Igniting river health? Testing the effectiveness of low intensity burns to improve riparian vegetation quality in modified ecosystems
Elisha Duxbury A * , Kirstie Fryirs
A
B
Abstract
Fire is largely untested for controlling invasive exotic plant species in highly modified riparian systems.
This study tested a low intensity burn in combination with herbicide application for reducing cover of three riparian woody weeds and increasing the presence of native plant species.
We surveyed native and exotic riparian vegetation and three target woody weeds over 1 year at a river reach that underwent herbicide treatment and a low intensity burn. Fire temperatures were recorded at the soil surface and 10-cm depth.
Maximum temperatures in burned areas exceeded 399°C at the soil surface and reached 47.1°C at 10-cm depth. Exotic midstorey species richness and woody riparian weed cover were considerably less 3-months after burning and herbicide treatment compared with herbicide treatment alone, then subsequently increased.
A combination of herbicide treatment with follow-up low intensity burning may be effective in reducing woody weed cover in the short term. However, fire temperatures recorded during the burn may not be hot enough to trigger fire-promoted native species germination.
Using low intensity burns in riparian systems to promote native plant regeneration has promise, however, integrated weed management utilising fire in highly modified riparian systems will require follow-up interventions within a relatively short timeframe.
Keywords: cultural burning, geomorphic recovery, low intensity burn, novel ecosystem, soil temperature, riparian ecology, river management, vegetation management.
Introduction
Land clearing has led to widespread ecological and geomorphic degradation of the world’s rivers (Brown et al. 2017; Wohl 2019). In coastal catchments of New South Wales (NSW), over 200 years of intense anthropogenic disturbance since European colonisation has led to the loss of geomorphic and vegetation condition of riparian systems (Richardson et al. 2007; Fryirs et al. 2013; Catford and Jansson 2014). However, since the end of the 20th century, the geomorphic and vegetative condition of rivers in coastal catchments of NSW has been improving, likely due to a mix of changing climate promoting increased plant growth, improved land management and active river rehabilitation (Fryirs et al. 2018; Cohen et al. 2022). In particular, riparian and instream vegetation cover has significantly increased since the 1980s in a phase referred to as ‘regreening’ (Cohen et al. 2022; Zhang and Fryirs 2023).
The reciprocal relationship between vegetation and geomorphic processes drives river recovery (Corenblit et al. 2014; Fryirs et al. 2018). In recovering rivers, vegetative recovery is the noticeable increase in woody vegetation cover in the riparian zone (Mabbott and Fryirs 2022; Zhang and Fryirs 2023). Geomorphic recovery is defined as an improvement in the physical structure and function of a river (Fryirs and Brierley 2016; Fryirs et al. 2018). The vegetation facilitating geomorphic recovery may be comprised of either native or exotic species, or both. However, preliminary research shows that a large proportion of returning vegetation in coastal NSW is dominated by exotic weeds, potentially leading to poor biodiversity outcomes in riparian corridors (O’Donnell et al. 2015, 2016; Duxbury et al. 2024). In some riparian systems, invasive exotic species incursions are so widespread and established that cost and time restraints hinder their effective rehabilitation. There is a need for large-scale, cost-effective techniques for managing the quality of riparian vegetation to improve biodiversity outcomes (McDonald and Williams 2009).
Fire presents a largely untested method for controlling invasive exotic species in riparian zones (Mandle et al. 2011; Bixby et al. 2015; Brancatelli et al. 2024). It has potential to be cost-effective and to work at-scale. Indigenous people in Australia have used fire for millennia to ‘clean up Country’ for cultural and spiritual reasons and to manage natural resources (Yibarbuk et al. 2001; Gott 2005). Low intensity burns are often perceived as the most beneficial for managing Australian native vegetation and are thought to cause mortality of exotic species propagules, and trigger germination of fire-adapted native species (Leavesley 2020). Low intensity burns are characterised by low flame height (<1.5 m), slow rate of spread (<100 m/h), and intensities less than 500 kW/m (Australasian Fire and Emergency Service Authorities Council Limited (AFAC) 2016). Low intensity burning may be used independently or in combination with other weed management techniques, such as herbicide application to target invasive species (Radford et al. 2008; Mabbott and Fryirs 2018). However, some native species and ecosystems do benefit from more intense fires and need hotter burns to cause germination in pyrogenic species (Bradstock and Auld 1995; Le Breton et al. 2020).
Burns conducted at low intensities are sometimes referred to as ‘cool burns’, though this term is poorly defined (Leavesley 2020). Cultural burning is conducted by Indigenous practitioners and is broadly defined as ‘burning practices developed by Aboriginal people to enhance the health of the land and its people’ (Firesticks Alliance Indigenous Corporation 2019). For example, a description of Cultural burning given by a Thungghutti practitioner is, ‘a burn done by Indigenous people aiming to bring back native vegetation and animals and keep them safe’ (Thungghutti Natural Regeneration Team, pers. comm. 2024). Hereafter, we will refer to low intensity burns as those that burn at cool temperatures with slow rates of spread and low flame heights. We will refer to burns conducted by Indigenous practitioners in the context of Indigenous values systems as Cultural burns.
Many ecosystems on recovering rivers are heavily modified and have no historic analogues (Fryirs et al. 2018). These systems have undergone anthropogenic impacts that cause irreversible changes to biotic and abiotic conditions, creating ‘novel’ ecosystems where species exist in combinations and abundances that have not previously occurred (Hobbs et al. 2006). As a result, burn regimes that were applied in perceived ‘natural’ or historic vegetation communities may no longer be appropriate for use in novel vegetation communities (Brooks et al. 2004; Enright et al. 2015). In addition, climate change is causing conditions to change so that riparian zones that had not previously burned are now susceptible to unprecedented bushfires because very dry years are causing the usually wet riparian biomass to dry and create large fuel loads (Pettit and Naiman 2007; Fryirs et al. 2022). For example, nearly 30% of riparian stream length in coastal catchments of NSW burned at high to extreme severity during the 2019–2020 Black Summer bushfires (Fryirs et al. 2022). Low intensity prescribed burning may now be necessary in highly modified riparian ecosystems to manage fuel loads, in addition to being used as a management method in novel riparian systems where environmental weeds are a significant issue.
Research into the use of fire to control woody exotic species in terrestrial shrubland, grassland and forest ecosystems has yielded promising results (Brancatelli et al. 2024). However, results on its effectiveness at specific sites are mixed (Mandle et al. 2011; Brancatelli et al. 2024). Few studies explore prescribed burning in highly modified ecosystems, or in riparian ecosystems (examples include Radford et al. 2008; Hankins 2013; Douglas et al. 2015; Mabbott and Fryirs 2018). While certain riparian systems act as refugia for fire sensitive species, other riparian species are adapted to frequent disturbance and may respond well to low intensity burns (Franklin et al. 2009; Petty and Douglas 2009; Bixby et al. 2015; Douglas et al. 2015; Le Breton et al. 2020). Additionally, Indigenous people in Australia and globally apply fire to certain riparian zones guided by seasonal cues for access, for spiritual reasons, to ‘care for Country’, and other reasons (Hankins 2013; Steffensen 2020; Thungghutti Natural Regeneration Team, pers. comm. 2024).
