area (SLA) and dry leaf mass (DLM) as the best traits to distinguish among species from seven leaf characters used as explanatory variables for classification.
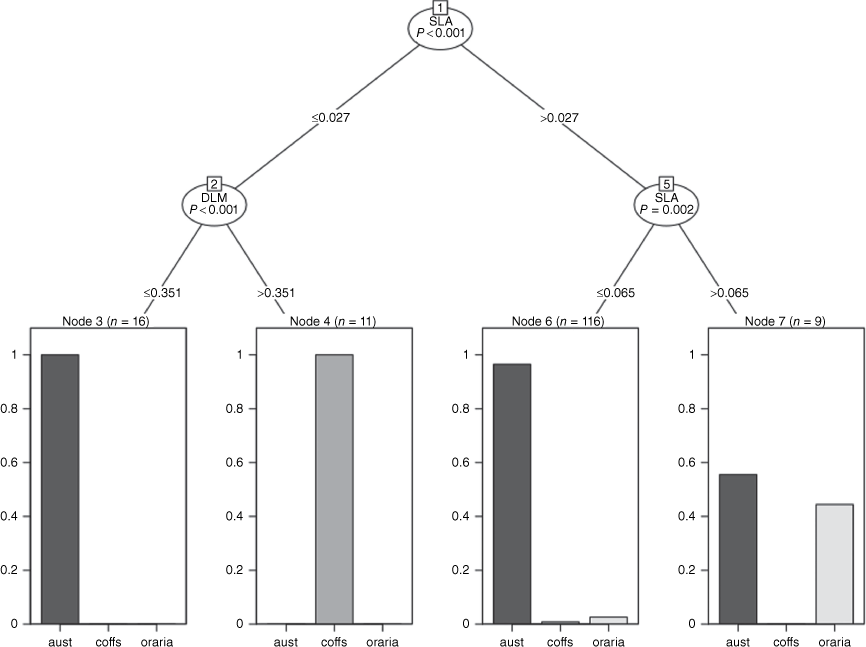
In addition to the conditional inference-tree results of SLA and DLM as key traits to classify among the three Fontainea groups, significance tests showed that three morphological characters (PL, SLA and DLM, Fig. 7a–c) were able to distinguish among the groups (P ≤ 0.05), namely, PL (P = 0.0417), SLA (P ≤ 0.0001) and DLM (P ≤ 0.0007). Variation among groups was supported in post hoc calculations of the mean 95% confidence interval for each significant trait (Table S4). These showed that for SLA, there was significant variation among all three Fontainea taxa (P ≤ 0.001). For DLM there was also significant (P = 0.008) difference among Coffs Harbour Fontainea, F. oraria and F. australis. However, there was a significant difference for PL between only Coffs Harbour Fontainea and F. australis. The remaining leaf characters (LL, LW, LA, and LR) showed no significant variation among species, although a weak relationship (P = 0.098) for LRTO was detected (Fig. S3).
Discussion
In this study, we used operational criteria that combined molecular-based analysis with results from morphometric analysis of seven leaf characters to delimit genetic and morphometric boundaries of three, narrow-range endemic rainforest shrubs. The close genetic relationships of F. australis and F. oraria, together with a recent discovery of a new Fontainea population beyond the known distribution of the genus, necessitated a focus that included morphological differentiation. We found that inter-population genetic and leaf variation were able to provide a somewhat clearer understanding of the species limits and evolutionary lineages within this complex of the threatened Fontainea. DNA-based analyses were relatively congruent with morphometric analyses and provided evidence in support of the recently discovered Fontainea population as a distinct evolutionary lineage. However, our results also showed further insights into the challenging boundaries between F. australis and F. oraria populations as well as the new Coffs Harbour entity. Although our data indicated that there are likely to be three distinct lineages, the intricate relationships among these lineages complicates interpretation, leaving open the possibility that they may constitute a single species. With unresolved geographic groups posing further challenges and the discovery of a new entity, we suggest that a renewed taxonomical description is necessary for the three Fontainea groups and would have significant implications for conservation priorities within the complex.
