The role of grass-tree Xanthorrhoea semiplana (Asphodelaceae) canopies in temperature regulation and waterproofing for ground-dwelling wildlife
Sophie Petit
A UniSA STEM, University of South Australia, Mawson Lakes, SA 5095, Australia.
B Kangaroo Island Research Station, Dudley West, Penneshaw, SA 5222, Australia.
Pacific Conservation Biology 29(5) 445-455 https://doi.org/10.1071/PC23014
Submitted: 6 March 2023 Accepted: 18 July 2023 Published: 8 August 2023
Abstract
The iconic grass-trees (Xanthorrhoea semiplana) of Australia are used by many animal species, but their role as shelters against weather extremes is poorly known. The severe contribution of the fungal pathogen Phytophthora cinnamomi to grass-tree deaths and current burning practices could affect small animal conservation by exacerbating impacts of weather extremes.
We examined the buffering role of X. semiplana canopies against extreme weather at four sites in the Mount Lofty Ranges, South Australia.
We measured ambient temperature, temperatures under grass-tree canopies, and 2 m away at random locations in summer (>35°C) and in winter (<13°C) over 24-h periods at each study site. We scored soil dryness under the canopies during and after heavy rainfall.
Temperatures under grass-tree canopies were more stable and with smaller ranges than other temperatures, and showed dramatic differences in summer when conditions were up to 20°C cooler than ambient. Temperatures were higher under grass-trees at night in winter. The soil under the largest canopies was completely dry during and after heavy rainfall.
Xanthorrhoea semiplana buffers ground-dwelling animals against temperature extremes and rain so that the animals maintain their thermal resistance. Animals may choose foraging times based on grass-tree availability. The largest grass-trees provide the best shelter.
Considerable grass-tree deaths from P. cinnamomi infestation, together with removal or burning, can have dramatic detrimental consequences for their habitat value and the survival of wildlife using them as shelter.
Keywords: bushfire, concealment from predators, cover for wildlife, foraging time, hazard reduction burning, microclimate regulation, prescribed burning, protection from rain, refuge, shade, shelter plant, thermoregulation, tree hollow, waterproofing, wildfire.
Introduction
Physiological and ecological processes influence an animal’s habitat selection (Huey 1991). Shelter selection affects growth, survival and reproduction (Huey 1991), and can decrease the cost of thermoregulation (Wilkinson et al. 1998; Bos and Carthew 2003). The choice of an appropriate shelter is especially important in stressful climatic conditions, when temperature and relative humidity can reach fatal levels (Waudby and Petit 2017). Sunday et al. (2014) showed that sheltering is vital for ectotherms because extreme operative body temperatures in exposed habitats could exceed their physiological thermal limits. Shelters are also essential to protect animals from predators, which include exotic cats and foxes in Australia (Bleicher and Dickman 2020). The waterproofing value of shelters has received scant attention, yet the effect of wetting on the insulation of bird and mammal coats is dramatic, because it reduces thermal resistance by approximately half of its dry value (Webb and King 1984). Although small mammals benefit from adaptations that may assist in waterproofing (e.g. sebum – Smith and Thiboutot 2008), it is likely that long exposure to rain would have detrimental impacts on many small vertebrates if they had no access to suitable shelters. Similarly, many unsheltered invertebrates would be negatively affected by heavy rainfall.
The quality of ground vegetation that moderates temperature and wind, and that protects animals from rain and predators should have a great impact on the biodiversity of small vertebrates using an area. Not all plants perform the same services. For example, Frazer and Petit (2007) determined that the bush rat (Rattus fuscipes) selected grass-trees (Xanthorrhoea semiplana F. Muell.) over other understorey vegetation for shelter while foraging. Haby et al. (2013) also found a preference of the Endangered southern brown bandicoot (Isoodon obesulus obesulus) for X. semiplana habitat. In this paper, we explore the temperature and waterproofing properties of X. semiplana as shelter vegetation.
The endemic and iconic Australian genus, Xanthorrhoea (Asphodelaceae, formerly Xanthorrhoeaceae) is represented by 29 species (World Flora Online 2022); individuals of some of these species have lived for hundreds of years (Lewis 1955; Gill and Ingwersen 1976; Lamont and Downes 1979). Their stems may be arborescent or subterranean. A single inflorescence per apex consists of a long wooden scape, topped by a spike bearing numerous nectar-rich flowers; the dry scape of some species is an important nesting site for the threatened green carpenter bee (Xylocopa aeratus) (Leys 2000; Steen and Schwarz 2000; Glatz et al. 2015). The grass-trees’ distinctive terminal crowns of fine, long and pointed leaves can provide shelter to a diversity of animals. In some species, including X. semiplana, the leaves of large individuals form a dense curving canopy and create a thick ground cover (Fig. 1). Senescent leaves dry up, but remain in the structure for a long time, unless burnt. The sheltering role of grass-tree canopies has been established for some mammals, for example bush rat (Rattus fuscipes) (Spencer et al. 2005; Frazer and Petit 2007), dasyurids (Garden et al. 2007), southern brown bandicoot (Isoodon obesulus obesulus) (Paull 1993; Haby et al. 2013; Robinson et al. 2018), several Cercartetus species of pygmy-possums (reviewed in Tulloch 2004), western ringtail possum (Pseudocheirus occidentalis) (Western Australia Department of Parks and Wildlife 2017 and references therein), Kangaroo Island dunnart (Sminthopsis fuliginosus aitkeni) (Gates 2001), mardo (Antechinus flavipes leucogaster) (Swinburn et al. 2007), yellow-footed antechinus (Antechinus flavipes) (Marchesan and Carthew 2004), silver-headed antechinus (Antechinus argentus) (Mason et al. 2017), agile antechinus (Antechinus agilis), bush rat, eastern pygmy-possum (Cercartetus nanus), and white-footed dunnart (Sminthopsis leucopus) (Laidlaw and Wilson 2006), heath rat (Pseudomys shortridgei) (Di Stefano et al. 2011), ash-grey mouse (Pseudomys albocinereus) (Smith et al. 2019), New Holland mouse (Pseudomys novaehollandiae) (Lazenby et al. 2008). A large amount of evidence highlights their use as food (e.g. Wanniarachchi et al. 2022 for some small mammal species) and shelter by many invertebrate and vertebrate species. In a review with a focus on two Queensland species, Borsboom (2005) determined that this evidence concerned over 315 invertebrate species and nearly 100 vertebrate species.
Considering their prevalence in many Australian ecosystems and their dome-shaped structure protecting a wide range of animals (also orchids, Petit and Dickson 2005), some grass-trees species are likely to be keystone (e.g. Wilson et al. 2020, in view of the significant association of small mammals with Xanthorrhoea australis, as in Laidlaw and Wilson 2006, and profound impact of grass-tree habitat loss on vertebrates, as in Cahill et al. 2008). Dependence of animals on grass-trees should be highest where predators are common and when temperature extremes can be fatal. In this study, we examined the temperature microclimate and waterproofing afforded by X. semiplana ssp. semiplana in hot and cold temperature extremes and in torrential rain, respectively. We compared the maximum differences in mean temperature between locations under the canopy of grass-trees and ambient temperature, as well as temperatures at random locations. We expected that grass-trees would buffer high temperatures (shown by Keppel et al. 2017 on the Fleurieu Peninsula), as well as low ones (Swinburn et al. 2007), to a level facilitating the survival of both ectotherms and endotherms. We also determined whether soil dryness under the canopy of grass-trees was independent of canopy size. We expected that the level of protection from temperature and rainfall extremes would increase with increasing size of grass-trees.