Existing research in riparian systems in northern Queensland and California has found that fire is effective in reducing target woody weed cover (Radford et al. 2008) and increasing the presence of native species (Hankins 2013). Riparian burning is a recommended management method for reducing the presence of the invasive riparian woody weed rubber vine (Cryptostegia grandiflora) in tropical savannas in eastern Australia (Bebawi and Campbell 2000, 2002; Radford et al. 2008). Radford et al. (2008) found burning riparian zones to control rubber vine did not have any irreversible effects on native riparian vegetation nor lead to ecological degradation. In the western United States of America, Hankins (2013) found that fire positively impacted native species richness in riparian vegetation due to reduced exotic species cover in the short term. They found that repeated burning every 2–5 years was necessary to maintain native species dominance (Hankins 2013).
Three common woody weeds in riparian zones in eastern Australia are lantana (Lantana camara), blackberry (Rubus anglocandicans), and madeira vine (Anredera cordifolia). These species pose significant biodiversity threats, with lantana and blackberry being identified as Weeds of National Significance (Department of Primary Industries (DPI) 1999). We could not find any studies on the use of fire to control blackberry, lantana, or madeira vine within a riparian context, though some studies exist on the use of fire to control them in other terrestrial environments (e.g. Ainsworth and Mahr 2004, 2006; Raizada and Raghubanshi 2010; Yeates and Schooler 2011; Ross et al. 2023). Hankins (2013) found that fire reduced the cover and exposed root crowns of a closely related blackberry species, Himalayan blackberry (Rubus discolor). There are no published studies on the use of fire to control madeira vine. Even results from studies in other terrestrial settings are mixed. Both lantana and blackberry can resprout post-fire, and short-term studies have found that these species recover after fire, or fire may trigger lantana germination (Gentle and Duggin 1997; Ainsworth and Mahr 2004, 2006; Raizada and Raghubanshi 2010). However, studies that track responses of competition between lantana and blackberry and native species after annual fire treatement show promising results (Ainsworth and Mahr 2004, 2006; Osunkoya et al. 2013). Further studies are needed to understand if and how fire can be used as an integrated weed management technique in riparian systems.
Our study was embedded within a broader program conducted by the Thungghutti Local Aboriginal Land Council and North Coast Local Land Services, local government authority aiming to rehabilitate a river reach of cultural significance to the local Thunggutti Traditional Custodians. Aims of this program were to heal part of the landscape that had been harmed by colonial land management practices, to recreate a meeting space that could be visited by local Thungghutti community members, and to provide employment opportunities for young people in the Thungghutti community. The experiment in our study focussed on determining the effectiveness of using a combination of techniques. Namely, treating target woody weeds with herbicide, which is considered business-as-usual in Australia, followed by burning the dried treated vegetation to clear debris, to suppress weeds and trigger the germination of native species to enhance biodiversity value. The aim of applying a Cultural burn was to conduct burning at a relatively low intensity during the winter months. This type of burn was deemed to be culturally suitable for the riparian vegetation community at the study site, which comprised a combination of rainforest-adapted and sclerophyll species. The broader Thungghutti project provided an opportunity to not only undertake an assessment of vegetation responses to the burn, but also to gather foundation data on the characteristics of low intensity and Cultural burns in these systems.
The aims of our study were to (1) quantify low intensity burn temperatures of this riparian system, and (2) assess the effectiveness of the combination of herbicide treatment followed by low intensity burning on native riparian vegetation recovery, and on three key woody weed species: lantana (Lantana camara), blackberry (Rubus anglocandicans) and madeira vine (Anredera cordifolia). We focussed on characterising soil surface and 10-cm depth temperatures before, during and after the burn. We expected that:
Native plant species richness and percentage cover would be higher in herbicide plus burn plots than in herbicide alone plots, and this cover would be higher 12 months post-burn than prior to the burn.
The cover and species richness of exotic species would be lower in plots treated with fire and herbicide compared with those treated with herbicide alone 12 months after burning.
The cover of three target woody weeds would be lower in plots treated with fire and herbicide compared with those treated with herbicide alone 12 months after burning.
Regional setting
This study was conducted on a 175 m long reach of Nulla Nulla Creek near Bellbrook (Wupu Manhatinum), New South Wales (NSW), in the Macleay River Catchment (Thunghutti Burrai) (Fig. 1). It is approximately 50 km west of Kempsey, NSW, and 470 km north of Sydney. The treatment reach is on the traditional lands of the Thungghutti people of the Macleay River Valley. Nulla Nulla Creek is a tributary of the Macleay River, and the junction of the two waterways adjacent to our study site is a place of cultural significance to the local Thungghutti peoples (Thungghutti Natural Regeneration Team, pers. comm. 2024). It was historically a meeting place and is still frequently used as a fishing spot. Prior to European colonisation, the site was maintained by frequent fire, which was applied through mosaic burns approximately annually, after leaving camp to care for country and to encourage the return of favourable flora and fauna. After European colonisation, Cultural burning ceased and has not resumed until recent years. Bushfires affected areas adjacent to the study site in 2001/2002, 2006/2007, 2013/2014, and 2019/2020; however, none directly affected the study reach (NSW DCCEEW SEED Fire History dataset (NSW DCCEEW 2010)). Since colonisation numerous changes to the region have occurred. Industry involving cutting of native red cedar (Toona ciliata) brought European colonisers to the Macleay region in the 1930s (National Parks and Wildlife Service New South Wales (NPWS) et al. 2003). Farming settlements began at the same time, leading to widespread land clearing on floodplains (National Parks and Wildlife Service New South Wales (NPWS) et al. 2003) and to displacement of local Indigenous peoples (National Parks and Wildlife Service New South Wales (NPWS) et al. 2003).
Site map showing the location of Bellbrook, NSW Australia, where Nulla Nulla Creek is located. The reach of Nulla Nulla Creek surveyed for this study was originally fitted with eight plots. The test (burned) and control (unburned) areas for this study needed to be retrofitted after a patchy burn. The map shows the post-burn burned and unburned survey areas. The eighth plot and other green areas were excluded from the final data analysis.