Genetic support of described species boundaries
Our extensive exploration of this Fontainea complex identified several distinct clusters, and delimitation among them on the basis of genomic characters. In relation to the genetic–species boundaries of F. australis and F. oraria, our analyses showed that the phylogenetic and morphological distinction between some F. australis populations and F. oraria are not so well defined. Our SNP-based phylogeny showed that F. australis and F. oraria share close affinities. The phylogeny highlighted that the most easterly F. australis populations from the Mooball area (MRR, MNP, KR), as well as two populations at the southern extent of F. australis (WG, HB), could represent a geographically separated entities that are genetically distinct from the typical forms found further inland. Genetic differentiation (FST) between the Mooball populations and the inland populations of F. australis was not as strong as at inter-species levels, yet PCA results highlighted similar genomic divergence relationships that were also reflected in the phylogenetic topology. These findings provided strong support to delineate the Mooball cluster and two populations in the Wanganui gorge (WG, HB) from the remaining populations of F. australis. This evidence could indicate they be recognised as a subspecies of F. australis. However, this raises a question about the genetic relationships among the other related groups. On the basis of genetic evidence, the classification of one entity as a distinct group with a subspecies (F. australis), while designating F. oraria and the Fontainea entity from Coffs Harbour as separate species might be questionable. Whereas some genetic results support the presence of three distinct lineages within F. australis, F. oraria, and the Coffs Harbour taxon, additional data such as IBD and pairwise FST values (genetic differentiation), could potentially challenge the traditional classification scheme and prompt a re-evaluation of how these species are categorised from a genetic perspective.
Genetic structure generally reflected the geographically fragmented and isolated range of F. australis, F. oraria and the Coffs Harbour Fontainea, with signals to indicate limited geneflow, which might be driving genetic drift along the latitudinal distribution of the three groups. This geographic structuring agrees with Brunton et al. (2024), who showed that reciprocal admixture was more frequent in the collected localities of F. australis closest to F. oraria, which included the Mooball cluster as well as WG and HB, to suggest that geneflow among the taxa may have occurred relatively recently. This scenario would likely have involved intermediate, relict populations in the ‘Big Scrub’, an area of subtropical rainforest (Complex Notophyll Vine Forest) that once covered up to 750 km2 of the deep, volcanic soils of the Alstonville plateau in northern NSW, from the high inland elevations, to within a few kilometres of the current coastline (Parkes et al. 2012). The fact that expected heterozygosity (HE) was higher in the smallest population (F. oraria) also supports recent admixed origins. Extensive clearing since the 1840s means that <1% of its original habitat extent remains as small, scattered rainforest fragments (Parkes et al. 2012), and the possibility of contemporary geneflow that includes novel, undiscovered F. australis populations within this region is highly unlikely. However, in the case of Coffs Harbour Fontainea relative to the other taxa, clear genetic differentiation suggests that recent geneflow from its northern congeners is unlikely.
Although some genetic evidence supported the presence of three distinct lineages within F. australis, F. oraria, and the Coffs Harbour taxon, the challenge in classifying each group as a distinct species stems from our broader genetic data, which showed an IBD pattern of genomic divergence. This IBD pattern could suggest that the three taxa represent a species continuum resulting from geographic partitioning, adding complexity to the interpretation of the evolutionary relationships among these Fontainea entities. We observed a north–south genetic cline, tied to the rainforest environment biome envelope where the F. australis populations in the northern extent of its range are genetically clustered. Signals of genetic variation indicated that there is emerging genetic drift driving the divergence of the central populations, which are isolated in refugia of high elevations (NC), deep gorges (HB, WG), and the most easterly situated (Mooball) populations. Fontainea oraria remains isolated within a small, coastal rainforest patch within 1 km of the ocean, and there is a large break of almost 200 km, which includes numerous pronounced geographic barriers, separating the newly discovered Fontainea at Coffs Harbour. Although this result is unsurprising, recent landscape genetic analysis have suggested that environmental and geographic factors play a distinct role in the intraspecific diversity of F. australis and F. oraria (Brunton et al. 2024). Therefore, we could infer that this effect is also a key factor in the genetic divergence of the putative new species F. sp. Coffs Harbour and this entity is a highly diverged geographic race within this complex.
