In vitro effects of Type I interferons (IFNτ and IFNα) on bovine hepatocytes cultured with or without Kupffer cells
Kai Josef Endriß A , Marie Margarete Meyerholz B , Teresa Fischbach A , Lutz Brimmers A , Christiane Pfarrer C , Christina Deborah Marth D and Marion Schmicke
A University of Veterinary Medicine Hanover, Clinic for Cattle, Endocrinology, Bischofsholer Damm 15, 30539 Hanover, Germany.
B Clinic for Ruminants with Ambulatory and Herd Health Services, Centre for Clinical Veterinary Medicine, Ludwig-Maximilians-University Munich, Sonnenstraße 16, 85764 Oberschleißheim, Germany.
C University of Veterinary Medicine Hanover, Anatomy, Bischofsholer Damm 15, 30539 Hanover, Germany.
D Melbourne Veterinary School, The University of Melbourne, 250 Princes Highway, Werribee, Vic. 3030, Australia.
E Martin-Luther University Halle-Wittenberg, Faculty of Natural Sciences III, Institute of Agricultural and Nutritional Sciences, Animal Health Management, Theodor-Lieser-Straße 11, 06120 Halle, Germany.
F Corresponding author. Email: marion.schmicke@landw.uni-halle.de
Reproduction, Fertility and Development 33(4) 305-317 https://doi.org/10.1071/RD20278
Submitted: 16 October 2020 Accepted: 17 December 2020 Published: 12 February 2021
Journal Compilation © CSIRO 2021 Open Access CC BY-NC-ND
Abstract
In cattle, maternal recognition of early pregnancy depends on the effects of the embryonic signal interferon (IFN)-τ. IFN-stimulated genes have been upregulated in the maternal liver during early pregnancy. In this study, primary hepatocyte cell culture models were evaluated for their suitability to test Type I IFN effects in vitro. The expression of target genes (interferon-stimulated gene 15 (ISG-15), interferon-induced GTP-binding protein (MX-1), C-X-C motif chemokine 10 (CXCL-10), CXCL-5, insulin-like growth factor 1 (IGF-1), IGF binding protein 2 (IGFBP-2)) was measured using reverse transcription–quantitative polymerase chain reaction in hepatocytes from monoculture or in indirect coculture with Kupffer cells (HKCid) on Days 1, 2, 3 and 4 of culture (n = 21 donor cows). Gene expression was also measured on Day 4 after challenging the cultures with recombinant IFNτ, IFNα, progesterone (P4), IFNτ + IFNα or IFNτ + P4 for 6 h. A significant increase in the mRNA expression of target genes in hepatocytes was shown in response to stimulation with IFNτ. The Kupffer cells in coculture did not influence the effects of IFNτ in hepatocytes. In conclusion, primary bovine hepatocyte cultures are suitable for stimulation experiments with Type I IFNs and as an extrauterine model for embryo–maternal communication. The proposed endocrine action of IFNτ in the liver may affect maternal metabolism and immune function in the liver.
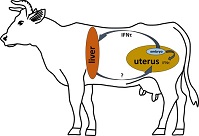
Keywords: cattle, cell culture, embryo, embryo-maternal communication, endocrinology, gestation, hepatocytes, IGF binding protein, insulin-like growth factor, interferone tau
Introduction
In sheep and cattle, there is evidence that interferon (IFN)-τ reaches the blood stream and induces expression of IFN-stimulated genes (ISG) in the endometrium and other organs. In 2008, an ‘antiviral activity’ was detected in the uterine vein in sheep at Day 15 of pregnancy (Oliveira et al. 2008). Two years later this ‘antiviral activity’ was confirmed to be IFNτ, and ISG expression was detected in ovarian tissue (Bott et al. 2010). Recently, significantly higher gene expression of ISG-15 and MX dynamin like GTPase 1 (MX1) and MX dynamin like GTPase 2 (MX2) was noted in cervical and vaginal tissues on Days 17–18 after AI in cows (Kunii et al. 2018). Increased expression of ISG genes has also been detected in leucocytes between Days 15 and 32 after insemination in both sheep (Yankey et al. 2001) and cattle (Han et al. 2006). This can be assumed to be in response to IFNτ. Similarly, several studies indicated a difference in ISG-15 expression in the liver between pregnant and non-pregnant sheep on Days 14 (Romero et al. 2015) and 15 (Bott et al. 2010). In cows, studies in Holstein Friesian and Angus heifers detected increased mRNA levels of ISG (MX1, MX2, ISG-15 and oligoadenulate synthetase 1(OAS-1)) in the liver on Day 18 of pregnancy compared with non-pregnant heifers (Meyerholz et al. 2015; Ruhmann et al. 2017). Ruhmann et al. (2017) also demonstrated OAS-1 protein expression in hepatocytes via immunohistochemistry. However, there is still no direct proof (e.g. immunoassays) of IFNτ in the blood circulation. Nakamura et al. (2016) detected IFNτ in extracellular vesicles and discussed its involvement in the regulation of ISGs.
MX1, MX2, ISG-15 and OAS-1 are classical ISGs. They are induced in response to IFNτ after binding to Type 1 IFN receptors (interferon-α/β receptor (IFNAR1/2)) via induction of the Janus tyrosine kinase (JAK) signal transducers and activators of transcription (STAT) pathway. In addition, Type I IFNs stimulate increased expression of other genes via non-classical signalling pathways. Arosh et al. (2004) showed that IFNτ reduced the expression of prostaglandin F synthases on Day 16 of pregnancy in cows. In contrast, increased expression of the non-classical ISG chemokine (C-X-C motif) ligand 6 (CXCL-6) in response to IFNτ was shown in endometrial cells (Teixeira et al.1997).
The present study focused on two chemokines that are traditionally produced during an inflammatory reaction. The chemokine CXCL-10 is produced in Kupffer cells (KCs) and induces decreased angiogenesis, whereas CXCL-5 supports angiogenesis and activates neutrophil granulocytes (Fernandez and Lolis 2002; Dembic 2015). Metabolic genes also seem to be affected by IFNτ. For example, there were changes in the expression of insulin like growth factor 1 (IGF-1) and IGF binding protein 2 (IGFBP-2) mRNA expression in the endometrium (Robinson et al. 2008) and peripheral blood (Meyerholz et al. 2015) during early pregnancy in cows. An effect of IFNτ on the somatotropic axis can therefore be speculated, because IGFBP-2 is mainly produced in the liver. However, it is still unclear whether non-classical ISG expression is induced by IFNτ in bovine hepatocytes and whether it is affected by local liver KCs or progesterone (P4). KCs are located in the endothelium of liver sinusoids and displace the largest population of tissue macrophages. KCs play an important role in host defence and stay in close paracrine contact to hepatocytes (Naito et al. 2004). It remains unknown whether all IFNτ effects are specific or whether other Type I IFNs can induce comparable expression of ISGs. IFNτ belongs to the group of Type I IFNs (IFNα, IFNβ, IFNϵ, IFNκ and IFNω; Roberts et al. 1998; Capobianchi et al. 2015) and shows 45–55% homology to IFNs of the IFNα group and 70.3% homology to bovine IFNα (Imakawa et al. 1987). All Type I IFNs bind to the same receptor (IFNAR1/2), but stimulate the expression of different ISGs downstream (Mogensen et al. 1999; Uzé et al. 2007).