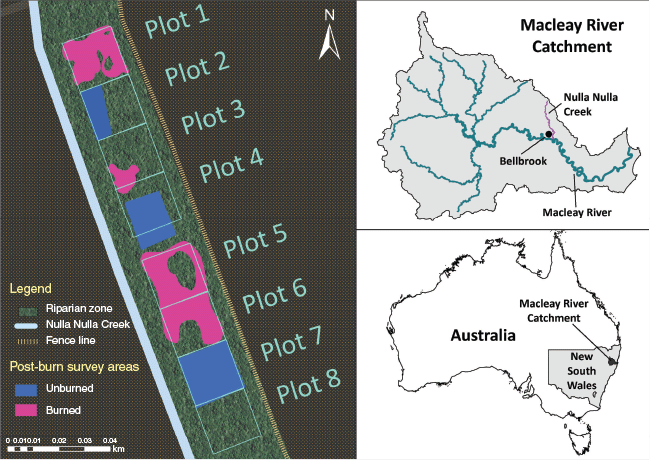
The Macleay catchment is in the North Coast Bioregion of NSW. The region has a subtropical climate characterised by hot summers and mild winters. Mean annual temperatures range between 20.1 and 29.5°C. The mean annual rainfall is 962.6 mm (Bureau of Meteorology 2024). Average local temperatures in August 2022, the month the burn took place, ranged between a minimum of 6°C to a maximum of 21°C (BOM 2024). That winter was the wettest on record in the state of NSW, with monthly average August 2022 rainfall of 47 mm. Soils derived from basalt occur to the north of the North Coast Bioregion (SEED, accessed April 2024). Historically, forms of Eastern Riverine Forests and Subtropical Rainforest were likely the most common riparian communities to occur around rivers in the area. Eastern Riverine Forest is dominated by river oak (Casuarina cunninghamiana), bottlebrush (Callistemon viminallis), and black tea-tree (Melaleuca bracteata) on the toe of banks, rainforest-adapted species and blackwood (Acacia melanoxylon) on the mid-bank, and cabbage gum (Eucalyptus amplifolia), forest red gum (Eucalyptus tereticornis) and broad-leaved apple (Angophora subvelutina) on the upper bank (SEED, accessed April 2024). Following colonisation, much of the original vegetation in the region was cleared and replaced with cattle grazing and paddocks largely covered by exotic grasses. Riparian corridors on low lying rivers in the region now generally consist of a mixture of native and exotic species with breaks in connectivity between vegetated patches.
The riparian community on Nulla Nulla Creek is now a highly modified Eastern Riverine Forest consisting of a mixture of native and exotic species, with exotic species being considerably more prominent (NSW SEED, accessed April 2024). The groundcover layer is dominated by the exotic grass broad-leaved paspalum (Paspalum mandiocanum) with scattered patches of native grasses, including blady grass (Imperata cylindrica) and tussock (Poa labillardierei). The midstorey comprises largely of exotic woody weeds with a few individuals of blackwood (Acacia melanoxylon), bottlebrush (Callistemon viminallis), black tea-tree (Melaleuca bracteata) and rainforest-adapted species (Mallotus philippensis, Maclura cochinchinensis). The canopy is mostly absent except for a few riveroak and native rough-leaved elm (Aphananthe philippinensis). Both the canopy and midstorey layer are infested with woody weeds including blackberry, lantana and exotic woody vines, such as madeira vine (Anredera cordifolia), white passionfruit (Passiflora subpeltata), and Dutchman’s pipe (Aristolochia elegans). Nulla Nulla Creek in is moderate geomorphic condition according to the NSW River Styles database (accessed May 2024). The banks are steeped and sloped, likely due to past erosion caused by historic vegetation clearing and subsequent channel widening. The river corridor is now moderately well-vegetated but there are few signs of ongoing erosion. Lateral deposition of cobble stone point bars is present in the river channel, though bench formation is only in the early stages and is in the process of becoming revegetated with canopy species.
Methods
Vegetation surveys
Pre- and post-burn vegetation surveys were undertaken using a Before-After-Control-Impact (BACI) experimental design over a 3500 m2 area running parallel to the study reach (Underwood 1991). The site was divided into (1) treatment areas, which were treated with both herbicide application and low intensity burning, and (2) control areas that were only treated with herbicide across a total of eight plots. Each plot was marked with a metal star pickets located in the south-east corner to mark its location for post-burn vegetation surveys along the 175 m reach (Fig. 1). The plots measuring 20 m × 20 m were located immediately adjacent to each other with exception of a 5 m gap between the fourth and fifth plots. Quadrats were utilised to sub-sample within each plot. Plots were assigned as either areas to be treated with herbicide and fire or only herbicide. We built a fallback plan into the design given that patchiness of the burn is a common issue with prescribed and cultural burn experiments. To account for this, we surveyed twice as many quadrats as necessary in our pre-burn vegetation surveys to have 30 useable quadrats per treatment even if the burn was incomplete. Burn and control areas were reconfigured after the burn was conducted to account for the irregular burn pattern (Fig. 1).
The original design was to burn plots one to four and leave plots five to eight unburned (Fig. 1). The 5 m gap between the fourth and fifth plots was to act as a buffer in case the burn spread beyond the planned area. Due to the unusually wet weather conditions of the 2022 La Niña year, the presence of exotic broad-leaved paspalum, which had low flammability, and the nature of Indigenous fire practices that allow a fire to progress slowly, the low intensity burn was patchy and parts of the site that were planned to burn did not burn. Other parts that had not initially been expected to burn were burned instead. As a result, only a quarter of the area that was expected to burn did burn. However, half of the expected unburned area did burn due to the presence of dried woody weeds and native ferns. The eighth plot was excluded from the study due to the unusually wet vegetation in this area.
A baseline vegetation survey was conducted before burning or any herbicide application occurred. After herbicide treatment, a second pre-burn vegetation survey was conducted, focusing only on woody midstorey plants. Post-burn surveys were conducted 3, 6, 9 and 12 months post-burn. In post-burn surveys, 30 1 m × 1 m quadrats and 30 2 m × 2 m quadrats were surveyed each in the burned and control areas. It was not expected that the fire would affect the canopy due to the low flame height and burn temperature. Due to the low sample size, the canopy surveys were observational and not statistically analysed. In each 1 m × 1 m quadrat and 2 m × 2 m quadrat, the identity and percentage cover of each species was recorded. The overall vegetation cover was recorded and the cover of each species was used to calculate the percentage of native compared to exotic cover. All surveys were conducted by the same experienced observer (E.D.) and at least one assistant under the supervision of the lead observer. Samples of species that could not be identified in the field were taken to the Macquarie University Herbarium for identification. Twice as many quadrats were surveyed in the baseline and post-herbicide application as a contingency plan for a partially complete burn. Only half of these quadrats were used in our final statistical analysis because only half of the survey area expected to burn actually burned due to wetter than usual conditions and the presence of the low flammability exotic grass species, broad-leaved paspalum. Grasses, herbaceous, and woody species under 0.5 m were surveyed in 1 m × 1 m quadrats, and woody species between 0.5 and 5 m were surveyed in 2 m × 2 m quadrats. Canopy surveys were conducted in seven of the eight 20 m × 20 m plots. The eighth plot was ultimately excluded from the surveys due to having very different vegetation than the other plots.