Morphological distinctions for taxonomic delineation
Species identification keys for Fontainea can be difficult to use for field collections because they are mainly based on reproductive features (Jessup and Guymer 1985; Forster 1997; Forster and Van Welzen 1999), which are not always present. To overcome this, leaf traits can offer an objective and independent approach to test the circumscription of taxonomic entities, keeping in mind variation owing to phenotypic expression. Our morphometric results largely corresponded to the current taxonomic treatments in identifying three taxa (albeit preliminary in the case of F. sp. Coffs Harbour). We tested a range of leaf characters that are generally not used in classical systematic treatments; however, metrics such as SLA, PL and DLM have been useful in demonstrating inter-species variation in a number of global (Duminil et al. 2012; Buzatti et al. 2019) and Australian (Wright et al. 2002, 2004) contexts. In this study, morphometric classifications showed that several leaf characters (SLA, DLM and PL) could be used to distinguish among some Fontainea species. Yet, phenological patterns were not well-resolved for all species and may not be regular to highlight the challenge of using only a morphometric approach in a set of plastic traits. This difficulty in distinguishing clear boundaries with leaf traits, therefore, further complicates the task of achieving conclusive species delineation solely on the basis of morphological characteristics used for this study.
For example, our results showed some mixed classifications between F. australis and F. oraria on the basis of the complete set of leaf characters. Leaf traits associated with light capture, such as SLA, were lower in Coffs Harbour Fontainea, which is likely to reflect the variation in local environment among the collection sites for each species. Fontainea australis and F. oraria are located in sites with climatic conditions favourable to plant productivity, such as high annual precipitation and high summer temperatures (Brunton et al. 2023), and showed generally higher values for high SLA and DLM, which signals growth traits in response to these conditions. However, we also found some cases within the classifications of F. australis related to lower SLA, and a handful of examples where all three species were classified together. This complex pattern of morphometric variance was also highlighted by the range of classification analyses that assigned some cases from the Mooball populations with F. oraria, and at various levels (taxon, population and individual) classified F. oraria with F. australis. This may lend support to a subspecies classification of the Mooball populations on the basis of a definition by Stebbins (1950) that suggests only one or several minor differences in characters (in this case SLA, PL, DLM), and an intermediate population (F. oraria) should occur that exhibits a link in character states. In addition, the distinct clustering of these regional populations as a genetic subgroup endorses the recognition of the Mooball population as a geographical race of F. australis that is in line with another subspecies definition by Stace (1991). Where we observed this mixed pattern of species classification may be best explained by the plasticity in leaf shape among the three species. We found numerous leaf forms both within and among the three species from our samples that are reflected even in the small number of species representatives presented in Fig. 2. This clearly showed that each taxon contains a range of leaf shapes from elliptic to oblanceolate, or ovate, which may be difficult to distinguish in the field. The challenge arises when character states overlap to an extent where they cannot be effectively utilised in a key, making it notably difficult to describe and recognise the taxa in the field.
Plasticity in leaf characteristics is distinct in the southern clade of Fontainea, unlike for other species in the genus, aligning with patterns observed in various tropical rainforest species (Wright et al. 2004; Rozendaal et al. 2006). This adaptability, influenced by factors such as climate, light conditions and extreme seasonal variations, is notable in the context of the distribution of this Fontainea clade across an environmentally complex landscape. Despite our data supporting taxonomic groupings based on morphological features within the Fontainea complex, uncertainties persist, particularly regarding certain geographic groups within F. australis–F. oraria. Plastic leaf traits are evident in all three groups, emphasising the need for comparison in a controlled environment (such as a common garden experiment) or when lineages coexist, which is not the case here. So, overall, the predominant signal suggests a pattern of geographic separation and drift in leaf morphology, necessitating further exploration of floral and fruiting characteristics for a conclusive taxonomic determination to support a theory of three distinct taxa.
Overall, our study has highlighted the inherent challenge in defining species boundaries among genetically and morphologically similar taxa, especially in the absence of reproductive material. Even though we found correlations between certain leaf characters and genetically diverged groups, particularly for the Coffs Harbour entity, the evidence remains inconclusive for the separation of F. australis and F. oraria. We suggest here that the pattern of genomic divergence and morphological variability among the three Fontainea taxa represents a continuum structured by prominent breaks in gene flow related to major geographic barriers as well as a complex series of contraction–expansion cycles related to paleoclimatic oscillations (Brunton et al. 2023). Yet, our results supported neither the combination of F. australis with F. oraria in a unified taxon, nor the unique classification of F. australis populations from Mooball and the Wanganui gorge. Nevertheless, we found that there are genetic signals of divergence among both this distinct group of F. australis and F. oraria, which are also represented in the morphometric classifications. Therefore, the complexities of our results do not necessarily support the recognition of a merged species that incorporates the eastern populations of F. australis and F. oraria. However, there is a growing evidence to support a renewed taxonomic revision for these species. Clearer distinction for this sister group would also improve the recovery of evolutionary distinct units that would no doubt offer a range of renewed conservation priorities to enhance the diversity within this narrow, rainforest complex with a significant representation in areas of climate refugia (Brunton et al. 2023).