In this study, a well-established bovine liver cell culture (Ehrhardt and Schmicke 2016; Witte et al. 2019) was used and a KC coculture was established to test the endocrine effects of IFNτ. The following questions were investigated: (1) is the liver cell culture suitable for testing the effects of Type I IFNs; (2) does the Type I IFN effect differ between pure hepatocytes and hepatocytes cocultured with KCs; (3) does the expression of ISGs differ between hepatocytes stimulated with IFNτ and other Type I IFNs; and (4) does simultaneous stimulation with P4 and IFNτ affect the expression of single target genes in the maternal liver?
Materials and methods
Extraction of hepatocytes and KCs
Between March 2017 and July 2018, 15 bovine livers (six obtained at an abattoir, nine that had been ‘patients’ of the Clinic for Cattle at the University of Veterinary Medicine Hannover; see below) were used to establish a hepatocyte coculture with KCs without dexamethasone. A further six livers from female pluriparous Holstein Friesian cows (also clinic ‘patients’) were used to investigate the effects of type I IFNs on bovine hepatocytes as a model for the metabolic effects of early pregnancy signals in ruminants.
All cows used in this study that had been patients of the Clinic for Cattle at the University of Veterinary Medicine Hannover were killed for medical reasons, not including any signs of metabolic disease and/or general inflammation with fever. Within 20 min (abattoir) or 5 min (clinic ‘patients’) of death, the caudate process of the caudate lobe of the liver was removed. It was then rinsed with 200 mL ice-cold EGTA buffer, followed by 200 mL EGTA-free perfusion buffer. Hepatocytes were isolated using a two-step collagenase perfusion as described previously (Witte et al. 2019), with one modification: in order to test the effects of Type I IFNs without bias, all buffers were used without addition of dexamethasone.
KC were also extracted from the livers using the cell suspension that was obtained after collagenase perfusion. In addition to hepatocytes, this suspension contained all non-parenchymal cells of the liver. This total cell suspension was centrifuged at 300g for 5 min at 4°C. The pellet was resuspended in William’s E medium (PAN-Biotech) and 10% fetal bovine serum (FBS; PAN-Biotech). According to the protocol of Smedsrød et al. (1985), the KCs were further purified by two-density gradient centrifugation (gradient: 5 mL of 50% Percoll (Sigma-Aldrich), 5 mL of 25% Percoll and 5 mL resuspended pellet). After centrifugation (1250g, 8 min, 4°C), the fraction between the two layers of Percoll contained KCs and fibroblasts. Further purification was based on selective adhesion properties between the two cell types on plastic surfaces (Smedsrød et al. 1985). After 20 min adhesion time at 37°C under 95% O2 and 5% CO2, cells were washed twice with phosphate-buffered saline (PBS) and harvested by the addition of 1 mL of 10% trypsin (Sigma-Aldrich) to the cells. Williams E Medium (PAN-Biotech) with 10% FBS (PAN-Biotech) was used to stop the enzymatic reaction, and the cells were centrifuged at 1300g for 1 min at 4°C. The cell pellet was resuspended in 1 mL Williams E Medium (PAN-Biotech) and cell vitality was tested by Trypan blue exclusion. The number of KCs was counted under a microscope (Fig. 1).
Establishment of hepatocyte–KC cocultures
In order to find the most suitable model, two different coculture methods were tested and compared with a hepatocyte monoculture (HMC) as a control. Hepatocytes were cocultured with KCs at a ratio of 6:1 (0.5 million hepatocytes:0.15 million KCs per well) in direct (HKCd) or indirect contact (HKCid). The three different models are shown in Fig. 2.
![]() |
Basal expression of genes of interest
Six livers were used to test whether the basal expression of genes of interest is comparable between hepatocytes extracted from different donors and on different days. To this end, hepatocytes were immediately frozen at –80°C after Percoll density centrifugation. Cell samples were also taken from HMC and HKCid on Days 1, 2, 3 and 4 of culture. The mRNA expression of MX1, ISG-15, CXCL-10, CXCL-5, IGFBP-2, IGF-1 and albumin was analysed by reverse transcription–quantitative polymerase chain reaction (RT-qPCR) and compared between the six donors. Concentrations of lactate dehydrogenase (LDH) and urea in the culture supernatant were measured as indicators of vitality.
Stimulation of hepatocytes and KC coculture with IFNτ, IFNα and P4
HMC and HKCid were established from five consecutive livers and cultured for 4 days. Each stimulation condition was evaluated in 12 wells. Two wells were pooled prior to analysis. The culture medium was changed daily. On Day 4, cells were stimulated for 6 h with 0.06 or 0.6 ng well–1 IFNτ or IFNα. Other cells were stimulated simultaneously with 0.6 ng well–1 IFNτ and 0.6 ng well–1 IFNα or 0.06 ng well–1 IFNτ and 0.06 ng well–1 IFNα. Cells were also stimulated with 0.6 ng well–1 IFNτ with or without 5 or 10 ng well–1 P4 progesterone and were compared with cells stimulated with 5 and 10 ng well–1 P4 alone. Cells and supernatants were stored at –80°C until further processing.
Live/dead staining
To evaluate the vitality of the hepatocytes, cells were stained using a LIVE/DEAD Viability/Cytotoxicity Kit (ThermoFisher Scientific) according to the manufacturer’s instructions and evaluated under an inverted fluorescence microscope with phase contrast (Axiovert 200M) using AxioVision 4.8.2.0 software. The proportion of live and dead cells was determined by counting 100 hepatocytes in different visual fields (various fields were used until 100 cells were counted).