Pre-burn woody weed herbicide treatment
Four weeks before the burn, target woody weeds were selected for treatment with herbicide by ecological restoration practitioners. All treatment of the woody weeds took place during the winter. Blackberry and madeira vine generally do not produce fruit, flowers, or new growth during winter months (NSW DPI Weedwise, accessed Dec 2024) and so were not fruiting or flowering during treatments. Madeira vine generally does not produce viable seeds in Australia (NSW DPI Weedwise, accessed Dec 2024). Lantana flowers nearly continuously in many parts of eastern Australia due to humid, warm weather (NSW DPI Weedwise, accessed Dec 2024) and so was in flower during herbicide treatment. All individuals of the focus species, lantana, blackberry and madeira vine, within the study area were treated. The average cover for each species was captured in a baseline survey (Table 1). Each plant was treated with herbicide in a targeted approach by cutting the stem at the base and applying herbicide to the cut surface (‘cutting-and-painting’), lightly scraping the stem to reveal the cambium layer and applying herbicide (scraping-and-painting), or by spraying large patches of woody weeds with herbicide (Buchanan 2009). Sprayed plants were treated with herbicide at concentrations 1 L of glyphosate, 25 g of metsulfuron and 100 mL of a wetting agent per 20 L of water. Plants that underwent cutting-and-painting were treated with a mixture of 25 g metsulfuron and 100 mL of a wetting agent per 2 L of undiluted glyphosate. Once treated, the plants were left to dry and contribute to the fuel load. In addition to the fuel load from treated exotic species, native ferns were trampled as a by-product of researchers and restoration professionals moving through the site. These ferns dried and consequently also contributed to the fuel load.
Surface fuel % cover | Near surface fuel % cover | Elevated fuel % cover | |||
---|---|---|---|---|---|
Dead | Dead | Living | Dead | Living | |
12% | 13% | 43% | 35% | 27% |
We followed definitions for fuel type as outlined by Hines et al. (2010).
Low intensity burning and soil temperature data collection
On 18th August 2022, half of the final study reach, approximately 725 m2, underwent a low intensity burn using traditional Indigenous burning techniques (Steffensen 2020). Typically, Cultural burns in the area are applied between autumn and spring depending on the vegetation types being burned and seasonal rainfall patterns. Despite the year being an unusually wet La Niña year, August is typically a drier month and the timing of the burn was determined to be Culturally appropriate. There was no rainfall on the day of the burn and minimal rainfall the preceding week. The morning of the day of the burn was overcast, but sunny by the time the burn was initiated. Wind speed on the day was 2 km/h blowing east (BOM, accessed April 2024). Cues for the right time for burning included the amount of moisture in the ground and the ‘crunching’ sound of the grass intended to be burned. Fires were ignited at points throughout the site and allowed to slowly spread south so that flames were perpendicular to the slope of the riverbank. The fire was kept away from fire-sensitive species within the vegetation community using Indigenous techniques of reading the landscape. The exotic grass broad-leaved paspalum was found to be less flammable than native species during the process and fire starters were used to aid ignition in certain areas. Though, in some areas the grass failed to burn at all. The fire was determined to be a low intensity burn by the presence of white smoke, slow pace of fire spread, flame colour, and relatively low flame height (Thungghutti Natural Regeneration Team, pers. comm. 2024). In the areas that did burn, the primary fuel consisted of elevated fuel comprised of dried lantana and blackberry canes and ferns (Table 1). Surface and near surface fuel consisted of living semi-dried grasses and, to a lesser extent, dried leaf litter. Fuel load estimations were visually assessed in 2 m × 2 m quadrats while conducing pre-burn vegetation surveys. The approximate length and width of the burned area was measured in the field directly post burn.
We collected data about the burn temperature using temperature sensors and tags painted with temperature sensitive paint. Forty temperature sensors (HOBO MX2201) were randomly placed across the half of the study site that was intended to be burned. Sensors were buried at 10 cm depth. Ten centimetres was chosen to ensure that the sensors stayed in place, would not experience direct flame or fire impact, and would not be disturbed by animals or humans for the duration of their deployment. We were not testing sub-surface ecological processes resulting from the burn, so the standard 5 cm was not used. The priority was to characterise temperatures at depth and ensure sensor resilience and stability. As a result, 39 of the 40 deployed sensors survived with only 1 lost to animal burrowing. In other words, no sensors were lost to the fire. The sensors were installed 24 h before the intended burn and removed 2 weeks after to capture both the burn and baseline soil temperatures. In addition to the in-soil sensors, aluminium tags were painted with temperature-sensitive paints and installed at the soil surface using metal irrigation pegs. Tempil® brand Tempilaq paints come in a range of sensitivities that change colour when exposed to different temperatures. Strips were painted on each tag with paints that change colour at 70, 121, 149, 218, 260, and 399°C. Another tag was placed on top of the painted tag and secured with a paper clip so that the paints were not disturbed by soot and char deposits (Iverson et al. 2004). Forty tags were installed directly above the buried temperature sensors, and an additional 160 tags were placed with a 6 m2 spacing in plots 1–4, which was the area expected to be burned.
Data analysis
Data were analysed using Generalised Linear Mixed Models (GLMMs) in R (R Core Team 2022). GLMMs were conducted using the glmmTMB package (Brooks et al. 2017). Overdispersion and normality of residuals were investigated using the DHARMa package (Hartig 2016). Where GLMM models were found significant, post hoc Tukey tests and effect size calculations were conducted using the emmeans package (Lenth et al. 2016). Cover data for total cover of midstorey, groundcover, exotic cover proportion, and exotic vine cover were fitted to GLMM models using beta distribution. Vegetation functional groups cover (shrubs, annual herbs, perennial/biennial herbs, ferns, vines, trees, grasses, non-grass graminoids – hereafter referred to as graminoids) was calculated by summing the percentage cover of each species in a functional group within a plot, and thus, total cover of a functional group sometimes exceeded 100 percent. As a result, we used Tweedie distribution instead of beta distribution to fit GLMMs for vegetation functional groups. Species richness data was analysed first using a Poisson distribution. Where overdispersion was an issue, zero inflation and hurdle models were explored. Shannon–Wiener diversity was also calculated for burned and unburned areas for each survey.
Results
Soil and surface temperatures
Maximum temperatures in burned areas exceeded 399°C at the soil surface and reached 47.1°C at a 10-cm depth (Fig. 2). Average soil temperatures during the burn were 29.1 (s.e. 3.7)°C at 10-cm depth. Sixteen tags recorded a maximum burn temperature of 399°C, 4 tags burned at 260°C, 10 at 149°C, and 10 at 121°C. The remaining tags did not exceed 70°C. Average soil temperature at 10-cm depth in control areas was 12.98 (s.e. 0.15)°C and 10.98 (s.e. 0.004)°C across both burn and control areas over the 24 h before the burn. It took soils approximately 2 days following the burn to return to their baseline temperatures (Fig. 3).
Maximum temperatures recorded (a) at the soil surface using painted tags, and (b) at 10- cm depth using buried temperature sensors. Tags with temperature sensitive paints only record maximum surface temperatures and do not reflect temperature changes over time. Tags can only be used once. Soil sensors display the maximum temperature recorded during burning activities.