Conservation of critically endangered and threatened species
There are several Australian examples that have explored the patterns of population divergence using an integrated-data approach to recover the relationships among closely related, threatened species (Robins et al. 2021; Wilson et al. 2022; O’Donnell et al. 2023). These studies highlighted various outcomes, such as subspecies recognition (Robins et al. 2021), designation of new species (O’Donnell et al. 2023) and the merging of some species (Wilson et al. 2022), that all contributed to updated conservation priorities. However, overestimating morphological diversity within species of taxonomic uncertainty can divert attention and has often restricted resources away from populations that are in greater need of conservation focus (Wilson et al. 2022). For the three Fontainea groups in this study, we suggest that they deserve ongoing conservation management to ensure that the species diversity within this already genetically restricted, narrow-range genus is retained. Fontainea oraria is currently undergoing a translocation program aimed at achieving genetic rescue, which has yielded great success in reproductive output and genetic improvements (Rossetto et al. 2023). Although F. australis has a broader distribution and pool of species diversity, the ambiguous relationships between some of their populations and F. oraria stress the importance of maintaining the geographically distinct clusters, prioritising formal protection of more populations through private conservation covenants, or strategically purchasing properties that could expand the current suitable habitat. Habitat protection remains a key conservation focus for all three Fontainea groups in this complex and the lack of formal protection presents the greatest threat to their immediate preservation (Brunton et al. 2023).
Should the new Fontainea entity from Coffs Harbour receive formal description as a new species, it will join five other threatened Australian Fontainea species. This formal description will be crucial in the conservation of this entity, nevertheless its small population size and southern-range extension of the genus support conservation attention. As currently circumscribed, this new population has been assigned a species name, F. sp. Coffs Harbour (National Herbarium of New South Wales 2021), in addition to a provisional Critically Endangered status (PlantNET, see https://plantnet.rbgsyd.nsw.gov.au). This potentially new species is restricted to just two small sites, with a limited number (<5) of mature, reproductive individuals that are subject to ongoing disturbance from road-construction activities. One site contains only a single, mature fruit-producing tree, which is designated for translocation to allow its current habitat to be cleared for a major road upgrade. Importantly, our results indicated that Coffs Harbour Fontainea had low levels of genomic variation compared with F. australis and F. oraria; yet, despite our expectations of a high degree of inter-relatedness, no evidence was found to indicate inbreeding among the limited number of individuals. This suggests that even though the small number of reproductively mature plants represent an adequate number of unrelated individuals or have self-mating limitations, a genetic-rescue program for Fontainea from Coffs Harbour with individuals of low levels of mean kinship will be crucial for its conservation.
Finally, the genetic and morphometric evidence we present has provided information to warrant further taxonomic attention to F. australis and F. oraria. This would determine whether systematic treatments support the signals of genomic and morphometric divergence found in this study or support a single species theory, with distinctions resulting from isolation by distance as result of genetic drift. If a future taxonomic revision determines that F. oraria merely represents a divergent F. australis population, it will not significantly extend the range or genetic diversity of that species. Therefore, the natural population of F. oraria remains a key conservation focus. A number of additional sites from translocated material has been established for this species (Brown et al. 2017); however, more attention is warranted in identifying potential additional translocation areas (Brunton et al. 2023). Similar to F. oraria, material has been captured to develop an ex situ population that can be used to enhance the genetic profile for the Coffs Harbour Fontainea, which is in an extreme risk of extinction, and where appropriate, provide material for translocation to suitable habitats.
Conclusions
So as to develop effective conservation actions and priorities for taxa facing a high risk of extinction, establishing clear systematic associations and species boundaries is crucial. This study aimed to enhance the species delimitation for a group of closely related subtropical rainforest plants by integrating genetic data and morphometrics. Although our results could potentially support the merging of F. australis, F. oraria, and the Coffs Harbour taxa into a geographically diverged, single species, it is important to recognise that this hypothesis requires further research. It is essential to approach these taxonomic challenges cautiously, acknowledging the possibility of alternative interpretations of the data. In light of the theory proposing that all three entities belong to the same species, our data as presented here, may not decisively refute this additional hypothesis. Similarly, the data could alternatively be interpreted as indicative of three distinct lineages or potentially separate species, an observation often made in geographically distinct, yet genetically linked, populations experiencing isolation by distance. The absence of distinguishing characters in the morphological data presented here adds to the challenge of definitively supporting the existence of multiple species. Although we recognise the possibility that distinguishing characters may exist in the reproductive parts of the plants, such evidence is not presented in this study; however, this element warrants further attention. Given the complexities and the absence of conclusive evidence, reframing the interpretation of our results to be less definitive in supporting the existence of multiple species would provide a more accurate depiction. Overall, our study underscores the importance of integrating various data sources to refine species boundaries among cryptic groups, improve taxonomic classifications, and unveil new insights into the evolutionary relationships among closely related plant species.