Staining of hepatocytes with liver-specific markers
For staining, hepatocytes were cultured on collagen-coated coverslips. Cells were collected on Days 1 and 5. A cryosection of bovine liver was used as a control. Cells were fixed using methanol and paraformaldehyde (PFA). Methanol (at –20°C) was added to the cultures for 10 min. Next, the cells were rehydrated in Tris-buffered saline (TBS) for 5 min, followed by treatment with 10 mL of 10% PFA. The cells were then washed three times with TBS and non-specific interactions were blocked using 5% skimmed milk with 20% Tween. After 45 min, the blocking solution was removed and primary antibodies were added to the cells (1 : 1000 dilutions; mouse anti-CK18 monoclonal antibody (C8541; Sigma-Aldrich) and rabbit anti-panCK monoclonal antibody (17916; Sigma-Aldrich). Cells were incubated overnight at 4°C, then washed with TBS for 5 min and then incubated with secondary fluorescence antibodies for 3 h at room temperature (goat anti-rabbit IgG (green; Alexa Fluor 488) and goat anti-mouse IgG (red; Alexa Fluor 546); dilution of 1:2000). Cells were incubated with 500 µL staining solution for 45 min, then washed with Tris-buffered saline Tween-20 (TBST) for 5 min, followed by 4 min incubation with Hoechst dye nucleus stain (1:1000 in TBS; 33342; ThermoFisher Scientific). After a final washing step, cover slides were gently removed from the cell culture plate and mounted on a microscope slide with Prolong Gold anti-fade reagent (P36930; Invitrogen). Cells were examined under an inverted fluorescence microscope (Zeiss Axiovert 25 with phase contrast).
Detection of functional KCs with latex beads
To assess the vitality of the gathered KCs in the culture model, specific latex beads (2.0 µm; L2778; Sigma-Aldrich) were used as vitality markers. Vital KCs phagocytose fluorescent microspheric polystyrol particles, which can be detected microscopically. To avoid non-specific binding, latex beads were opsonised by incubation for 1 h at 37°C with 1:1 PBS/FBS. HMC and HKCid were incubated with latex beads at a ratio of 1:40 (KCs:latex beads) for 24 h. Then, plates were washed twice with PBS to remove any non-phagocytosed beads. The phagocytosed particles were counted using a fluorescence microscope with phase contrast equipment (Zeiss Axiovert 25) in four fields of view per well.
Urea concentrations and LDH activity in the cell culture medium
Urea concentrations were measured using a Urea Assay Kit (ab83362; Abcam) and LDH activity was measured using a Lactate Dehydrogenase Activity Assay Kit (Sigma-Aldrich), as described previously (Witte et al. 2019). Both assays were performed according to the manufacturers’ instructions (https://www.sigmaaldrich.com/catalogue/product/sigma/mak066?lang=deandregion=DE). The intra-assay CVs were 6.1% for urea and 5.2% for LDH.
Isolation of mRNA and RT-qPCR
The expression of S18, MX1, ISG-15, CXCL-10, CXCL-5, IGFBP-2, IGF-1 and albumin mRNA in cell samples was determined using RT-qPCR. To this end, total RNA was extracted using TRIzol reagent (Sigma-Aldrich), as described previously (Ehrhardt and Schmicke 2016). The extracted RNA was quantified using a NanoDrop One spectrophotometer (ThermoFisher Scientific). RNA quality was checked in HKCid samples using the 2100 Bioanalyzer System (Agilent 6000 Nano Kit). All samples had RNA integrity numbers (RIN) >8.5. A CFX96 C1000 Touch thermocycler (Real-Time PCR Detection System; Bio-Rad) was used to reverse transcribe mRNA into cDNA, as described previously (Witte et al. 2019). Each PCR contained 2.5 ng µL–1 cDNA, 4 µL HOT FIREPol EvaGreen qPCR Supermix (5×; Solis BioDyne) and 0.4 µM forward and reverse primers (Eurofins MWG Operon). The primer sequences and Accession numbers are listed in Table 1. The PCR cycler was programmed as follows: activation of the DNA polymerase at 95°C for 10 min, followed by 40 cycles of RNA denaturation at 95°C for 15 s, annealing at 60°C for 30 s and elongation at 72°C for 30 s. A melting curve analysis was performed between 55 and 95°C in 0.5°C increments to confirm the identity of the amplicons. Gene expression levels were determined by relative quantification (ΔΔCt method) using S18 as a reference gene. Determinations were performed in duplicate.
Statistical analyses
Statistical analyses were performed with GraphPad Prism 8. All data were tested for normal distribution using the Shapiro–Wilk normality test. Normally distributed data were evaluated using Student’s t-test. Data that were not normally distributed were evaluated using the Mann–Whitney U-test. The mRNA data are presented as ΔΔCt relative to a calibrator with respect to the different efficiencies of the primers. Data are presented as the mean ± s.d. if data was normally distributed, or mean ± 95% CI if data was not normally distributed. Differences were considered significant at two-tailed P < 0.01.
Results
Establishment of KC cocultures
On average, 23 827 812 hepatocytes were isolated per gram liver tissue, with 84% viability, and 387 340 KCs were isolated (Table 2). The hepatocytes showed typical cell morphology and vitality. Initially, KCs were morphologically round with a diameter of 10 µm (Fig. S1, Fig. S2). After Day 1, the cells developed typical stellate cell extensions (Fig. S1). Liver-specific markers were detected by immunostaining on Days 1 and 5 with CK18 and panCK (Fig. 3). On Day 2, 41.3% of KCs contained phagocytosed fluorescent latex beads. This decreased to 25.1% on Day 3 and to 13.6% on Day 4 (Fig. 4).
![]() |
Suitability of cell culture conditions during stimulation experiments
Mean viability after Percoll density centrifugation was 91.4 ± 1.9% in the six livers used for the experiments with IFNτ. The mean urea concentration in each experiment was comparable, with concentrations of 12.43 ± 0.94, 12.26 ± 1.25 and 12.62 ± 1.55 nmol well–1 in ES1 (HKCd), ES2 (HKCid) and ES3 (HMC) respectively. LDH concentrations were also comparable in each experiment: 3.32 ± 0.51, 1.79 ± 0.84 and 4.97 ± 2.89 mU well–1 in ES1, ES2 and ES3 respectively. There were also no significant differences in albumin expression (9.62 ± 1.85, 10.77 ± 2.21, 11.60 ± 1.91 and 12.09 ± 1.92 for basal albumin expression and expression in ES1, ES2 and ES3 respectively).
Basal expression of genes of interest
The intra-assay CVs for basal expression were 7.5% for MX1, 11.6% for ISG-15, 5.9% for CXCL-5, 7.4% for CXCL-10, 12.5% for IGF-1 and 7.9% for IGFBP-2 (Table 3). The relative mRNA expression of the target genes did not differ significantly between the six livers analysed in the stimulation experiments.