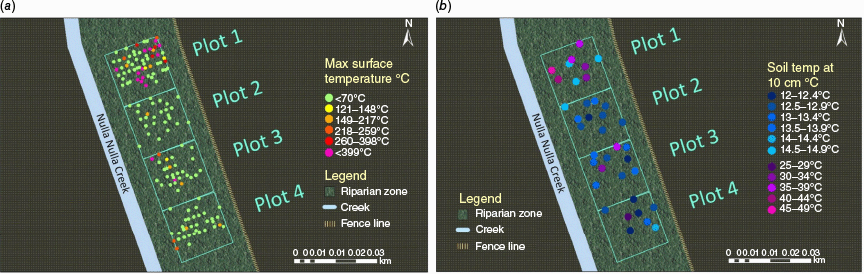
Average with a 95% confidence interval for soil temperature over time at 10-cm depth in burned (B, red line) and unburned (UB, blue line) areas. This data was collected using the buried temperature sensors. Sensors were left in the soil from the 17th August 2022 to the 2nd September 2022 to capture baseline soil temperatures before burning and after burning, respectively. The burn was ignited on 18th August and flames had extinguished by the morning of 19th August, however embers may have continued to burn in some locations.
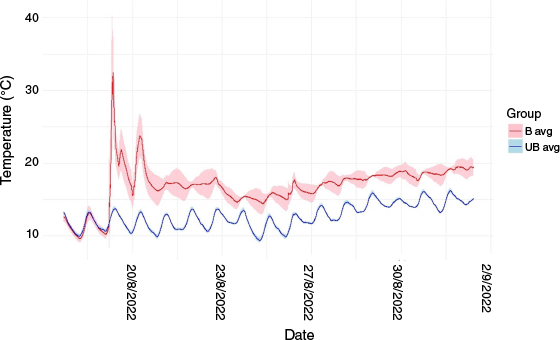
Vegetation
Overall species richness was higher after burning than before, for both native and exotic species. Altogether, we recorded 19 exotic species and 9 native species before burning, and 47 exotic species and 21 native species in burned areas, and 37 exotic species and 18 native species in control areas after treatment. Overall, species diversity for both native and exotic categories increased post-burn (Table 2). Increases in diversity were more pronounced in burned compared to unburned plots.
Baseline | 3-months | 6-months | 9-months | 12-months | ||
---|---|---|---|---|---|---|
Overall | 2.6 | 3.3 | 3.2 | 3.3 | 3.1 | |
Burned | 2.4 | 3.2 | 3.1 | 3.4 | 3.1 | |
Unburned | 2.2 | 2.9 | 3.0 | 2.8 | 2.7 |
The pre-burn midstorey survey was excluded from the calculations because it only recorded woody vegetation over 0.5 m tall and not the characteristics of the whole vegetation community.
There were no significant differences for either native species richness or cover between burned and unburned areas between any of the survey times. Few native woody midstorey species were observed regenerating post-fire. Out of the species recorded post-burn, annual herbs comprised 45% of exotic species and 10% of native species in burned areas, and 49% of exotic species and 6% of native species in control areas post treatment. Prior to treatment, annual herbs only comprised 21% of exotic species and 0% of native species.
Exotic species cover
Exotic species cover was high across all midstorey and groundcover plots, with baseline average percentages of 54% exotic midstory cover and 84% exotic groundcover cover, and 41% exotic midstorey cover post-herbicide treatment (Appendix 1). After burning, average midstorey and groundcover exotic cover in burned areas was 43% and 97%, respectively, and in unburned areas 39% and 90%, respectively. There was no significant difference in proportion of native to exotic groundcover species between burned and unburned quadrats.
Groundcover
Groundcover exotic species richness was significantly different between burned and unburned areas (χ2 = 16.997, P = 0.0019). Exotic species richness was significantly higher in burned compared to unburned areas at the 3-, 9- and 12-month surveys (Fig. 4). Annual herb cover and richness followed a similar pattern to exotic species richness (Fig. 4). Annual herb richness in burned plots at the 3-month survey was significantly higher than the baseline, 6-, 9- and 12-month surveys (Table 3). Herb richness in unburned areas was significantly higher at the 6-month survey than the 3-, 9- and 12-month surveys (Table 3).
(a) Annual herb cover for each of the surveys in burned (B, red) and unburned (UB, blue) areas, and (b) exotics species richness, native species richness, and annual herb species richness for each survey in burned (full line) and unburned (dashed line) areas.
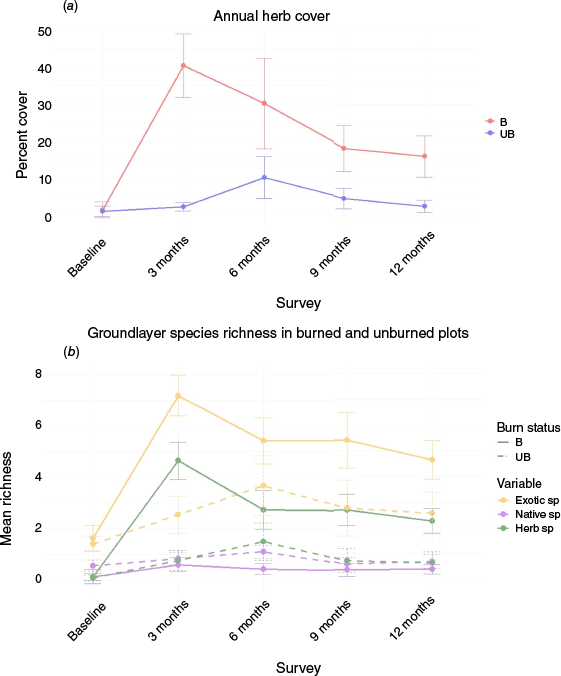
P-value | z-statistic | Effect size ± s.e. | P-value | z-statistic | Effect size ± s.e. | |||
---|---|---|---|---|---|---|---|---|
Exotic species richness | ||||||||
3-month B/UB | <0.01 | 3.9 | 1.1 ± 0.3 | 12-month B/UB | 0.01 | 2.6 | 0.7 ± 0.3 | |
9-month B/UB | 0.006 | 2.8 | 0.7 ± 0.3 | |||||
Annual herb richness | ||||||||
B: 3-month/Baseline | <0.01 | 7.0 | 3.6 ± 0.5 | UB: 6-month/3-month | 0.03 | −2.9 | −0.6 ± 0.3 | |
B: 3-month/6-month | 0.04 | 2.9 | 0.5 ± 0.1 | UB: 6-month/9-month | 0.02 | 3.0 | 0.7 ± 0.3 | |
B: 3-month/9-month | 0.04 | 2.8 | 0.5 ± 0.1 | UB: 12-month/9-month | 0.009 | 3.3 | −0.9 ± 0.3 | |
B: 3-month/12-month | < 0.01 | −4.1 | −0.7 ± 0.2 | |||||
Annual herb cover | ||||||||
3-month B/UB | <0.01 | 5.8 | 5.8 ± 1.0 | B: Baseline/3-month | <0.01 | 7.4 | 6.9 ± 1.0 | |
6-month B/UB | 0.006 | 2.7 | 2.3 ± 0.8 | B: Baseline/6-month | <0.01 | 6.7 | 6.3 ± 1.0 | |
9-month B/UB | 0.0012 | 3.2 | 3.1 ± 1.0 | B: Baseline/9-month | <0.01 | 5.5 | 5.3 ± 1.0 | |
12-month B/UB | <0.01 | 3.9 | 4.1 ± 1.1 | B: Baseline/12-month | <0.01 | 5.2 | 5.0 ± 1.0 | |
UB: 6-month/Baseline | 0.0004 | 4.1 | 4.2 ± 1.0 | B: 3-month/9-month | 0.02 | 2.9 | 1.6 ± 0.5 | |
UB: 6-month/3-month | 0.008 | −3.3 | −3.0 ± 0.9 | B: 3-month/12-month | 0.005 | −3.5 | −1.9 ± 0.5 | |
UB: 6-month/12-month | 0.006 | −3.4 | −3.1 ± 0.9 |
P values, z-statistics, and effect sizes are only reported for tests where significance was found. B, burned areas and UB, unburned areas. For example, ‘UB: 6-month/3-month’ indicates a comparison of outcomes between 6- and 3-month surveys in unburned areas.