This work is of significance because it will contribute to the mounting evidence for grass-trees as keystone species, which are highly susceptible to the key threatening fungal pathogen Phytophthora cinnamomi (Government of South Australia 2006; Commonwealth of Australia 2018), have suffered extensive clearance (Bickford et al. 2008), and are affected by soil compaction in silviculture environments (Ward et al. 2011). In this context, bushfires and prescribed burns are likely to have inordinate interactive or cumulative impacts on fauna by decreasing the protective functions of grass-trees as habitat.
Materials and methods
Study sites
Xanthorrhoea semiplana represents a prevalent mid-stratum habitat in the Mount Lofty Ranges of South Australia (Armstrong et al. 2003), where this study took place in 2005 (Fig. 2). We worked at four sites: (1) Deep Creek National Park (Deep Creek); (2) Scott Creek Conservation Park (Scott Creek); (3) Para Wirra Conservation Park (Para Wirra); and (4) Warren Conservation Park (Warren). Deep Creek (35°39′S, 138°46′E) is located at the southern end of the Fleurieu Peninsula, 100 km south-west of Adelaide. It consists of 4554 ha of sclerophyll open forest and shrubland associations. Two open forest formations are dominated by Eucalyptus obliqua and Eucalyptus fasciculosa; common understorey species include Banksia marginata, Acacia spp., Lepidosperma semiteres, Pultenaea spp, Hibbertia spp, and X. semiplana (DENR 1997). Scott Creek (35°01′S, 138°71′E,) is located 30 km south-east of Adelaide, and supports 706 ha of native vegetation. Eucalyptus obliqua, Eucalyptus cosmophylla, and Eucalyptus baxteri are the dominant overstorey (Schram 1986); common understorey species are similar to those detailed for Deep Creek. Para Wirra (34°43′S, 138°48′E) is located 40 km north-east of Adelaide and covers 1409 ha. Nearby, Warren (34°44′S, 138°54′E) covers 365 ha. Both Para Wirra and Warren support low open E. obliqua forests over common understorey plants of Acacia pycnantha, X. semiplana, Hibbertia exutiacies, and Pultenaea largiflorens (DEP 1991). All study sites are characterised by a Mediterranean climate with warm dry summers and cool wet winters (Table 1).
January | February | March | April | May | June | July | August | September | October | November | December | Average annual | Number of years included | ||
---|---|---|---|---|---|---|---|---|---|---|---|---|---|---|---|
Parawa | |||||||||||||||
Mean maximum temperature (°C) | 23.8 | 23.2 | 21.2 | 18.3 | 14.9 | 12.4 | 11.7 | 12.5 | 14.6 | 16.9 | 19.6 | 21.9 | 17.6 | 28.0 | |
Mean minimum temperature (°C) | 13.8 | 13.9 | 13.0 | 11.3 | 9.6 | 7.7 | 7.0 | 7.0 | 8.0 | 9.0 | 10.7 | 12.1 | 10.3 | 28.0 | |
Mean rainfall (mm) | 27.9 | 30.8 | 33.2 | 56.2 | 97.0 | 122.4 | 126.6 | 103.9 | 81.8 | 61.2 | 40.9 | 36.0 | 827.0 | 26.0 | |
Mount Barker | |||||||||||||||
Mean maximum temperature (°C) | 27.3 | 26.8 | 24.7 | 20.5 | 16.6 | 13.8 | 13.0 | 14.3 | 16.6 | 19.6 | 22.7 | 25.2 | 20.1 | 120.0 | |
Mean minimum temperature (°C) | 12.0 | 12.0 | 10.5 | 8.4 | 6.8 | 5.3 | 4.6 | 5.0 | 5.9 | 7.3 | 9.0 | 10.5 | 8.1 | 121.0 | |
Mean rainfall (mm) | 26.0 | 25.9 | 30.9 | 57.7 | 87.3 | 100.0 | 107.3 | 102.5 | 85.2 | 65.6 | 39.8 | 34.9 | 763.4 | 159.0 | |
Mount Crawford | |||||||||||||||
Mean maximum temperature (°C) | 27.7 | 26.9 | 23.5 | 19.4 | 14.9 | 11.7 | 11.0 | 12.3 | 15.2 | 18.3 | 22.4 | 25.2 | 19.0 | 27.0 | |
Mean minimum temperature (°C) | 14.0 | 14.1 | 12.2 | 10.6 | 8.6 | 6.5 | 5.9 | 6.1 | 7.3 | 8.5 | 10.7 | 12.2 | 9.7 | 27.0 | |
Mean rainfall (mm) | 23.1 | 25.8 | 26.9 | 43.8 | 68.6 | 85.8 | 96.8 | 87.0 | 71.2 | 47.1 | 33.7 | 33.0 | 651.9 | 25.0 |
Temperature under the canopy of grass-trees
At all four study sites, we measured temperature on the ground under single grass-trees (n = 12), which had canopies that were dense enough to cover at least 50% of the ground and were all located on the same slope and aspect. In winter at Warren n = 10 and at Scott Creek n = 11 because some data loggers failed to record. We also measured ambient temperature in the shade and the ground temperature 2 m away from each grass-tree, in a random direction. These points away from the grass-trees were either on bare ground or under other understorey shelter.
We sampled temperature once each in summer and winter, when expected diurnal temperatures in summer were above 35°C, and below 13°C in winter. Temperature was recorded every 2 h over a 24-h period, in time intervals beginning at 08:30 hours. At each grass-tree in summer, we placed an alcohol thermometer (−10°C to 60°C; accuracy ± 0.5°C) on the ground under a dense section of the grass-tree canopy that appeared to provide good cover for vertebrate wildlife, and another at a random point 2 m away selected by spinning a compass dial. We also recorded ambient temperature in the shade (other than that provided by a grass-tree) during the same period. In winter, we used Hygrochron iButtons DS1923 (Dallas Semiconductor Corp., range from −20°C to 85°C, accuracy ± 0.5°C) instead of thermometers, but followed the same procedures. We calculated the mean temperatures for the 12 (or 10 or 11) grass-trees at each time interval. We also calculated the largest difference in mean temperature between ambient and grass-tree, and 2 m away and grass-tree for each site, the temperature range for grass-trees, ambient, and 2 m away, and plotted the temperature data. As a result of the loss of most of the raw data files after compilations of the means, we are unable to present the standard errors other than for Scott Creek in winter. However, these Scott Creek data show very small standard errors. Such small standard errors also appear in Keppel et al. (2017) for grass-trees in summer and Waudby and Petit (2017) for soil cracks in summer, indicating that similar shade types are associated with similar temperatures in the same environment at the same time. We are thus confident that means are fair representations of temperatures. To determine how grass-trees may buffer animals against cold in winter and heat in summer, we compared the maximum differences between the mean temperatures under grass-trees and ambient temperatures at the four sites each season, and did the same with 2-m away temperatures (night in winter, day in summer; see Supplementary Table S1). We used a paired t-test because this test is robust at extremely small sample sizes (de Winter 2013). SPSS (IBM Corp. 2021) was used for all statistical analyses, and we calculated effect size as recommended by Field (2013). The ranges of temperatures in the three different conditions were compared with repeated-measure ANOVA and Bonferroni post-hoc tests, with partial eta squared (ηp2) indicating effect size. Temperature stability increases as the range in temperature decreases.