Stimulation of hepatocytes and KC coculture with IFNτ, IFNα and P4
The mRNA expression of ISGs (MX1, ISG-15, CXCL-5 and CXCL-10) increased in mono- and cocultures during the first days of culture until Day 3, but decreased sharply when morphological restructuring of hepatocytes was finished on Day 4 (Fig. 5). On Day 4, the expression of the inflammatory-/IFN-related genes MX1, ISG-15, CXCL-5 and CXCL-10 was back to baseline levels in HMC and HKCid. In contrast, mRNA levels of the metabolic genes (IGF-1 and IGFBP-2) increased towards Day 3 and remained higher on Day 4 than on Day 1. The overall expression profiles were comparable between mono- and cocultures (Fig. 5).
The addition of 0.6 ng mL–1 IFNτ upregulated ISG-15 and MX1 mRNA levels in HMC and HKCid (Fig. 6a, b). CXCL-10 mRNA levels increased sharply in HMC stimulated with 0.6 ng mL–1 IFNτ or IFNα, but not in HKCid after stimulation with 0.6 ng mL–1 IFNτ. In HKCid, the addition of 0.06 ng mL–1 IFNα resulted in significant upregulation of CXCL-10 mRNA expression (P = 0.0041; Fig. 6c). Stimulation of HMC with 0.06 ng mL–1 IFNτ or IFNα resulted in higher CXCL-5 mRNA expression than without stimulation (Fig. 6d). The addition of IFNα increased IGFBP-2 mRNA expression exclusively in HKCid, whereas the addition of IFNτ reduced the expression of IGFBP-2 (Fig. 6f).
The addition of P4 alone had no effect on MX1 and CXCL-10 mRNA expression in HMC or HKCid (Fig. 7a, c). However, CXCL-5 mRNA levels increased significantly after stimulation with 10 ng mL–1 P4 (P = 0.0055; Fig. 7d). The addition of IFNτ increased MX1, ISG-15, CXCL-10 and CXCL-5 mRNA expression, whereas additional stimulation with 5 ng mL–1 P4 reduced the expression of these four genes. This reduction was more pronounced when the P4 concentration was increased to 10 ng mL–1 (Fig. 7a–d). In HKCd, IGF-1 and IGFBP-2 mRNA expression was comparable in the presence and absence of P4, but decreased after stimulation with 0.6 ng mL–1 IFNτ alone. However, this decrease was reversed by the addition of 5 ng mL–1 P4 to the HKCd (Fig. 7e, f).
Discussion
Establishment of KC cocultures
The first aim of the present study was to establish a hepatocyte coculture with KCs to test whether hepatocytes respond to IFN directly or whether KCs transduce the signal instead. The hepatocyte sandwich culture had been established previously (Witte et al. 2019). The present work confirmed that, in a sandwich culture system, the shape of hepatocyte cells changed to cuboidal and polygonal, and hepatocytes formed cords reminiscent of the hepatic trabeculae with bile canaliculi, which can be detected as bright elucidations between hepatocytes. This has already been reported by Witte et al. (2019). The separation of sessile KCs from bovine livers was established using a previously established protocol (Smedsrød et al. 1985). KCs in culture were viable, as indicated by the phagocytosis of fluorescent latex beads. In HKCid cocultures, KC could be detected based on phagocytosed latex beads on Days 2, 3 and 4, suggesting immunologically active cells. KCs are located in the endothelium of liver sinusoids and are responsible for the phagocytosis of pathogens before they enter the liver, as well as the elimination of apoptotic endothelial cells. KCs are normally in an inactive state, but can be activated by inflammatory signals (Duque and Descoteaux 2014). The activation of sessile KCs can be differentiated into classical activation (e.g. by IFNγ or toll-like receptors (TLRs)/interleukin (IL)-1 receptors). This results in the production of proinflammatory cytokines, such as IL-1, IL-6, tumour necrosis factor-α, transforming growth factor-β, IFNγ, epidermal growth factor and leukaemia inhibitory factor (Gordon and Martinez 2010). In addition to the classical activation, IL-4 and/or IL-13 can also activate M2 macrophages (Stein et al. 1992; Wynn et al. 2013) to exhibit anti-inflammatory functions. The third group of activated KCs are regulatory KCs, which need two activation signals: a priming-signal from apoptotic cells, IL-10, prostaglandins or glucocorticoids and a second signal via their TLRs (Mosser and Edwards 2008). It would be interesting to determine whether the sessile KCs in the hepatocyte culture are inactivated or activated, but unfortunately mRNA expression analyses of factors differentiating between the two states were not performed in the present study.
The basal expression of the genes of interest varied less than 13% between the six livers from different donors. This leads to the assumption, that regardless of the donor animal, mono- and cocultures of hepatocytes lead to comparable results. During the 4 days of incubation, the expression profiles of MX1, ISG-15, CXCL-5 and CXCL-10 were also comparable. The expression of inflammatory genes was initially increased, which paralleled the morphological changes noticeable in the cultures and is suggestive of a proinflammatory state. Separation of hepatocytes leads to an increase in nuclear factor (NF)-κB expression (Li and Verma 2002; Vinken et al. 2014). NF-κB is an important regulator of inflammation (Li and Verma 2002) and may indirectly induce ISG via IFNβ (Sen and Sarkar 2007; Oeckinghaus and Ghosh 2009). Although not further examined in the present study, this may be a possible pathway for the upregulation of the chemokines investigated in this study.
Within the first 3 days of incubation, hepatocytes developed the specific polygonal cell shape, cell–cell contacts and canaliculi (Ehrhardt and Schmicke 2016; Witte et al. 2019). In parallel with an end to the morphological changes, the expression of inflammatory genes decreases. In agreement with Ehrhardt and Schmicke (2016) and Witte et al. (2019), this led us to conclude that Day 4 of culture is most suitable for experiments in primary bovine hepatocytes. Moreover, the continuously increasing IGFBP-2 mRNA expression levels throughout the 4 days of incubation indicate that the highest rate of metabolic synthesis is achieved on Day 4.
Effects of IFNτ versus IFNα on target gene expression in the HMC and HKCid models
Once established, the HMCid model was used to investigate the following questions: (1) is liver cell culture suitable for testing the effects of Type I IFNs; (2) does the effect of Type I IFNs differ between pure hepatocytes and hepatocytes cocultured with KCs; (3) does the expression of ISGs differ between hepatocytes stimulated with IFNτ and other Type I IFNs; and 4) does simultaneous stimulation with P4 and IFNτ affect the expression levels of individual target genes in the maternal liver?