There were no significant differences in percent cover of exotic groundcover between surveys, however, overall cover was significantly different between burned and unburned areas (χ2 = 9.90, P = 0.042; Table 1; Fig. 4), and key species showed significant differences post-treatment. The exotic grass broad-leaved paspalum (Paspalum mandiocanum) decreased in cover after burning and stayed low in cover in burned areas 12 months after burning. Paspalum cover was significantly different in burned and unburned areas (χ2 = 12.87, P = 0.012). In burned areas, cover at the baseline survey was significantly higher than the 6-month (z = −3.0, P = 0.03, effect size = −1.1) and 12-month surveys (z = −2.8, P = 0.04; effect size = −1.3)
Annual herb cover was significantly higher in burned compared to unburned areas (χ2 = 19.7, P = 0.0006, Fig. 4). Herb cover in burned and unburned areas was significantly different at all times except the baseline survey (Table 3). In the burned areas, the baseline survey was significantly lower in annual herb cover than all other surveys (Table 3). The 3-month survey had significantly higher cover than the 9- and 12-month surveys in burned areas (Table 3). In unburned areas, the cover at the 6-month survey was significantly higher than the baseline, 3- and 12-month surveys (Table 3).
Midstorey
Percentage midstorey exotic cover decreased after herbicide and burning treatments, and was significantly different between burned and unburned areas (χ2 = 14.09, P = 0.015; Fig. 5). In burned areas percentage exotic midstorey cover decreased after treatments and was significantly higher at the baseline survey than the 3-month (z = −4.63, P < 0.01, effect size = −0.5) and 6-month (z = −2.99, P < 0.033, effect size = −0.3) surveys. In unburned areas, the percentage exotic midstorey cover was significantly lower at the pre-burn survey, after herbicide treatment, than the baseline survey (z = 2.914, P = 0.042, effect size = 0.2) and 9-month survey (z = 3.43, P = 0.008, effect size = 0.2) (Fig. 5). Cover was significantly higher at the 9-month survey than the 12-month survey (z = −3.29, P = 0.013, effect size = −0.4) in the control areas (Fig. 5).
(a) Percentage cover of midstorey exotic species compared to native species cover in burned and unburned areas for each survey, and (b) richness of native and exotic species in burned and unburned areas. B refers to burned areas and UB refers to unburned areas.
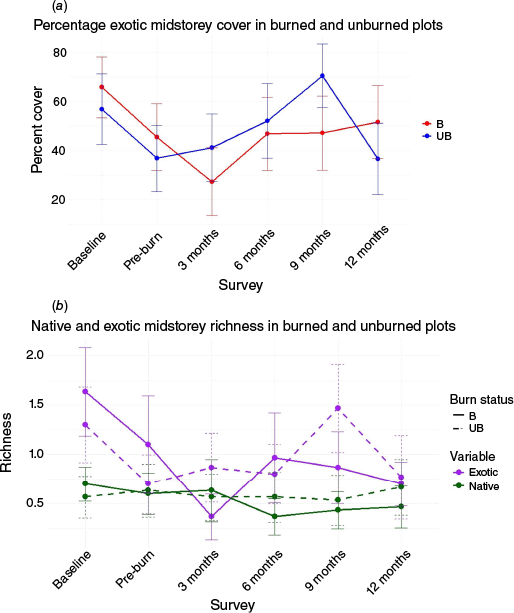
Midstorey exotic species richness, decreased after burning and after spraying herbicide in control areas, and stayed at lower levels in treatment areas after burning (Fig. 5). Midstorey exotic richness increased across all treatments after the 3-month survey. Exotic midstorey species richness was significantly different between burned and unburned areas (χ2 = 11.9, P = 0.036), and lower at the 3-month survey than the baseline survey in burned areas (z = −3.77, P = 0.0023, effect size = −1.5).
Target woody weeds responses to treatments
Decreases in lantana, blackberry and madeira vine cover were observed, both after herbicide treatment and directly post-burn, and cover remained low until 3-months post-burn (Fig. 6). However, there were no significant differences in the target woody weed cover between burned and unburned areas post-treatments. Midstorey cover returned to pre-burn levels for all three woody weeds by the 12-month survey in burned areas and for madeira vine in control areas. Percentage cover of groundcover seedlings for lantana and madeira vine was very low. No groundcover seedling regrowth of blackberry was recorded (Table A1).
Cover for three key woody weeds in the (a) midstorey (plants between 0.5 and 5 m) and (b) groundlayer (plants under 0.5 m) in burned and unburned areas. B refers to burned areas and UB refers to unburned areas.