Soil examination during and after rainfall under grass-tree skirts
We examined the soil under X. semiplana ssp. semiplana at Scott Creek Conservation Park in June 2005. It was the wettest June between 2001 and 2021 at Longwood Alert station (023108), 5.5 km away. It rained a total of 227.4 mm for the month compared to a June mean of 127.3 mm over the 20 years of operation of the station (Bureau of Meteorology 2022c). The study took place after it had rained 141.8 mm over 13 days and during heavy rainfall (Bureau of Meteorology 2005). East of the Bandicoot Track (Gate 3), we walked along a transect line, stopping every 10 m to sample the nearest X. semiplana until we had sampled 60 individuals, 20 in each canopy cover category: 0 (<50% of the ground covered by the canopy), 1 (50–90% of the ground covered), and 2 (>90% of the ground covered; after Frazer and Petit 2007). If we had already sampled 20 grass-trees of a particular canopy cover, and the nearest grass-tree to be measured at the 10-m point had the same canopy cover, we measured the next closest grass-tree in another canopy cover category. At each grass-tree, we parted the canopy and gave the soil under the canopy a score of 0 (dry, will sift through fingers when scooped; no moist clump), 1 (partially dry with wet areas; wet clumps present when soil is scooped), or 2 (saturated). We tested the independence of canopy cover and soil dryness with a two-way chi-squared test; Cramer’s V indicated effect size. We also scored the soil at a randomly selected point 5 m away.
Results
Summer temperatures over 24-h periods under grass-trees
Grass-trees had a large mitigating impact over hot summer temperatures. Over the 24-h period, the mean temperature range under grass-trees was smaller at Warren, Scott Creek, Deep Creek, and Para Wirra, respectively (4.6, 2.4, 1.8, and 3.8°C; overall = 3.1°C ± s.e. 0.6) than it was for 2 m away (27.3, 11.7, 16.9, and 27.3°C; overall = 20.8°C ± s.e. 3.9) and ambient (16.0, 20.0, 14.0, and 19.0°C; overall = 17.3°C ± s.e. 1.4; F2,6 = 16.35, P = 0.004, ηp2 = 0.845, Bonferroni difference only between grass-trees and 2 m away, P = 0.041 and grass-trees and ambient, P = 0.007; Figs 3 and 4). The largest mean temperature differences between 2 m away and grass-trees, and ambient and grass-trees, respectively, were: 19.8 and 8.9°C (Warren), 10.9 and 19.7°C (Scott Creek), 15.2 and 14.1°C (Deep Creek), and 19.0 and 13.7°C (Para Wirra). The largest mean difference between grass-tree canopy and ambient temperatures in summer was significant (mean = −14.1°C ± s.e. 2.2; t3 = −6.38, one-tailed P = 0.004), with a strong effect size of 0.965. The largest mean difference between temperatures under grass-tree and 2 m away was even more dramatic (mean = −16.2 ± s.e. 2.0; t3 = −7.96, one tailed P = 0.002; effect size = 0.975).
Mean temperatures under grass-trees (n = 12, except for Warren in winter (n = 10) and Scott Creek (n = 11)), at random positions 2 m away, and in the shade (ambient) for 24-h periods in winter and summer at Warren Conservation Park, Scott Creek Conservation Park, Deep Creek Conservation Park, and Para Wirra Recreation Park. Standard errors are shown only for Scott Creek in winter (see text).
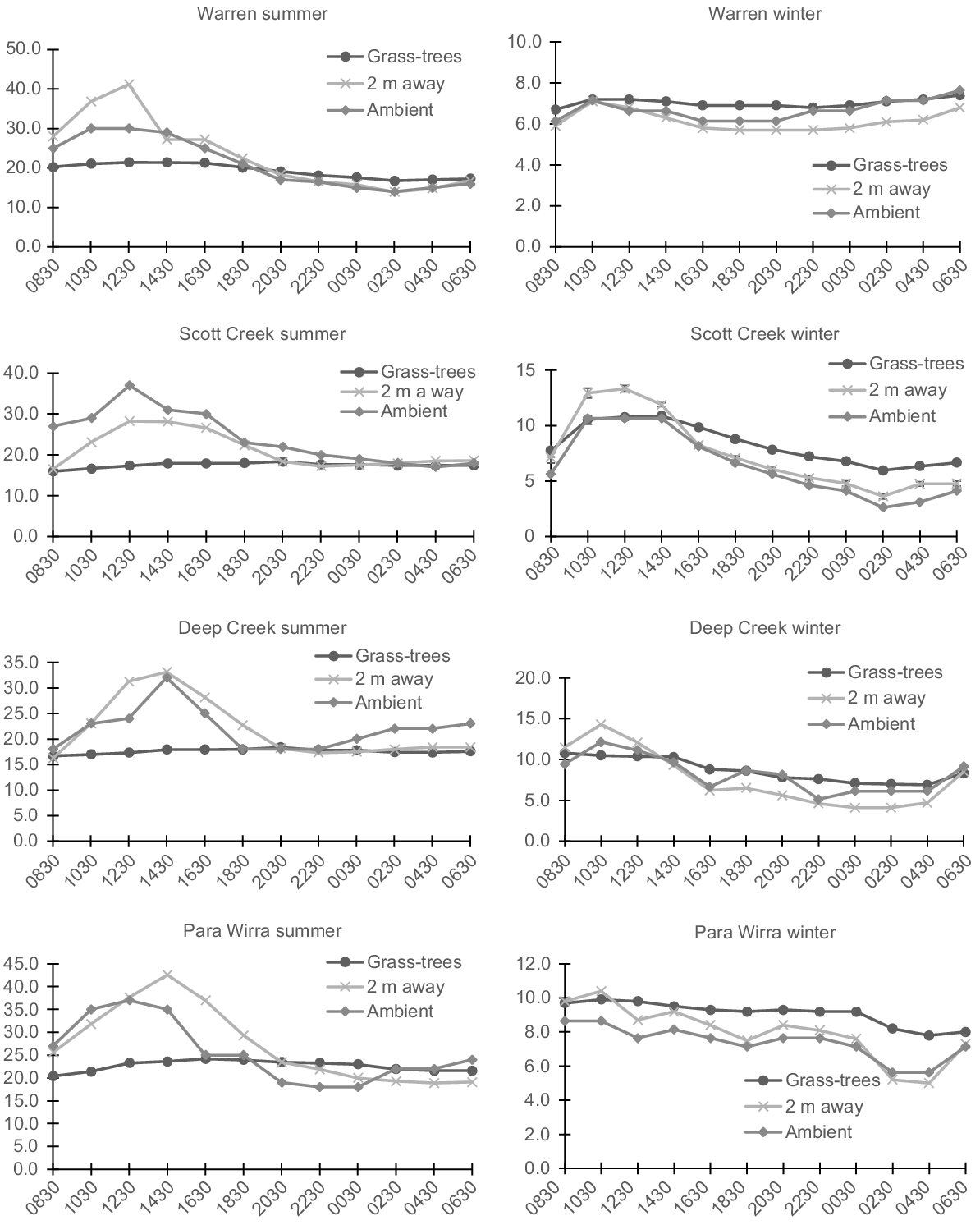
Mean temperature ranges at the four parks studied (Warren Conservation Park, Scott Creek Conservation Park, Deep Creek Conservation Park, and Para Wirra Recreation Park) under the canopy of grass-trees Xanthorrhoea semiplana ssp. semiplana, at random locations 2 m away, and in the shade (ambient) in (a) summer and (b) winter. Although the differences among locations are significant overall for each season, pairwise comparisons are not for winter (see text).