Data availability
The raw data that support this study will be made publicly available at https://github.com/AaronBrunton/Mol_moprho_article_data upon publication.
Conflicts of interest
A. J. Brunton reports that financial support was provided by QBiotics Group Limited Australia. S. M. Ogbourne reports a relationship with QBiotics Group Limited Australia that includes: board membership, employment, and equity or stocks. The authors declare that they have no further financial interests or personal relationships that may be considered potential conflicts of interest.
Declaration of funding
A. J. Brunton was assisted by a Research Training Program scholarship and gratefully thanks QBiotics Group Ltd and the UniSC–Rotary scholarship for providing research funding.
Acknowledgements
We thank Dr Andrew Benwell, QPWS, NSW National Parks, and TNSW for their assistance with field collections. We gratefully acknowledge the Yugambeh, Bundjalung and Gumbaynggirr people who are the First Nations custodians of the land on which this research was conducted.
References
Baraloto C, Bonal D, Goldberg DE (2006) Differential seedling growth response to soil resource availability among nine neotropical tree species. Journal of Tropical Ecology 22, 487-497.
| Crossref | Google Scholar |
Bradbury D, Binks RM, Webb A, Byrne M (2023) Defining conservation units in a species complex with genomic-taxonomic discordance: a case study of Conospermum caeruleum (Proteaceae). Biodiversity and Conservation 32, 1949-1975.
| Crossref | Google Scholar |
Brown D, James R, Mckinley A (2017) Fontainea dude thinks he’s a lady-recovery of the coastal Fontainea and investigation into temporal monoecy. Australasian Plant Conservation: Journal of the Australian Network for Plant Conservation 25, 11-13.
| Crossref | Google Scholar |
Brunton AJ, Lamont RW, Conroy GC, Yap S, Rossetto M, Taylor-Brown A, Maggia L, Reddell PW, Ogbourne SM (2022) Phylogenetic reconstruction of the rainforest lineage Fontainea Heckel (Euphorbiaceae) based on chloroplast DNA sequences and reduced-representation SNP Markers. Diversity 14, 725.
| Crossref | Google Scholar |
Brunton AJ, Conroy GC, Schoeman DS, Rossetto M, Ogbourne SM (2023) Seeing the forest through the trees: applications of species distribution models across an Australian biodiversity hotspot for threatened rainforest species of Fontainea. Global Ecology and Conservation 42, e02376.
| Crossref | Google Scholar |
Brunton AJ, Farleigh K, Ogbourne SM, Rossetto M, Schoeman DS, Conroy GC (2024) The geno-geo-climate nexus: contributions of geographic and ecological factors in shaping the genomic divergence of two closely related threatened rainforest species of Fontainea Heckel (Euphorbiaceae). Landscape Ecology 39, 11.
| Crossref | Google Scholar |
Buzatti R, Pfeilsticker TR, Muniz AC, Ellis VA, de Souza RP, Lemos-Filho JP, Lovato MB (2019) Disentangling the environmental factors that shape genetic and phenotypic leaf trait variation in the tree Qualea grandiflora across the Brazilian savanna. Frontiers in Plant Science 10, 1580.
| Crossref | Google Scholar | PubMed |
Carstens BC, Pelletier TA, Reid NM, Satler JD (2013) How to fail at species delimitation. Molecular ecology 22, 4369-4383.
| Crossref | Google Scholar | PubMed |
Chaves MM, Pereira JS, Maroco J, Rodrigues ML, Ricardo CP, Osório ML, Carvalho I, Faria T, Pinheiro C (2002) How plants cope with water stress in the field? Photosynthesis and growth. Annals of Botany 89, 907-916.
| Crossref | Google Scholar | PubMed |
Chifman J, Kubatko L (2014) Quartet inference from SNP data under the coalescent model. Bioinformatics 30, 3317-3324.