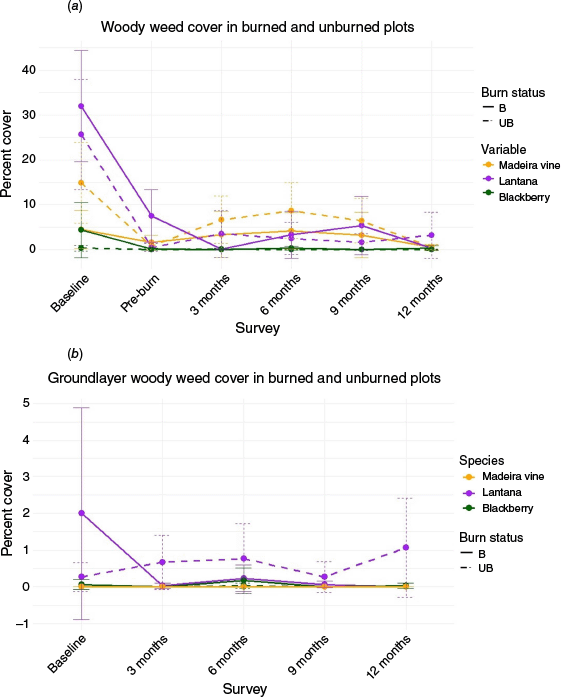
Overall midstorey shrub cover followed a similar pattern to target woody weed cover and decreased after both burning and herbicide treatment. Midstorey shrub cover was significantly different between burned and unburned areas (χ2 = 13.58, P = 0.018; Table 1; Fig. 4) and was lower in unburned areas compared to burned areas at the 6-month survey (z = 2.1, P = 0.03, effect size = 1.0). In burned areas, midstorey shrub cover at the baseline survey was significantly higher than the 3-month (z = −3.8, P = 0.002, effect size = −1.3), 9-month (z = −3.5, P = 0.006, effect size = −1.2), and 12-month (z = −3.8, P = 0.002, effect size = −1.3) surveys. In unburned areas, shrub cover at the baseline survey was significantly higher than the pre-burn (z = 3.5, P = 0.006, effect size = 1.2) and 6-month (z = −4.1, P = 0.0007, effect size = −1.5) surveys (Fig. 2). Groundcover shrub regrowth was also significantly different between burned and unburned areas (χ2 = 19.3, P = 0.0007), and was lower in unburned areas than burned areas at the baseline (z = 2.6, P = 0.008, effect size = 16.8) and 3-month surveys (z = 2.0, P = 0.05, effect size = 9.2). In unburned areas, cover at the 6-month survey was significantly higher than the baseline (z = 3.1, P = 0.008, effect size = 12.6) and 3-month survey (z = −2.7, P = 0.05, effect size = −4.8) (Table A2).
As noted previously, canopy cover results are observational only given that low intensity burns do not penetrate the canopy. However, the burn may have affected the base of vines that are invading the canopy. We observed that overall canopy cover decreased after herbicide and fire treatments in the burned area (Fig. 7). Exotic canopy and vine cover did not decrease in the burn or control areas directly after herbicide treatment but did decrease in the burned area after fire treatment. Both decreased in the unburned area at the 3-month survey. Exotic canopy and vine cover returned to pre-treatment levels in burn and exceeded pre-treatment levels in control areas after 12-months.
Discussion
We found no significant decreases in the cover of lantana, blackberry and madeira vine in plots treated with fire and herbicide, and those treated with herbicide alone. However, we found significant decreases in percentage exotic woody weed cover and richness between the baseline and first post-burn survey at 3-months in burned areas. Therefore, our results support the hypothesis that target woody weed cover is lower in plots treated with fire and herbicide than those only treated with business-as-usual weed control techniques. We also found significant decreases in midstorey shrub cover, of which the three target woody weeds species formed a large component. Both shrub cover and percentage of exotic midstorey species began to increase after the 6-month post-burn survey and by the 12-month survey - there were few differences between the burned and control areas. Our results do not support the hypothesis that native species richness and cover will increase in plots treated with fire more than in those treated with herbicide alone. While midstorey exotic species richness decreased after burning, groundcover exotic species richness increased considerably. Most of this increase was driven by growth of exotic annual species. Few native woody species were observed regenerating after burning.
Our findings suggest that fire and herbicide are more effective at treating woody weeds in a riparian zone than herbicide alone, at least over a relatively short term. Fewer differences between burned and unburned areas were observed after 12 months, suggesting that ongoing management is required to capitalise on the benefit of the initial treatment (Fig. 8). Increases in exotic midstorey cover and richness after the 6-month post-burn survey suggest that ongoing maintenance is needed to curb exotic weed invasion further. Native species richness and cover did not increase after burning, but exotic species richness and cover did. This was driven mainly by an increase in exotic annual herb cover and species richness. Only isolated native species were observed regenerating. Therefore, the risk of creating disturbance and conditions where new invasive species can colonise must be considered when conducting low intensity burns. This suggests that there is a short window within which follow-up treatments are needed to suppress exotic regrowth post-burn. Follow-up treatments are needed within a 3-month window to further control or eliminate invasive woody species. Localised moderate intensity burns may need to be considered to trigger the germination of fire-resistant and pyrogenic native species (Auld and O’Connell 1991). Additionally, a single low intensity burn does not replicate historic, more frequent burn regimes implemented by Indigenous peoples, which remain insufficiently studied in the context of highly modified riparian ecosystems.
Target woody weed, Lantana camara, cover (a) before burning and herbicide treatment, (b) immediately after burning (c) 3 months after burning, and (d) 9 months after burning. Source of photos: E. Duxbury.
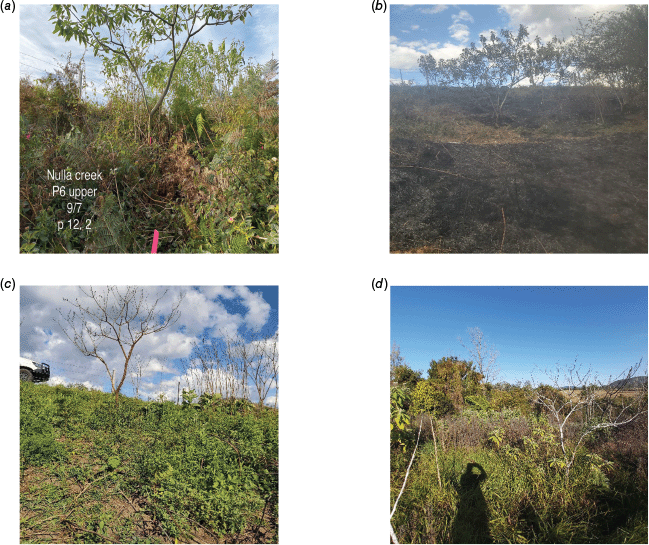
Low intensity burning is not a set-and-forget technique, but it does provide an important window of opportunity for riparian vegetation management to take place. It also holds the potential to be rolled out over much larger areas than common business-as-usual manual or mechanical removal of exotics. Our findings suggest that low intensity burns using traditional techniques could be readily built into riparian vegetation management plans, particularly in novel riparian ecosystems. However, different indicators and additional herbicide application may need to be considered to establish fire timings in systems affected by climate change and where exotic species are present.
We did not find statistically significant decreases in lantana, blackberry or madeira vine cover after burning and herbicide treatments compared to herbicide treatments alone. However, non-significant decreases in the cover of these species after fire and herbicide treatment were pronounced. Much of the lantana and blackberry at our site was eliminated as a result of burning. Unlike past studies, we did not observe significant blackberry or lantana seedling recruitment post-burn, and the cover of standing vegetation remained low for 12 months after the burn. We found that lantana regrowth was the highest 3 months post-burn but very low overall and dropped below pre-burn levels by 12 months post-burn (Fig. 8). Our burn was conducted at a low temperature, so the disturbance it created may not have been significant enough to facilitate lantana invasion. Young seedlings may have been outcompeted by faster-growing grass and annual species (Laterra et al. 2006). Although we found a short-term decrease in lantana and blackberry cover, further studies are needed to understand the effectiveness of using a combination of herbicide treatment and burning on these two target weed species over the long term.