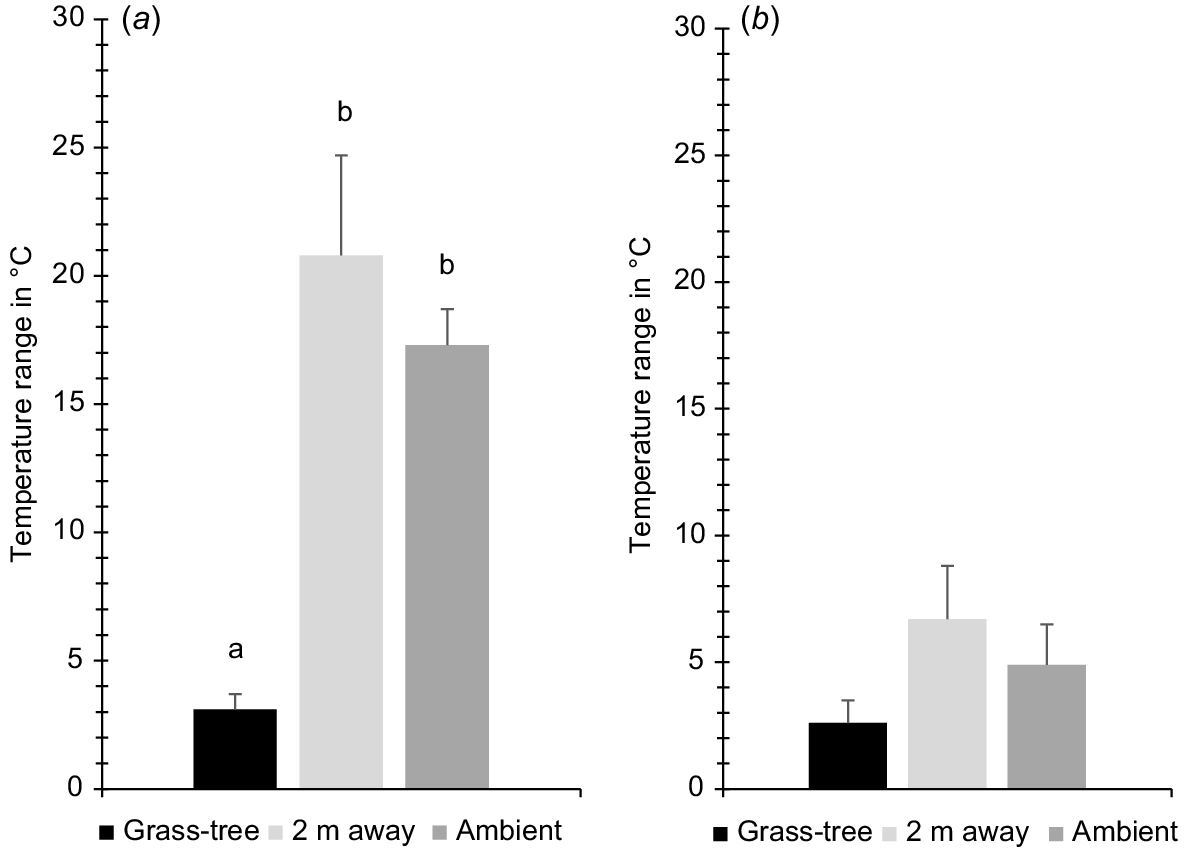
Winter temperatures over 24-h periods under grass-trees
In winter, grass-tree canopies also provided more stable temperatures than did other areas, but the differences were not as large as they were in summer. At Warren, Scott Creek, Deep Creek, and Para Wirra, respectively we measured mean temperature ranges under grass-trees of 0.7, 4.9, 3.9, and 2.1°C (overall = 2.9°C ± s.e. 0.9), compared to 1.4, 9.7, 10.2, and 5.4°C for 2 m away (overall = 6.7°C ± s.e. 2.1), and 1.5, 8.0, 7.0, and 3.0°C (overall = 4.9°C ± s.e. 1.6) for ambient (Figs 3 and 4). Although the difference among conditions is significant (F2,6 = 9.13, P = 0.015, ηp2 = 0.753), pairwise comparisons are not, possibly because of the conservativeness of the Bonferroni test and the small sample size. At these sites, the largest mean temperature differences between grass-trees and 2 m away were 1.2, 2.5, 3.0, and 3.0°C, and they were 0.8, 3.4, 2.5, and 2.6°C between grass-trees and ambient. Conditions were warmer under grass-trees during the night. The largest difference between mean temperature under grass-trees and ambient temperature was significant (mean = 2.3°C ± s.e. 0.5; t3 = 4.24, one-tailed P = 0.012; effect size = 0.926), as was that between temperature under grass-trees and temperature 2 m away (mean = 1.8°C ± s.e. 0.4; t3 = 4.35, one-tailed P = 0.011; effect size = 0.929).
Sampling of soil during and after heavy rainfall
Grass-tree canopy cover and soil dryness were strongly associated ( = 67.88, P < 0.001; Cramer’s V = 0.752), with z-scores indicating the significant superiority of canopy cover 2 (>90%) in keeping the soil dry, and the significantly better performance of canopy cover 1 over canopy cover 0 as well. During and after heavy rainfall, 80% of X. semiplana individuals with a canopy cover of 2 provided completely dry conditions under the trees, where the soil was dry to touch. In contrast, grass-trees that had a canopy cover of 0 provided no protection from rainfall and the soil was always saturated at the base of the tree. As for grass-trees with a canopy cover of 1, the majority (65%) had partially dry soil; the soil was driest close to the trunk and became more saturated at the edge of the canopy. Another 25% of these kept the soil completely dry, and 10% had saturated soil. Soils at the location 5 m away were predominantly saturated despite the presence of other understorey vegetation; the 3% recorded as partially dry were under large specimens of wire rapier-sedge (Lepidosperma semiteres) (Table 2).