| Crossref | Google Scholar | PubMed |
De Queiroz K (2007) Species concepts and species delimitation. Systematic Biology 56, 879-886.
| Crossref | Google Scholar | PubMed |
Department of Environment, Climate Change and Water NSW (2010) Border Ranges Rainforest Biodiversity Management Plan: NSW & Queensland. March 2010. (DECCW: Sydney, NSW, Australia) Available at https://www.dcceew.gov.au/sites/default/files/documents/brrb-management-plan.pdf
Duminil J, Kenfack D, Viscosi V, Grumiau L, Hardy OJ (2012) Testing species delimitation in sympatric species complexes: the case of an African tropical tree, Carapa spp. (Meliaceae). Molecular Phylogenetics and Evolution 62, 275-285.
| Crossref | Google Scholar | PubMed |
Forster PI (1997) Three new species of Fontainea Heckel (Euphorbiaceae) from Australia and Papua New Guinea. Austrobaileya 5, 29-37.
| Crossref | Google Scholar |
Forster PI, Van Welzen PC (1999) The Malesian species of Choriceras, Fontainea, and Petalostigma (Euphorbiaceae). Blumea-Biodiversity, Evolution and Biogeography of Plants 44, 99-107.
| Google Scholar |
Frankham R, Ballou JD, Dudash MR, Eldridge MDB, Fenster CB, Lacy RC, Mendelson III JR, Porton IJ, Ralls K, Ryder OA (2012) Implications of different species concepts for conserving biodiversity. Biological Conservation 153, 25-31.
| Crossref | Google Scholar |
Fujita MK, Leaché AD, Burbrink FT, Mcguire JA, Moritz C (2012) Coalescent-based species delimitation in an integrative taxonomy. Trends in Ecology & Evolution 27, 480-488.
| Crossref | Google Scholar | PubMed |
Goudet J (2005) Hierfstat, a package for R to compute and test hierarchical F‐statistics. Molecular Ecology Notes 5, 184-186.
| Crossref | Google Scholar |
Hothorn T, Zeileis A (2015) Partykit: a modular toolkit for recursive partytioning in R. The Journal of Machine Learning Research 16, 3905-3909.
| Google Scholar |
Huson DH (1998) SplitsTree: analyzing and visualizing evolutionary data. Bioinformatics 14, 68-73.
| Crossref | Google Scholar |
Jessup LW, Guymer GP (1985) A revision of Fontainea Heckel (Euphorbiaceae–Cluytieae). Austrobaileya 2, 112-125.
| Crossref | Google Scholar |
Jombart T (2008) adegenet: a R package for the multivariate analysis of genetic markers. Bioinformatics 24, 1403-1405.
| Crossref | Google Scholar | PubMed |
Jones RC, Steane DA, Lavery M, Vaillancourt RE, Potts BM (2013) Multiple evolutionary processes drive the patterns of genetic differentiation in a forest tree species complex. Ecology and Evolution 3, 1-17.
| Crossref | Google Scholar | PubMed |
Keenan K, Mcginnity P, Cross TF, Crozier WW, Prodöhl PA (2013) diveRsity: an R package for the estimation and exploration of population genetics parameters and their associated errors. Methods in Ecology and Evolution 4, 782-788.
| Google Scholar |
Kilian A, Wenzl P, Huttner E, Carling J, Xia L, Blois H, Caig V, Heller-Uszynska K, Jaccoud D, Hopper C, Aschenbrenner-Kilian M, Evers M, Peng K, Cayla C, Hok P, Uszynski G (2012) Diversity Arrays Technology: a generic genome profiling technology on open platforms. In ‘Data Production and Analysis in Population Genomics’. (Eds F Pompanon, A Bonin) Methods in Molecular Biology, vol. 888, pp. 67–89. (Humana Press: Totowa, NJ, USA) 10.1007/978-1-61779-870-2_5
Lambers H, Poorter H (1992) Inherent variation in growth rate between higher plants: a search for physiological causes and ecological consequences. Advances in Ecological Research 23, 187-261.
| Crossref | Google Scholar |
Milla R, Reich PB (2007) The scaling of leaf area and mass: the cost of light interception increases with leaf size. Proceedings of the Royal Society of London – B. Biological Sciences 274, 2109-14.