As suspected, and previously shown in hepatocytes, stimulation with IFNτ induced classical ISGs (MX1 and ISG-15; Ruhmann et al. 2017). The stimulation was dose dependent and did not depend on the presence of sessile KCs because the expression levels were comparable between the HMC and HKCid models. Others have previously reported an increase in CXCL-10 expression in response to IFNα in human thyrocytes (Antonelli et al. 2010) and in response to IFNτ in sheep endometrial cells (Imakawa et al. 2006). In line with these findings, CXCL-10 mRNA expression was increased in response to the higher concentration of 0.6 ng mL–1 IFNτ in hepatocytes in HMC in the present study. CXCL-10 expression has previously been identified in KCs and in the uterus of sheep during the peri-implantation period (Fernandez and Lolis 2002; Imakawa et al. 2006; Dembic 2015). It has previously been shown that the endometrial IGF system changes in early pregnancy, and IFNτ has been speculated as a possible factor for changes in the endometrial gene expression (Robinson et al. 2008). Following on from this, Meyerholz et al. (2015) reported a decrease in IGFBP-2 during early pregnancy (towards Day 18 of gestation) in dairy heifers. IGFBP-2 isolated from blood samples is mainly produced in the liver. Therefore, the hypothesis that IFNτ directly affects hepatic IGFBP-2 production was confirmed in the present study. The addition of a low concentration (0.06 ng mL–1) of IFNτ to the HMC for 6 h led to a reduction in IGFBP-2 mRNA. Furthermore, the present study examined differences in responses to IFNτ compared with other Type I IFNs. For this comparison, IFNα was chosen as an alternative Type I IFN because it is normally produced by nearly all nucleated cells, especially leucocytes (Ohmann et al. 1987).
IFNα was shown to induce CXCL-5 and CXCL-10 mRNA expression but not the expression of MX1 and ISG-15. This appears to be a specific IFNτ pattern despite the fact that all Type I IFNs bind to the same receptor, namely IFNAR1/2 (Li and Roberts 1994; Mogensen et al. 1999; Uzé et al. 2007). Future studies will need to show why ISG-15 and MX1 mRNA expression is exclusively induced by IFNτ in primary hepatocytes and whether comparable effects can be observed in vivo. However, should be noted that in the present study only mRNA expression was evaluated, and not protein expression. This aspect should also be further investigated in future studies.
Effects of P4 versus IFNτ on target gene expression in HMC and HKCid
During early pregnancy, ISG expression is dependent on steroid hormones like P4 (Spencer et al. 2016). In heifers supplemented with P4 on Day 16 of pregnancy, ISG-15 and CXCL-10 mRNA expression was higher in uterine epithelial cells than in heifers with lower endogenous P4 concentrations (Forde et al. 2009). In the present study, based on physiological P4 concentrations on Day 16 of pregnancy (5–10 ng mL–1; Matsuyama et al. 2012), concentrations of 5 and 10 ng mL–1 P4 were used in the HKCid cultures to detect potential effects not only in reproductive tissues, but also in the liver. In liver cells, only ISG-15 mRNA expression was slightly increased in response to P4. There was no change in MX1 and CXCL-10 mRNA expression after stimulation with P4. After the addition of 5 ng mL–1 P4 and 0.6 ng mL–1 IFNτ to cultures, ISG-15 expression was higher than after the addition of 10 ng mL–1 P4 and 0.6 ng mL–1 IFNτ. This is in contrast to the results in endometrial tissue reported by Forde et al. (2009), who detected higher ISG-15 and CXCL-10 mRNA expression in samples stimulated with higher concentrations of P4. However, the P4 concentrations used by Forde et al. (2009) were higher in vivo than the concentrations used in the present in vitro study. Moreover, different cell types were used and the reaction to P4 may differ between the endometrium and liver. Further studies should also focus on respective hormone receptor expression and intracellular signal transduction pathways, which may differ in different tissues. Interestingly, CXCL-5 mRNA expression was only stimulated by P4 and the additional effect of IFNτ was lower compared with that on the other genes. Increased CXCL-5 expression in response to P4 was previously reported in vaginal tissue of mice after ovariectomy (Gillgrass et al. 2005). This led to speculation about the presence of a protection mechanism for pregnant animals against infectious particles during pregnancy. This is imaginable for the liver as well, because CXCL-5 attracts and activates neutrophils and induces an immune response (Dembic 2015). However, P4 receptors were not analysed in the present study, and therefore the comparison with reproductive tissue is debatable. After stimulation of the HKCid with IFNτ and P4, IGF-1 mRNA expression decreased, which is in line with previous studies. McCarthy et al. (2012) showed that IGF-1 expression on Day 16 after ovulation decreased significantly in pregnant but not cycling cows compared with expression on Day 5. Several other studies also detected lower IGF-1 expression during the luteal phase in endometrial (Meikle et al. 2001) and serum (Mense et al. 2015) samples.
The expression of IGFBP-2 was lower in HMC and HKCid after stimulation with P4 and IFNτ. As mentioned above, Meyerholz et al. (2015) also detected a decrease in blood IGFBP-2 in early pregnant cows (Day 14). However, McCarthy et al. (2012) detected an increase in IGFBP-2 mRNA expression in the endometrium from Day 7 towards Day 13. Because the liver is the main source of IGFBP-2, it can be hypothesised that lower peripheral IGFBP-2 may lead to higher free IGF-1 concentrations, whereas higher local IGFBP-2 may bind the additional IGF-1, which is essential for fetal growth (Lassarre et al. 1991).
In conclusion, primary bovine hepatocyte cultures were proven to be a suitable model for stimulation experiments with Type I IFNs and investigation of extrauterine embryo–maternal communication. The results of the present study show that there is a significant increase in MX1, ISG-15, CXCL-5, CXCL-10, IGF-1, IGFBP-2 mRNA expression in hepatocytes in response to stimulation with IFNτ. Thus, IFNτ has been confirmed to have a direct effect on the mRNA expression of target genes in hepatocytes. The KCs in cocultures seemed to make no major contribution to the effect of IFNτ on hepatocytes. In contrast, hepatocytes seem to respond differently when P4 was added to the cultures. Although MX1 und ISG-15 mRNA expression was stimulated by IFNτ in both hepatocytes and endometrial cells, the downregulation of IGFBP-2 mRNA expression was only detected in hepatocytes and not in the endometrium. The proposed endocrine action of IFNτ in the maternal liver may affect maternal metabolism, as well as maternal immune function in the liver. Further investigations are needed to elucidate the underlying mechanisms, which may be relevant for pregnancy establishment, and further research is required to examine potential systemic and endocrine effects on other organs during the early pregnancy in ruminants.