Percentages of plants and locations 5 m away | |||||
---|---|---|---|---|---|
Canopy cover category | 5 m away | ||||
2 | 1 | 0 | |||
Soil saturated | 0 | 10 | 100 | 96 | |
Soil partially dry | 20 | 65 | 0 | 3 | |
Soil dry | 80 | 25 | 0 | 0 |
Discussion
Microclimate under grass-trees
This study demonstrated that the area under a thick X. semiplana ssp. semiplana canopy provides a remarkably stable temperatures in both winter and summer (Figs 3 and 4). In winter, grass-trees moderated cold extremes, with a smaller range of mean temperatures than found in other conditions, as determined also by Swinburn et al. (2007) for Xanthorrhoea preissii. The mean of the largest difference in temperature between grass-tree and ambient for all sites was 2.3°C, representing a significant difference, which may be consequential to determine animal activity. The difference was lower between grass-trees and 2 m away, since there was a chance that some of the 2-m away temperatures could have been taken under some protection from vegetation, but it still showed a slightly higher temperature under grass-trees (1.8°C on average). Torpor below and above minimal body temperature in animals is costly (Geiser and Broome 1993), and it is possible that the stability in temperature offered by grass-trees facilitates the maintenance of torpor in some species. Certainly, the protection of animals in torpor from predators would be enhanced by grass-trees. Species that do not use torpor could also benefit from the cover of thick skirts, the effect of which could be studied in the field with a warm animal body mimic.
The buffering role of grass-trees was strong in summer, with a maximum range for mean temperatures of 4.6°C (Warren), in comparison to random locations (27.3°C: Warren, Para Wirra) and ambient conditions in the shade (19.7°C: Scott Creek). The fact that the mean temperature at the hottest time of day for 12 grass-trees could be ~20°C lower under the grass-trees than in other shade points at their extraordinary value as shelters. For the times of largest mean differences between grass-tree and ambient temperatures for all sites, we found a mean of – 14.10°C, which demonstrates the likely contribution of grass-trees to the survival of several animal species. This difference calculated for 2 m away reached – 16.23°C, with mean potentially lethal temperatures at Warren and Para Wirra of 41.2 and 42.6°C, respectively, whereas mean temperatures under grass-trees at those sites were 21.4 and 23.6°C. Likewise, Keppel et al. (2017) found a significant impact of grass-trees in moderating high temperatures, compared to ground-below-canopy sensors. Our results cannot be compared directly to theirs because methods differed, and although they did not specify the subspecies of grass-tree, it appears from a figure that they were using Xanthorrhoea semiplana ssp. tateana. It would be interesting to compare the performance of the two subspecies, which vary in growth form, with X. semiplana ssp. tateana growing elongated stems as they age.
The Adelaide Hills and Mount Lofty Ranges are sometimes subjected to temperatures >40°C in summer. For example, Mount Crawford Bureau of Meteorology Station 023878, close to Para Wirra and Warren, the temperature reached 43.7°C in January 2019 and 42.0°C in December 2019 (Bureau of Meteorology 2022b). An increase above thermoneutral temperature can cause cognitive impairment (Soravia et al. 2021) and be lethal to ectotherms (e.g. Spellerberg 1972) and endotherms (e.g. Ikonomopoulou and Rose 2003). Maximum body temperatures in the few small marsupial species studied were in the 38–40°C range (McKechnie and Wolf 2019). Some Australian mammals avoid high ambient temperatures by sheltering in burrows during the heat of the day (Collins 1973; Walsberg 2000; Waudby and Petit 2017), but selecting appropriate shelters to escape hot weather extremes is particularly important for small mammals that do not normally burrow, such as Peramelidae (bandicoots and bilbies), Portoridae (potoroos and bettongs), and short-beaked echidnas (Tachyglossus aculeatus). We observed the entrance of vertebrate burrows hidden under the skirts of grass-trees. Echidnas radio-tracked at Warren Conservation Park sought shelter under grass-trees and in rabbit warrens (Feuerherdt 2002; L. Feuerherdt and S. Petit, unpubl. data). In Robinson’s (1954) experiments, the echidna was unable to thermoregulate at a temperature of 35°C and the southern long-nosed bandicoot (Perameles nasuta) could not endure prolonged periods at 40°C, although Brice et al. (2002) showed that in Western Queensland echidnas tolerated ambient temperatures of 35–40°C in the field and Barker et al. (2016) confirmed evaporative water loss above thermoneutrality in Tachyglossus aculeatus acanthion. The eastern barred bandicoot (Perameles gunnii) had difficulty thermoregulating above 30°C (Ikonomopoulou and Rose 2003). Seven out of eight bandicoot species have become extinct in South Australia, leaving the last one as the focus of great conservation concern. The Endangered southern brown bandicoot was observed to nest in a cluster of grass-trees at Scott Creek Conservation Park. It also sought shelter from the radio-tracker under grass-trees and foraged in a creek line with dense grass-trees (Frazer 2005). Although these observations took place in winter, they indicated that bandicoots used grass-tree habitat there, as they did in other studies (Paull 1993; Haby et al. 2013; Robinson et al. 2018).
We did not establish the impacts of grass-trees on humidity in summer, but X. preissii with skirts offered a higher and more stable relative humidity than did recently burnt ones in winter (Swinburn et al. 2007). Grass-trees may affect evaporative water loss as do burrows (e.g. Bulova 2002), and additional research would need to establish how grass-trees may minimise this loss in summer for different local species. Keppel et al. (2017) showed that at a time when relative humidity was <10% away from a grass-tree on the Fleurieu Peninsula in summer, it remained above 40% under the grass-tree, although Walsberg (2000) indicated that absolute humidity is a better index, because it sets the gradient for evaporative cooling. The risk of desiccation is associated with spatiotemporal patterns of air humidity (Rozen-Rechels et al. 2019), and humidity should be considered in future studies that examine the role of shelter plants. Air moisture under grass-trees may be critical for some animals to survive very high temperatures, since it reduces water loss in animals, which normally benefit from humidity inside burrows (Schmidt-Nielsen and Schmidt-Nielsen 1950).
Protection from rainfall
A crucial role of grass-trees in winter comes from the extraordinary capacity of the large specimens to keep the soil conditions completely dry in torrential rainfall, and undoubtedly protect animals from the wind as well. The largest, and presumably oldest, grass-trees with canopy covers of 2 provided completely dry shelter in 80% of cases, and performed significantly better than did the other categories of 0 and 1. Experiments conducted on cold and wet sheep showed a dramatic increase in plasma cortisol concentration before the final phase of hypothermia, accompanied by cardiac insufficiency (Panaretto and Vickery 1970, 1971). Such experiments have been mostly conducted on livestock, and we assume that small wildlife will seek shelter during downpours, considering potential impact of wetting on thermal protection (Webb and King 1984). The disappearance of grass-trees could limit dramatically the protection from wetness normally afforded to small ground-dwelling animals.