| Crossref | Google Scholar | PubMed |
National Herbarium of New South Wales (2021) Fontainea sp. Coffs Harbour (A.S.Benwell 341, NSW1102027) NSW Herbarium. In ‘PlantNET Flora of New South Wales Online’. (APNI) Available at https://biodiversity.org.au/nsl/services/rest/name/apni/51406166
O’Donnell RP, Bruhl JJ, Telford IRH, Wilson TC, Zimmer HC, Taseski GM, Andrew RL (2023) Molecular and morphological analyses support recognition of Prostanthera volucris (Lamiaceae), a new species from the Central Tablelands of New South Wales. Australian Systematic Botany 36, 1-20.
| Crossref | Google Scholar |
Parkes T, Delaney M, Dunphy M, Woodford R, Bower H, Bower S, Bailey D, Joseph R, Nagle J, Roberts T (2012) Big Scrub: a cleared landscape in transition back to forest? Ecological Management & Restoration 13, 212-223.
| Crossref | Google Scholar |
Peterson BK, Weber JN, Kay EH, Fisher HS, Hoekstra HE (2012) Double Digest RADseq: an inexpensive method for de novo SNP discovery and genotyping in model and non-model species. PLoS ONE 7(5), e37135.
| Crossref | Google Scholar |
Pérez-Harguindeguy N, Díaz S, Garnier E, Lavorel S, Poorter H, Jaureguiberry P, Bret-Harte MS, Cornwell WK, Craine JM, Gurvich DE, Urcelay C, Veneklaas EJ, Reich PB, Poorter L, Wright IJ, Ray P, Enrico L, Pausas JG, de Vos AC, Buchmann N, Funes G, Quétier F, Hodgson JG, Thompson K, Morgan HD, ter Steege H, Sack L, Blonder B, Poschlod P, Vaieretti MV, Conti G, Staver AC, Aquino S, Cornelissen JHC (2013) New handbook for standardised measurement of plant functional traits worldwide. Australian Journal of Botany 61, 167-234.
| Crossref | Google Scholar |
Poorter H, De Jong R (1999) A comparison of specific leaf area, chemical composition and leaf construction costs of field plants from 15 habitats differing in productivity. New Phytologist 143, 163-176.
| Crossref | Google Scholar |
Revell LJ (2012) phytools: an R package for phylogenetic comparative biology (and other things). Methods in Ecology and Evolution 3, 217-223.
| Crossref | Google Scholar |
Robins TP, Binks RM, Byrne M, Hopper SD (2021) Contrasting patterns of population divergence on young and old landscapes in Banksia seminuda (Proteaceae), with evidence for recognition of subspecies. Biological Journal of the Linnean Society 133, 449-463.
| Crossref | Google Scholar |
Rossetto M, Mcnally J, Henry RJ, Hunter J, Matthes M (2000) Conservation genetics of an endangered rainforest tree (Fontainea oraria–Euphorbiaceae) and implications for closely related species. Conservation Genetics 1, 217-229.
| Crossref | Google Scholar |
Rossetto M, Bragg J, Brown D, Van Der Merwe M, Wilson TC, Yap J-YS (2023) Applying simple genomic workflows to optimise practical plant translocation outcomes. Plant Ecology 224, 803-816.
| Crossref | Google Scholar |
Rossetto M, Bragg J, Kilian A, McPherson H, van der Merwe M, Wilson PD (2019) Restore and Renew: a genomics‐era framework for species provenance delimitation. Restoration Ecology 27(3), 538-548.
| Crossref | Google Scholar |
Rozendaal DMA, Hurtado VH, Poorter L (2006) Plasticity in leaf traits of 38 tropical tree species in response to light; relationships with light demand and adult stature. Functional Ecology 20, 207-216.
| Crossref | Google Scholar |
Rumpunen K, Bartish IV (2002) Comparison of differentiation estimates based on morphometric and molecular data, exemplified by various leaf shape descriptors and RAPDs in the genus Chaenomeles (Rosaceae). Taxon 51, 69-82.
| Crossref | Google Scholar |
Sansaloni C, Petroli C, Jaccoud D, Carling J, Detering F, Grattapaglia D, Kilian A (2011) Diversity Arrays Technology (DArT) and next-generation sequencing combined: genome-wide, high throughput, highly informative genotyping for molecular breeding of Eucalyptus. BMC Proceedings 5, P54.
| Crossref | Google Scholar |
Schliep KP (2011) phangorn: phylogenetic analysis in R. Bioinformatics 27, 592-593.