Conflicts of interest
The authors declare no conflicts of interest.
Acknowledgements
The authors thank the J. and G. Bruns Foundation for financial support. The authors also thank Martina Baumgarten, Angela Jordan and Sandra Wilkening. Finally, the authors thank the staff and the leadership of the Clinic for Cattle, University of Veterinary Medicine Hanover for their support during sample collection, especially W. Kehler.
References
Antonelli, A., Martina, S., Fallahi, P., Ghiri, E., Crescioli, C., Romagnani, P., Vitti, P., Serio, M., and Ferrannini, E. (2010). Cytokine Interferon-alpha, -beta and -gamma induce CXCL9 and CXCL10 secretion by human thyrocytes: Modulation by peroxisome proliferator-activated receptor-gamma agonists. Cytokine 50, 260–267.| Cytokine Interferon-alpha, -beta and -gamma induce CXCL9 and CXCL10 secretion by human thyrocytes: Modulation by peroxisome proliferator-activated receptor-gamma agonists.Crossref | GoogleScholarGoogle Scholar | 20299237PubMed |
Arosh, J. A., Banu, S. K., Kimmins, S., Chapdelaine, P., MacLaren, L. A., and Fortier, M. A. (2004). Effect of interferon-τ on prostaglandin biosynthesis, transport, and signaling at the time of maternal recognition of pregnancy in cattle: Evidence of polycrine actions of prostaglandin E2. Endocrinology 145, 5280–5293.
| Effect of interferon-τ on prostaglandin biosynthesis, transport, and signaling at the time of maternal recognition of pregnancy in cattle: Evidence of polycrine actions of prostaglandin E2.Crossref | GoogleScholarGoogle Scholar | 15308607PubMed |
Bott, R. C., Ashley, R. L., Henkes, L. E., Antoniazzi, A. Q., Bruemmer, J. E., Niswender, G. D., Bazer, F. W., Spencer, T. E., Smirnova, N. P., Anthony, R. V., and Hansen, T. R. (2010). Uterine vein infusion of interferon tau (IFNT) extends luteal life span in ewes. Biol. Reprod. 82, 725–735.
| Uterine vein infusion of interferon tau (IFNT) extends luteal life span in ewes.Crossref | GoogleScholarGoogle Scholar | 20042537PubMed |
Capobianchi, M. R., Uleri, E., Caglioti, C., and Dolei, A. (2015). Type I IFN family members: similarity, differences and interaction. Cytokine Growth Factor Rev. 26, 103–111.
| Type I IFN family members: similarity, differences and interaction.Crossref | GoogleScholarGoogle Scholar | 25466633PubMed |
Dembic, Z. (2015). ‘The cytokines of the immune system – The role of cytokines in disease related to immune response.’ (Academic Press)
Duque, G. A., and Descoteaux, A. (2014). Macrophage cytokines: involvement in immunity and infectious diseases. Front. Immunol. 5, 491.
| Macrophage cytokines: involvement in immunity and infectious diseases.Crossref | GoogleScholarGoogle Scholar |
Ehrhardt, S., and Schmicke, M. (2016). Isolation and cultivation of adult primary bovine hepatocytes from abattoir derived liver. EXCLI J. 15, 858–866.
| Isolation and cultivation of adult primary bovine hepatocytes from abattoir derived liver.Crossref | GoogleScholarGoogle Scholar | 28275320PubMed |
Fernandez, E. J., and Lolis, E. (2002). Structure, function, and inhibition of chemokines. Annu. Rev. Pharmacol. Toxicol. 42, 469–499.
| Structure, function, and inhibition of chemokines.Crossref | GoogleScholarGoogle Scholar | 11807180PubMed |
Forde, N., Carter, F., Fair, T., Crowe, M. A., Evans, A. C. O., Spencer, T. E., Bazer, F. W., Mcbride, R., Boland, M. P., Gaora, P. O., Lonergan, P., and Roche, J. F. (2009). Progesterone-regulated changes in endometrial gene expression contribute to advanced conceptus development in cattle. Biol. Reprod. 81, 784–794.
| Progesterone-regulated changes in endometrial gene expression contribute to advanced conceptus development in cattle.Crossref | GoogleScholarGoogle Scholar | 19553605PubMed |
Gillgrass, A. E., Fernandez, S. A., Rosenthal, K. L., and Kaushic, C. (2005). Estradiol regulates susceptibility following primary exposure to genital herpes simplex virus type 2, while progesterone induces inflammation. J. Virol. 79, 3107–3116.
| Estradiol regulates susceptibility following primary exposure to genital herpes simplex virus type 2, while progesterone induces inflammation.Crossref | GoogleScholarGoogle Scholar | 15709030PubMed |
Gordon, S., and Martinez, F. O. (2010). Alternative activation of macrophages: mechanism and functions. Immunity 32, 593–604.
| Alternative activation of macrophages: mechanism and functions.Crossref | GoogleScholarGoogle Scholar | 20510870PubMed |
Han, H., Austin, K. J., Rempel, L. A., and Hansen, T. R. (2006). Low blood ISG15 mRNA and progesterone levels are predictive of non-pregnant dairy cows. J. Endocrinol. 191, 505–512.
| Low blood ISG15 mRNA and progesterone levels are predictive of non-pregnant dairy cows.Crossref | GoogleScholarGoogle Scholar | 17088421PubMed |
Imakawa, K., Anthony, R. V., Kazemi, M., Marotti, K. R., Polites, H. G., and Roberts, R. M. (1987). Interferon-like sequence of ovine trophoblast protein secreted by embryonic trophectoderm. Nature 330, 377–379.
| Interferon-like sequence of ovine trophoblast protein secreted by embryonic trophectoderm.Crossref | GoogleScholarGoogle Scholar | 2446135PubMed |
Imakawa, K., Imai, M., Sakai, A., Suzuki, M., Nagaoka, K., Sakai, S., Lee, S. R., Chang, K.-T., Echternkamp, S. E., and Christenson, R. K. (2006). Regulation of conceptus adhesion by endometrial CXC chemokines during the implantation period in sheep. Mol. Reprod. Dev. 73, 850–585.