Grass-tree conservation in view of their role as shelters
Many vertebrates and invertebrates will use soil cracks, burrows, and other structures such as caves and rock crevices to avoid lethal temperatures or heavy rains. However, few plants other than those bearing hollows (e.g. Gibbons et al. 2002), some sedges (e.g. Happold 1976), and other dome-shaped plants (e.g. Triodia spp., Bell et al. 2021) can perform the same functions as those of grass-trees, and deliver with their foliage the remarkable stability of temperatures and waterproofing quality demonstrated for X. semiplana ssp. semiplana. Grass-trees with trunks and dense skirts also offer the benefit of a more stable microclimate than may be found away from the canopy (Swinburn et al. 2007; Keppel et al. 2017).
The availability of thermally appropriate microhabitats combined with concealment is potentially a major limiting factor affecting animal survival in degraded ecosystems, such as the Hills and Fleurieu region of Adelaide, where 41% of mammal species have already become extinct (Landscapes South Australia Hills and Fleurieu 2022). Considering the extremely high value of grass-trees as shelters for wildlife, it is likely that their availability may also affect foraging times of animals, with implications for predation.
The slow growth rate of grass-trees (e.g. Lamont et al. 2004 cited stem growth rates from 5 mm to 50 mm per year depending on species and condition) and the increasing value of the shelter they provide as they age make them particularly important to conserve. Although the resilience of some grass-tree species to bushfires is well known (e.g. Lamont et al. 2000), it may be overestimated by some. Starch loss may reduce resprouting success after fires depending on season (Korczynskyj and Lamont 2005). Tozer and Keith (2012) demonstrated that a single fire interval of 17 years resulted in the local extinction of Xanthorrhoea resinosa. Curtis (1998) showed that prescribed burning, which is now widely undertaken in South Australia, had a long-term deleterious impact on large X. australis. It also reduces tree hollow availability (Flanagan-Moodie et al. 2018) and could compound habitat loss. Southern brown bandicoots, vulnerable to predation after fires, established nests in dense eucalypt regrowth in New South Wales, when Xanthorrhoea spp. had been burnt and were unavailable (MacGregor et al. 2023); although the comparison of grass-tree and eucalypt regrowth canopy was not made, the latter is more open and likely to be inferior to that of grass-trees. The re-establishment of large skirts of dry leaves is a very slow process for Xanthorrhoea species. Skirt burning of X. semiplana ssp. tateana is undertaken by some landholders on Kangaroo Island because they fear that the grass-trees will carry bushfires. Although some invertebrates may persist even in burning grass-trees (Brennan et al. 2011), this practice is likely to have a severe impact on local animal diversity, and we suggest examining how different fire intensities modify the skirts of grass-trees. Swinburn et al. (2007) demonstrated the loss of microclimate-stabilising properties associated with the burning of grass-tree skirts.
Long-term negative impacts of Phytophthora cinnamomi, a soil fungal pathogen to which grass-trees are particularly sensitive, can be dramatic for both plant and animal communities (Wilson et al. 2020). It has resulted in significant declines in grass-trees and associated biodiversity, with little evidence of recovery post infestation (Aberton et al. 2001; Laidlaw and Wilson 2003; Lamont et al. 2004; Casey 2022). Our recent observations of the spread of the pathogen on Kangaroo Island after the 2019–2020 bushfires are alarming. Soil compaction and its negative impacts on X. preissii (Ward et al. 2011) also indicate that special care must be taken in silviculture environments.
We recommend evaluating the relevance of grass-tree microclimate, including humidity, to specific animal taxa. The effect of grass-tree skirt availability on foraging times of animals should be examined. We also recommend the study of population dynamics of X. semiplana to facilitate its conservation, particularly in relation to the highly detrimental impacts of plant losses due to P. cinammomi, and those of fire on the temporal availability of protective foliage.
Acknowledgements
We thank B. Wilson, R. Sharrad, F. Tiver, and J. Gibbs for comments on an earlier version of the manuscript, S. and G. Frazer for field assistance, R. Aebi for help with logistics, J. Weber, and Department for Environment and Heritage (DEH) now Department for Environment and Water staff P. Canty, E. Dahl, M. Trebilcock, and S. Taylor. The research was conducted under DEH permit G24919 and animal ethics permit 74/04.
References
Aberton MJ, Wilson BA, Hill J, Cahill DM (2001) Development of disease caused by Phytophthora cinnamomi in mature Xanthorrhoea australis. Australian Journal of Botany 49, 209-219.
| Crossref | Google Scholar |
Barker JM, Cooper CE, Withers PC, Nicol SC (2016) Reexamining echidna physiology: the big picture for Tachyglossus aculeatus acanthion. Physiological and Biochemical Zoology 89, 169-181 PMID:.
| Crossref | Google Scholar | PubMed |
Bell KJ, Doherty TS, Driscoll DA (2021) Predators, prey or temperature? Mechanisms driving niche use of a foundation plant species by specialist lizards. Proceedings of the Royal Society B: Biological Sciences 288, 20202633.
| Crossref | Google Scholar |
Bickford S, Gell P, Jancock GJ (2008) Wetland and terrestrial vegetation change since European settlement on the Fleurieu Peninsula, South Australia. The Holocene 18, 425-436.
| Crossref | Google Scholar |
Bleicher SS, Dickman CR (2020) On the landscape of fear: shelters affect foraging by dunnarts (Marsupialia, Sminthopsis spp.) in a sandridge desert environment. Journal of Mammalogy 101, 281-290.
| Crossref | Google Scholar |
Bos DG, Carthew SM (2003) The influence of behaviour and season on habitat selection by a small mammal. Ecography 26, 810-820.
| Crossref | Google Scholar |
Brennan KEC, Moir ML, Wittkuhn RS (2011) Fire refugia: the mechanism governing animal survivorship within a highly flammable plant. Austral Ecology 36, 131-141.
| Crossref | Google Scholar |
Brice PH, Grigg GC, Beard LA, Donovan JA (2002) Heat tolerance of short-beaked echidnas (Tachyglossus aculeatus) in the field. Journal of Thermal Biology 27, 449-457.
| Crossref | Google Scholar |
Bulova S (2002) How temperature, humidity, and burrow selection affect evaporative water loss in desert tortoises. Journal of Thermal Biology 27, 175-189.
| Crossref | Google Scholar |
Bureau of Meteorology (2005) South Australian climatic averages. Available at http://www.bom.gov.au/climate/averages/tables/ca_sa_names.shtml [accessed 20 April 2005]
Bureau of Meteorology (2022a) Climate data online. Available at http://www.bom.gov.au/climate/data/index.shtml?bookmark=200 [accessed 4 January 2022]
Bureau of Meteorology (2022b) Climate statistics for Australian locations – Mount Crawford AWS. Available at http://www.bom.gov.au/climate/averages/tables/cw_023878_All.shtml [accessed 20 November 2022]
Bureau of Meteorology (2022c) Monthly rainfall – Longwood Alert. Available at http://www.bom.gov.au/jsp/ncc/cdio/weatherData/av?p_nccObsCode=139&p_display_type=dataFile&p_startYear=&p_c=&p_stn_num=023108 [accessed 20 November 2022]
Cahill DM, Rookes JE, Wilson BA, Gibson L, McDougall KL (2008) Phytophthora cinnamomi and Australia’s biodiversity: impacts, predictions and progress towards control. Australian Journal of Botany 56, 279-310.