| Crossref | Google Scholar | PubMed |
Schneider CA, Rasband WS, Eliceiri KW (2012) NIH Image to ImageJ: 25 years of image analysis. Nature Methods 9, 671-675.
| Crossref | Google Scholar | PubMed |
Silva MFS, De Andrade IM, Mayo SJ (2012) Geometric morphometrics of leaf blade shape in Montrichardia linifera (Araceae) populations from the Rio Parnaíba Delta, north-east Brazil. Botanical Journal of the Linnean Society 170, 554-572.
| Crossref | Google Scholar |
Sites JW, Jr, Marshall JC (2004) Operational criteria for delimiting species. Annual Review of Ecology, Evolution, and Systematics 35, 199-227.
| Crossref | Google Scholar |
Šlenker M, Koutecký P, Marhold K (2022) MorphoTools2: an R package for multivariate morphometric analysis. Bioinformatics 38, 2954-2955.
| Crossref | Google Scholar | PubMed |
Steane DA, Nicolle D, Sansaloni CP, Petroli CD, Carling J, Kilian A, Myburg AA, Grattapaglia D, Vaillancourt RE (2011) Population genetic analysis and phylogeny reconstruction in Eucalyptus (Myrtaceae) using high-throughput, genome-wide genotyping. Molecular Phylogenetics and Evolution 59, 206-224.
| Crossref | Google Scholar | PubMed |
Ullah S, Skidmore AK, Groen TA, Schlerf M (2013) Evaluation of three proposed indices for the retrieval of leaf water content from the mid-wave infrared (2–6 μm) spectra. Agricultural and Forest Meteorology 171, 65-71.
| Crossref | Google Scholar |
van Welzen PC, Arias Guerrero S, Arifiani D, Bangun TJF, Bouman RW, Eurlings MCM, Gushilman I, Phillipson PB, Tabak I, Winkel E, Wurdack KJ (2021) Weda, a new genus with two new species of Euphorbiaceae–Crotonoideae from Halmahera (North Maluku, Indonesia) and phylogenetic relationships of the Australasian tribe Ricinocarpeae. Journal of Systematics and Evolution 59, 1000-1017.
| Crossref | Google Scholar |
Viscosi V, Cardini A (2011) Leaf morphology, taxonomy and geometric morphometrics: a simplified protocol for beginners. PLoS ONE 6, e25630.
| Crossref | Google Scholar | PubMed |
Viscosi V, Lepais O, Gerber S, Fortini P (2009) Leaf morphological analyses in four European oak species (Quercus) and their hybrids: a comparison of traditional and geometric morphometric methods. Plant Biosystems 143, 564-574.
| Crossref | Google Scholar |
Wilson TC, Rossetto M, Bain D, Yap JS, Wilson PD, Stimpson ML, Weston PH, Croft L (2022) A turn in species conservation for hairpin banksias: demonstration of oversplitting leads to better management of diversity. American Journal of Botany 109, 1652-1671.
| Crossref | Google Scholar | PubMed |
Wright S (2016) Using DECIPHER v2. 0 to analyze big biological sequence data in R. The R Journal 8, 352-359.
| Crossref | Google Scholar |
Wright IJ, Westoby M, Reich PB (2002) Convergence towards higher leaf mass per area in dry and nutrient‐poor habitats has different consequences for leaf life span. Journal of Ecology 90, 534-543.
| Crossref | Google Scholar |
Wright IJ, Groom PK, Lamont BB, Poot P, Prior LD, Reich PB, Schulze ED, Veneklaas EJ, Westoby M (2004) Leaf trait relationships in Australian plant species. Functional Plant Biology 31, 551-558.
| Crossref | Google Scholar | PubMed |
Wright IJ, Dong N, Maire V, Prentice IC, Westoby M, Díaz S, Gallagher RV, Jacobs BF, Kooyman R, Law EA, Leishman MR, Niinemets Ü, Reich PB, Sack L, Villar R, Wang H, Wilf P (2017) Global climatic drivers of leaf size. Science 357, 917-921.
| Crossref | Google Scholar | PubMed |
Zachos FE (2018) Mammals and meaningful taxonomic units: the debate about species concepts and conservation. Mammal Review 48, 153-159.
| Crossref | Google Scholar |
Zheng H, Fan L, Milne RI, Zhang L, Wang Y, Mao K (2017) Species delimitation and lineage separation history of a species complex of aspens in China. Frontiers in Plant Science 8, 375.
| Crossref | Google Scholar | PubMed |