| Regulation of conceptus adhesion by endometrial CXC chemokines during the implantation period in sheep.Crossref | GoogleScholarGoogle Scholar | 16596627PubMed |
Kunii, H., Koyama, K., Ito, T., Suzuki, T., Balboula, A. Z., Shirozu, T., Bai, H., Nagano, M., Kawahara, M., and Takahashi, M. (2018). Hot topic: pregnancy-induced expression of interferon-stimulated genes in the cervical and vaginal mucosal membranes. J. Dairy Sci. 101, 8396–8400.
| Hot topic: pregnancy-induced expression of interferon-stimulated genes in the cervical and vaginal mucosal membranes.Crossref | GoogleScholarGoogle Scholar | 29935833PubMed |
Lassarre, C., Hardouin, S., Daffos, F., Forestier, F., Frankenne, F., and Binoux, M. (1991). Serum insulin-like growth factors and insulin-like growth factor binding proteins in the human fetus. Relationships with growth in normal subjects and in subjects with intrauterine growth retardation. Pediatr. Res. 29, 219–225.
| Serum insulin-like growth factors and insulin-like growth factor binding proteins in the human fetus. Relationships with growth in normal subjects and in subjects with intrauterine growth retardation.Crossref | GoogleScholarGoogle Scholar | 1709729PubMed |
Li, J., and Roberts, R. M. (1994). Interferon-τ and interferon-α interact with the same receptors in bovine endometrium. Use of a readily iodinatable form of recombinant interferon-τ for binding studies. J. Biol. Chem. 269, 13544–13550.
| Interferon-τ and interferon-α interact with the same receptors in bovine endometrium. Use of a readily iodinatable form of recombinant interferon-τ for binding studies.Crossref | GoogleScholarGoogle Scholar | 8175789PubMed |
Li, Q., and Verma, I. M. (2002). NF-κB regulation in the immune system. Nat. Rev. Immunol. 2, 725–734.
| NF-κB regulation in the immune system.Crossref | GoogleScholarGoogle Scholar | 12360211PubMed |
Matsuyama, S., Kojima, T., Kato, S., and Kimura, K. (2012). Relationship between quantity of IFNT estimated by IFN-stimulated gene expression in peripheral blood mononuclear cells and bovine embryonic mortality after AI or ET. Reprod. Biol. Endocrinol. 10, 21.
| Relationship between quantity of IFNT estimated by IFN-stimulated gene expression in peripheral blood mononuclear cells and bovine embryonic mortality after AI or ET.Crossref | GoogleScholarGoogle Scholar | 22439976PubMed |
McCarthy, S. D., Roche, J. F., and Forde, N. (2012). Temporal changes in endometrial gene expression and protein localization of members of the IGF family in cattle: effects of progesterone and pregnancy. Physiol. Genomics 44, 130–140.
| Temporal changes in endometrial gene expression and protein localization of members of the IGF family in cattle: effects of progesterone and pregnancy.Crossref | GoogleScholarGoogle Scholar | 22085906PubMed |
Meikle, A., Sahlin, L., Ferraris, A., Masironi, B., Blanc, J. E., Rodríguez-Irazoqui, M., Rodríguez-Piñón, M., Kindahl, H., and Forsberg, M. (2001). Endometrial mRNA expression of oestrogen receptor α, progesterone receptor and insulin-like growth factor-I (IGF-I) throughout the bovine oestrous cycle. Anim. Reprod. Sci. 68, 45–56.
| Endometrial mRNA expression of oestrogen receptor α, progesterone receptor and insulin-like growth factor-I (IGF-I) throughout the bovine oestrous cycle.Crossref | GoogleScholarGoogle Scholar | 11600273PubMed |
Mense, K., Meyerholz, M., Gil Araujo, M., Lietzau, M., Knaack, H., Wrenzycki, C., Hoedemaker, M., and Piechotta, M. (2015). The somatotropic axis during the physiological estrus cycle in dairy heifers – effect on hepatic expression of GHR and SOCS2. J. Dairy Sci. 98, 2409–2418.
| The somatotropic axis during the physiological estrus cycle in dairy heifers – effect on hepatic expression of GHR and SOCS2.Crossref | GoogleScholarGoogle Scholar | 25704974PubMed |
Meyerholz, M. M., Mense, K., Lietzau, M., Kassens, A., Linden, M., Knaack, H., Wirthgen, E., Hoeflich, A., Raliou, M., Richard, C., Sandra, O., Schuberth, H., Hoedemaker, M., and Schmicke, M. (2015). Serum IGFBP4 concentration decreased in dairy heifers towards day 18 of pregnancy. J. Vet. Sci. 16, 413–421.
| Serum IGFBP4 concentration decreased in dairy heifers towards day 18 of pregnancy.Crossref | GoogleScholarGoogle Scholar | 26243597PubMed |
Mogensen, K. E., Lewerenz, M., Reboul, J., Lutfalla, G., and Uzé, G. (1999). The type I interferon receptor: structure, function, and evolution of a family business. J. Interferon Cytokine Res. 19, 1069–1098.
| The type I interferon receptor: structure, function, and evolution of a family business.Crossref | GoogleScholarGoogle Scholar | 10547147PubMed |
Mosser, D. M., and Edwards, J. P. (2008). Exploring the full spectrum of macrophage activation. Nat. Rev. Immunol. 8, 958–969.
| Exploring the full spectrum of macrophage activation.Crossref | GoogleScholarGoogle Scholar | 19029990PubMed |
Naito, M., Hasegawa, G., and Yamamoto, J. T. (2004). Differentiation and function of Kupffer cells. Med. Electron Microsc. 37, 16–28.
| Differentiation and function of Kupffer cells.Crossref | GoogleScholarGoogle Scholar | 15057601PubMed |
Nakamura, K., Kusama, K., Bai, R., Sakurai, T., Isuzugawa, K., Godkin, J. D., Suda, Y., and Imakawa, K. (2016). Induction of IFNT-stimulated genes by conceptus-derived exosomes during the attachment period. PLoS One 11, e0158278.
| Induction of IFNT-stimulated genes by conceptus-derived exosomes during the attachment period.Crossref | GoogleScholarGoogle Scholar | 27649071PubMed |
Oeckinghaus, A., and Ghosh, S. (2009). The NF-κB family of transcription factors and its regulation. Cold Spring Harb Perspect Biol. 1, a000034.
| The NF-κB family of transcription factors and its regulation.Crossref | GoogleScholarGoogle Scholar | 20066092PubMed |
Ohmann, H. B., Lawman, M. J. P., and Babiuk, L. A. (1987). Bovine interferon: its biology and application in veterinary medicine. Antiviral Res. 7, 187–210.