| Crossref | Google Scholar |
Collins BG (1973) The ecological significance of thermoregulatory responses to heat stress shown by two populations of an Australian murid, Rattus fuscipes. Comparative Biochemistry and Physiology Part A: Physiology 44, 1129-1140.
| Crossref | Google Scholar |
Commonwealth of Australia (2018) ‘Threat abatement plan for disease in natural ecosystems caused by Phytophthora cinnamomi.’ (Department of the Environment and Energy: Australia). Available at https://www.dcceew.gov.au/sites/default/files/documents/tap-phytophthora-cinnamomi-2018.pdf [accessed 21 November 2022]
Curtis NP (1998) A post-fire ecological study of Xanthorrhoea australis following prescribed burning in the Warby Range State Park, North-eastern Victoria, Australia. Australian Journal of Botany 46, 253-272.
| Crossref | Google Scholar |
de Winter JCF (2013) Using the Student’s t-test with extremely small sample sizes. Practical Assessment, Research, and Evaluation 18, 10.
| Crossref | Google Scholar |
Di Stefano J, Owen L, Morris R, Duff T, York A (2011) Fire, landscape change and models of small mammal habitat suitability at multiple spatial scales. Austral Ecology 36, 638-649.
| Crossref | Google Scholar |
Feuerherdt L (2002) The role of echidnas (Tachyglossus aculeatus) in dispersing mycorrhizal fungi associated with the endangered pink-lipped spider orchid (Caladenia behrii) north of Adelaide and distribution of these fungi at Warren Conservation Park, South Australia. Honours thesis, University of South Australia, Adelaide.
Flanagan-Moodie AK, Holland GJ, Clarke MF, Bennett AF (2018) Prescribed burning reduces the abundance of den sites for a hollow-using mammal in a dry forest ecosystem. Forest Ecology and Management 429, 233-243.
| Crossref | Google Scholar |
Frazer DS, Petit S (2007) Use of Xanthorrhoea semiplana (grass-trees) for refuge by Rattus fuscipes (southern bush rat). Wildlife Research 34, 379-386.
| Crossref | Google Scholar |
Garden JG, McAlpine CA, Possingham HP, Jones DN (2007) Habitat structure is more important than vegetation composition for local-level management of native terrestrial reptile and small mammal species living in urban remnants: a case study from Brisbane, Australia. Austral Ecology 32, 669-685.
| Crossref | Google Scholar |
Gibbons P, Lindenmayer DB, Barry SC, Tanton MT (2002) Hollow selection by vertebrate fauna in forests of southeastern Australia and implications for forest management. Biological Conservation 103, 1-12.
| Crossref | Google Scholar |
Geiser F, Broome LS (1993) The effect of temperature on the pattern of torpor in a marsupial hibernator. Journal of Comparative Physiology B 163, 133-137.
| Crossref | Google Scholar |
Gill AM, Ingwersen F (1976) Growth of Xanthorrhoea australis R. Br. in relation to fire. Journal of Applied Ecology 13, 195-203.
| Crossref | Google Scholar |
Glatz RV, Leijs R, Hogendoorn K (2015) Biology, distribution and conservation of green carpenter bee (Xylocopa aeratus: Apidae) on Kangaroo Island, South Australia. Technical Report. Available at http://www.dess.net.au/Glatz%20et%20al%202015%20Xylocopa%20aeratus%20report.pdf
Haby NA, Conran JG, Carthew SM (2013) Microhabitat and vegetation structure preference: an example using southern brown bandicoots (Isoodon obesulus obesulus). Journal of Mammalogy 94, 801-812.
| Crossref | Google Scholar |
Happold M (1976) Social behaviour of the conilurine rodents (Muridae) of Australia. Zeitschrift für Tierpsychologie 40, 113-182 PMID:.
| Crossref | Google Scholar | PubMed |
Huey RB (1991) Physiological consequences of habitat selection. The American Naturalist 137, S91-S115.
| Crossref | Google Scholar |
Ikonomopoulou MP, Rose RW (2003) The metabolic rate and thermal conductance of the eastern barred bandicoot (Perameles gunnii) at different ambient temperatures. Australian Journal of Zoology 51, 603-610.
| Crossref | Google Scholar |
Keppel G, Anderson S, Williams C, Kleindorfer S, O’Connell C (2017) Microhabitats and canopy cover moderate high summer temperatures in a fragmented Mediterranean landscape. PLoS ONE 12, e0183106 PMID:.
| Crossref | Google Scholar | PubMed |
Korczynskyj D, Lamont BB (2005) Grasstree (Xanthorrhoea preissii) recovery after fire in two seasons and habitats. Australian Journal of Botany 53, 509-515.
| Crossref | Google Scholar |
Laidlaw WS, Wilson BA (2003) Floristic and structural characteristics of a coastal heathland exhibiting symptoms of Phytophthora cinnamomi infestation in the eastern Otway Ranges, Victoria. Australian Journal of Botany 51, 283-293.
| Crossref | Google Scholar |
Laidlaw WS, Wilson BA (2006) Habitat utilisation by small mammals in a coastal heathland exhibiting symptoms of Phytophthora cinnamomi infestation. Wildlife Research 33, 639-649.
| Crossref | Google Scholar |
Lamont BB, Downes S (1979) The longevity, flowering and fire history of the grasstrees Xanthorrhoea pressii and Kingia australis. Journal of Applied Ecology 16, 93-99.
| Crossref | Google Scholar |
Lamont BB, Swanborough PW, Ward D (2000) Plant size and season of burn affect flowering and fruiting of the grasstree Xanthorrhoea preissii. Austral Ecology 25, 268-272.
| Crossref | Google Scholar |
Lamont BB, Wittkulan R, Korczynskyj D (2004) Ecology and ecophysiology of grasstrees. Australian Journal of Botany 52, 561-582.
| Crossref | Google Scholar |
Landscapes South Australia Hills and Fleurieu (2022) Mammals. Available at https://www.landscape.sa.gov.au/hf/our-priorities/nature/native-plants-animals-and-biodiversity/native-plants-and-animals/native-animals/mammals [accessed 20 November 2022]
Lazenby BT, Pye T, Richardson A, Bryant SL (2008) Towards a habitat model for the New Holland mouse Pseudomys novaehollandiae in Tasmania? population vegetation associations and an investigation into individual habitat use. Australian Mammalogy 29, 137-148.
| Crossref | Google Scholar |
Leys R (2000) A revision of the Australian carpenter bees, genus Xylocopa Latreille, subgenera Koptortosoma Gribodo and Lestis Lepeletier & Serville (Hymenoptera: Apidae). Invertebrate Systematics 14, 115-136.
| Crossref | Google Scholar |
MacGregor CI, Robinson NM, Blanchard W, Lindenmayer DB (2023) Selection, characteristics, and frequency of use of shelter sites by the Southern Brown Bandicoot Isoodon obesulus obesulus and the Southern Long-nosed Bandicoot Perameles nasuta in a post-fire landscape. Australian Zoologist
| Crossref | Google Scholar |
Marchesan D, Carthew SM (2004) Autecology of the yellow-footed antechinus (Antechinus flavipes) in a fragmented landscape in southern Australia. Wildlife Research 31, 273-282.
| Crossref | Google Scholar |
Mason ED, Firn J, Hines HB, Baker AM (2017) Plant diversity and structure describe the presence of a new, threatened Australian marsupial within its highly restricted, post-fire habitat. PLoS ONE 12, e0182319 PMID:.
| Crossref | Google Scholar | PubMed |
McKechnie AE, Wolf BO (2019) The physiology of heat tolerance in small endotherms. Physiology 34, 302-313.
| Crossref | Google Scholar |
Panaretto BA, Vickery MR (1970) The rates of plasma cortisol entry and clearance in sheep before and during their exposure to a cold, wet environment. Journal of Endocrinology 47, 273-285.
| Crossref | Google Scholar |
Panaretto BA, Vickery MR (1971) Cardiovascular parameters in shorn sheep prior to and during their exposure to a cold, wet environment. Experimental Physiology 56, 101-107.
| Crossref | Google Scholar |
Petit S, Dickson CR (2005) Grass-tree (Xanthorrhoea semiplana, Liliaceae) facilitation of the endangered pink-lipped spider orchid (Caladenia syn. Arachnorchis behrii, Orchidaceae) varies in South Australia. Australian Journal of Botany 53, 455-464.
| Crossref | Google Scholar |
Robinson KW (1954) Heat tolerances of Australian monotremes and marsupials. Australian Journal of Biological Sciences 7, 348-360.
| Crossref | Google Scholar |
Robinson NM, MacGregor CI, Hradsky BA, Dexter N, Lindenmayer DB (2018) Bandicoots return to Booderee: initial survival, dispersal, home range and habitat preferences of reintroduced southern brown bandicoots (eastern sub species; Isoodon obesulus obesulus). Wildlife Research 45, 132-142.
| Crossref | Google Scholar |
Rozen-Rechels D, Dupoué A, Lourdais O, Chamaillé-Jammes S, Meylan S, Clobert J, Le Galliard J-F (2019) When water interacts with temperature: ecological and evolutionary implications of thermo-hydroregulation in terrestrial ectotherms. Ecology and Evolution 9, 10029-10043 PMID:.
| Crossref | Google Scholar | PubMed |
Schmidt-Nielsen B, Schmidt-Nielsen K (1950) Evaporative water loss in desert rodents in their natural habitat. Ecology 31, 75-85.
| Crossref | Google Scholar |
Smith KR, Thiboutot DM (2008) Thematic review series: Skin Lipids. Sebaceous gland lipids: friend or foe? Journal of Lipid Research 49, 271-281 PMID:.
| Crossref | Google Scholar | PubMed |
Smith KJ, Fleming PA, Kreplins TL, Wilson BA (2019) Population monitoring and habitat utilisation of the ash-grey mouse (Pseudomys albocinereus) in Western Australia. Australian Mammalogy 41, 170-178.
| Crossref | Google Scholar |
Soravia C, Ashton BJ, Thornton A, Ridley AR (2021) The impacts of heat stress on animal cognition: implications for adaptation to a changing climate. WIREs Climate Change 12, e713.
| Crossref | Google Scholar |
Spellerberg IF (1972) Temperature tolerances of Southeast Australian reptiles examined in relation to reptile thermoregulatory behaviour and distribution. Oecologia 9, 23-46.
| Crossref | Google Scholar |
Spencer R-J, Cavanough VC, Baxter GS, Kennedy MS (2005) Adult free zones in small mammal populations: response of Australian native rodents to reduced cover. Austral Ecology 30, 868-876.
| Crossref | Google Scholar |
Steen Z, Schwarz MP (2000) Nesting and life cycle of the Australian green carpenter bees Xylocopa (Lestis) aeratus Smith and Xylocopa (Lestis) bombylans (Fabricius) (Hymenoptera: Apidae: Xylocopinae). Australian Journal of Entomology 39, 291-300.
| Crossref | Google Scholar |
Sunday JM, Bates AE, Kearney MR, Colwell RK, Dulvy NK, Longino JT, Huey RB (2014) Thermal-safety margins and the necessity of thermoregulatory behavior across latitude and elevation. Proceedings of the National Academy of Sciences 111, 5610-5615.
| Crossref | Google Scholar |
Swinburn ML, Fleming PA, Craig MD, Grigg AH, Garkaklis MJ, Hobbs RJ, Hardy GEStJ (2007) The importance of grasstrees (Xanthorrhoea preissii) as habitat for mardo (Antechinus flavipes leucogaster) during post-fire recovery. Wildlife Research 34, 640-651.
| Crossref | Google Scholar |
Tozer MG, Keith DA (2012) Population dynamics of Xanthorrhoea resinosa Pers. over two decades: implications for fire management. Proceedings of the Linnean Society of New South Wales 134, B249-B266.
| Google Scholar |
Walsberg GE (2000) Small mammals in hot deserts: some generalizations revisited. BioScience 50, 109–120. 10.1641/0006-3568(2000)050[0109:SMIHDS]2.3.CO;2
Wanniarachchi S, Swan M, Nevil P, York A (2022) Using eDNA metabarcoding to understand the effect of fire on the diet of small mammals in a woodland ecosystem. Ecology and Evolution 12, e9457 PMID:.
| Crossref | Google Scholar | PubMed |
Ward B, Robinson RM, Cranfield RJ, Williams MR (2011) Forestcheck: the response of vascular flora to silviculture in jarrah (Eucalyptus marginata) forest. Australian Forestry 74, 276-287.
| Crossref | Google Scholar |
Waudby HP, Petit S (2017) Thermoregulatory value of cracking-clay soil shelters for small vertebrates during extreme desert conditions. Integrative Zoology 12, 237-249 PMID:.
| Crossref | Google Scholar | PubMed |
Webb DR, King JR (1984) Effects of wetting of insulation of bird and mammal coats. Journal of Thermal Biology 9, 189-191.
| Crossref | Google Scholar |
Wilkinson DA, Gordon CG, Beard LA (1998) Shelter selection and home range of echidnas, Tachyglossus aculeatus, in the highlands of south-east Queensland. Wildlife Research 25, 219-232.
| Crossref | Google Scholar |
Wilson BA, Annett K, Laidlaw WS, Cahill DM, Garkaklis MJ, Zhuang-Griffin L (2020) Long term impacts of Phytophthora cinnamomi infestation on heathy woodland in the Great Otway National Park in south-eastern Australia. Australian Journal of Botany 68, 542-556.
| Crossref | Google Scholar |
World Flora Online (2022) Xanthorrhoea Sm. Available at http://www.worldfloraonline.org/taxon/wfo-4000040894 [accessed 12 November 2022]