| Bovine interferon: its biology and application in veterinary medicine.Crossref | GoogleScholarGoogle Scholar | 2441661PubMed |
Oliveira, J. F., Henkes, L. E., Ashley, R. L., Purcell, S. H., Smirnova, N. P., Veeramachaneni, D. N. R., Anthony, R. V., and Hansen, T. R. (2008). Expression of interferon (IFN)-stimulated genes in extrauterine tissues during early pregnancy in sheep is the consequence of endocrine IFN-τ release from the uterine vein. Endocrinology 149, 1252–1259.
| Expression of interferon (IFN)-stimulated genes in extrauterine tissues during early pregnancy in sheep is the consequence of endocrine IFN-τ release from the uterine vein.Crossref | GoogleScholarGoogle Scholar | 18063687PubMed |
Roberts, R. M., Liu, L., Guo, Q., Leaman, D. W., and Bixby, J. (1998). The evolution of the type I interferons. J. Interferon Cytokine Res. 18, 805–816.
| The evolution of the type I interferons.Crossref | GoogleScholarGoogle Scholar | 9809615PubMed |
Robinson, R. S., Hammond, A. J., Wathes, D. C., Hunter, M. G., and Mann, G. E. (2008). Corpus luteum–endometrium–embryo interactions in the dairy cow: underlying mechanisms and clinical relevance. Reprod. Domest. Anim. 43, 104–112.
| Corpus luteum–endometrium–embryo interactions in the dairy cow: underlying mechanisms and clinical relevance.Crossref | GoogleScholarGoogle Scholar | 18638111PubMed |
Romero, J. J., Antoniazzi, A. Q., Nett, T. M., Ashley, R. L., Webb, B. T., Smirnova, N. P., Bott, R. C., Bruemmer, J. E., Bazer, F. W., Anthony, R. V., and Hansen, T. R. (2015). Temporal release, paracrine and endocrine actions of ovine conceptus-derived interferon-tau during early pregnancy. Biol. Reprod. 93, 146.
| Temporal release, paracrine and endocrine actions of ovine conceptus-derived interferon-tau during early pregnancy.Crossref | GoogleScholarGoogle Scholar | 26559679PubMed |
Ruhmann, B., Giller, K., Hankele, A. K., Ulbrich, S. E., and Schmicke, M. (2017). Interferon-τ induced gene expression in bovine hepatocytes during early pregnancy. Theriogenology 104, 198–204.
| Interferon-τ induced gene expression in bovine hepatocytes during early pregnancy.Crossref | GoogleScholarGoogle Scholar | 28888122PubMed |
Sen, G. C., and Sarkar, S. N. (2007). The Interferon-Stimulated Genes: Targets of Direct Signaling by Interferons, Double-Stranded RNA, and Viruses. In ‘Interferon: The 50th Anniversary’. (Ed. P. M. Pitha.) pp. 233–250. (Springer.)
Smedsrød, B., Pertoft, H., Eggertsen, G., and Sundström, C. (1985). Functional and morphological characterization of cultures of Kupffer cells and liver endothelial cells prepared by means of density separation in Percoll, and selective substrate adherence. Cell Tissue Res. 241, 639–649.
| Functional and morphological characterization of cultures of Kupffer cells and liver endothelial cells prepared by means of density separation in Percoll, and selective substrate adherence.Crossref | GoogleScholarGoogle Scholar | 2992796PubMed |
Spencer, T. E., Forde, N., and Lonergan, P. (2016). The role of progesterone and conceptus-derived factors in uterine biology during early pregnancy in ruminants1. J. Dairy Sci. 99, 5941–5950.
| The role of progesterone and conceptus-derived factors in uterine biology during early pregnancy in ruminants1.Crossref | GoogleScholarGoogle Scholar | 26387021PubMed |
Stein, M., Keshav, S., Harris, N., and Gordon, S. (1992). Interleukin 4 potently enhances murine macrophage mannose receptor activity: a marker of alternative immunologic macrophage activation. J. Exp. Med. 176, 287–292.
| Interleukin 4 potently enhances murine macrophage mannose receptor activity: a marker of alternative immunologic macrophage activation.Crossref | GoogleScholarGoogle Scholar | 1613462PubMed |
Teixeira, M. G., Austin, K. J., Perry, D. J., Dooley, V. D., Johnson, G. A., Francis, B. R., and Hansen, T. R. (1997). Bovine granulocyte chemotactic protein-2 is secreted by the endometrium in response to interferon-tau (IFN-τ). Endocrine 6, 31–37.
| Bovine granulocyte chemotactic protein-2 is secreted by the endometrium in response to interferon-tau (IFN-τ).Crossref | GoogleScholarGoogle Scholar | 9225113PubMed |
Uzé, G., Schreiber, G., Piehler, J., and Pellegrini, S. (2007). The Receptor of the Type I Interferon Family. In ‘Interferon: The 50th Anniversary’. (Ed. P. M. Pitha.) pp. 71–90. (Springer.)
Vinken, M., Maes, M., Oliveira, A. G., Cogliati, B., Marques, P. E., Menezes, G. B., Dagli, M. L. Z., Vanhaecke, T., and Rogiers, V. (2014). Primary hepatocytes and their cultures in liver apoptosis research. Arch. Toxicol. 88, 199–212.
| Primary hepatocytes and their cultures in liver apoptosis research.Crossref | GoogleScholarGoogle Scholar | 24013573PubMed |
Witte, S., Brockelmann, Y., Haeger, J. D., and Schmicke, M. (2019). Establishing a model of primary bovine hepatocytes with responsive growth hormone receptor expression. J. Dairy Sci. 102, 7522–7535.
| Establishing a model of primary bovine hepatocytes with responsive growth hormone receptor expression.Crossref | GoogleScholarGoogle Scholar | 31155243PubMed |
Wynn, T. A., Chawla, A., and Pollard, J. W. (2013). Origins and hallmarks of macrophages: development, homeostasis, and disease. Nature 496, 445–455.
| Origins and hallmarks of macrophages: development, homeostasis, and disease.Crossref | GoogleScholarGoogle Scholar | 23619691PubMed |
Yankey, S. J., Hicks, B. A., Carnahan, K. G., Assiri, A. M., Sinor, S. J., Kodali, K., Stellflug, J. N., and Ott, T. L. (2001). Expression of the antiviral protein Mx in peripheral blood mononuclear cells of pregnant and bred, non-pregnant ewes. J. Endocrinol. 170, R7–R11.
| Expression of the antiviral protein Mx in peripheral blood mononuclear cells of pregnant and bred, non-pregnant ewes.Crossref | GoogleScholarGoogle Scholar | 11479146PubMed |