Previous studies have found that burning may not be effective on its own in controlling blackberry (New South Wales Department of Primary Industries (NSW DPI) 2009). Fire kills herbicide-treated canes, but plants can resprout from crowns and roots (Ainsworth and Mahr 2004, 2006). Ainsworth and Mahr (2006) found inconsistent results when examining blackberry regrowth 2 years after the Victorian Black Summer bushfires. Similarly, lantana can resprout from basal dormant buds during low intensity burns (Swarbrick et al. 1998). However, various studies have shown that moderate to high intensity fires are likely to kill adult lantana plants (Swarbrick et al. 1998) and that annual or biennial burning may be enough to eliminate the species in some vegetation communities such as a sub-tropical or dry eucalypt forest (Osunkoya et al. 2013; Debuse and Lewis 2014). In contrast, Raizada and Raghubanshi (2010) found that smoke may increase lantana seedling germination success and reduce seedling mortality and that the disturbance that fire creates can facilitates lantana invasion (Gentle and Duggin 1997). Osunkoya et al. (2013) found that lantana seedling recruitment may negate any loss of standing vegetation caused by infrequent burning. However, they found in simulations that biennial burning over 4–15 years could control the weed (Osunkoya et al. 2013).
Like exotic shrub species, exotic vine cover decreased to its lowest level after burning in the test areas and after herbicide application in the control areas. Post-treatment exotic vine cover peaked during the 6-month post-burn survey during summer and dropped far below pre-burn levels during the 9- and 12-month post-burn surveys. Differences between these surveys are likely due to seasonal differences in leaf cover (Vivian-Smith et al. 2007). Cover of the target weed madeira vine (Anredera cordifolia) peaked during the 6-month post-burn survey (which was in summer), suggesting this species exploited the disturbance created by burning and then either died off in the subsequent months or grew to heights above 5 m so it was not captured in the midstorey survey. We observed canopy regrowth of madeira vine and other exotic vines that exceeded pre-burn levels by the 12-month post-burn survey, so the latter scenario is more likely. We found no previous research on madeira vine’s response to fire. However, this vine reproduces via aerial tubers that drop from vines and become buried in the propagule bank (Vivian-Smith et al. 2007). Anecdotal reports have observed that these tubers resist fire and exploit disturbances created by fire (Thungghutti Natural Regeneration Team, pers. comm. 2024; Urban Bushland Council WA). Unlike this predicted response, we found higher cover of madeira vine propagule recruitment in unburned compared to burned areas, potentially due to relatively moist conditions in these areas that this species prefers (Vivian-Smith et al. 2007).
Our results do not support the hypotheses that low intensity burning in combination with herbicide treatment of woody weeds will increase native plant species richness and cover. On the contrary, we found higher exotic annual herb cover and species richness after burning. Annual herbs often rapidly colonise disturbed sites and have been found in higher abundances at sites that are burned more frequently (Woinarski et al. 2004). Annual herbs exploit disturbance and are short-lived (Keeley et al. 2005). Percentage of annual herb cover peaked 3 months post-burn in the burned area and decreased in subsequent surveys. It remained higher than the pre-treatment survey for 12 months post-burn. It is possible that the seasonality of the winter burn affected germination of native species, however the pyrogenic species blackwood (Acacia melanoxylon) and blady grass (Imperata cylindrica) can germinate in any season under appropriate conditions (Austraits database accessed Dec 2024). Grass cover decreased slightly in burned areas after the fire treatment. Then, it increased in subsequent surveys, except during the 12-month post-burn survey, which was conducted during a drier winter than the previous year and likely resulted in lower leaf cover from drying and leaf death. It is possible that vegetation succession is occurring, and exotic perennial grasses are succeeding annual herb species as they end their life cycles (Cuneo and Leishman 2014; Thomson et al. 2020). Thomson et al. (2020) found that exotic (and native) annual herbs increased substantially after an unplanned fire with the exotics persisting for 5 years. At our site, we observed decreases in annuals cover after 6 months and the return of perennial grasses, which may inhibit the presence of annual species in the long term (Cuneo and Leishman 2014).
We observed perennial grasses growing in burned areas, potentially outcompeting the regrowth of woody weeds. In native grasslands and savannas, exotic shrubs outcompete native grasses when fire regimes involve infrequent burning (Platt et al. 2015). Frequent fire may preference exotic grasses over exotic shrubs (Silvério et al. 2013). At our site, the cover of the exotic grass species broad-leaved paspalum decreased post-burn and was replaced by a mixture of native and exotic grass species. Amongst these species was a locally significant weed, African pigeon grass (Setaria sphacelate) (New South Wales Local Land Services (LLS) 2022) and Weed of National Significance, red natal grass (Melinis repens) (Department of Primary Industries (DPI) 1999). It is possible that the disturbance caused by the low intensity burn provided the opportunity for these species to establish. Thus, the risk of creating conditions, where weeds that pose greater biodiversity risks than pre-burn species assemblages can establish, must be considered when using fire for vegetation management in highly modified ecosystems (Graham and Taylor 2018).
Very little native species regrowth was observed post-burn aside from ferns that provided the initial fuel load. It was isolated to a few acacia seedlings, a small amount of blady grass (Imperata cylindrica), and a flush of the native annual Sigisbekia orientalis. The acacia seedlings were too small to be identified but were likely the local species blackwood (Acacia melanoxylon) because adult individuals were present at the site. The low overall return of native vegetation could be due to a few factors. Firstly, the river reach had been unfenced and accessible to cattle for many years before it began recovering (landholder, pers. comm.) and there was a much greater quantity of exotic standing vegetation than native standing vegetation, so it is possible that exotic propagules dominate the seedbank. For example, at our site only a few (2–3) individual acacias were observed regenerating in the months after the burn. It is possible that more acacia regeneration was not observed because there were only two parent trees in the burn area, and both were relatively small adult trees, meaning there may have been limited acacia seeds in the propagule bank. Research on riparian seedbanks in the Hunter Valley, which is further south, supports this assumption. There, O’Donnell et al. (2016) found that river reaches in low to moderate geomorphic condition had a high proportion of exotic species propagules in the seedbank, largely dominated by exotic grasses and annuals. Additionally, herbicide residue in the soil may have affected native species germination and seedling establishment (Cornish and Burgin 2005; Fos et al. 2024). In certain greenhouse experiments, glyphosate residue in soils has been found to inhibit seed germination and native seedling establishment, and native species may have been more sensitive to herbicides that exotic species at the study site (Cornish and Burgin 2005; Fos et al. 2024). However, these effects are most prevalent in low absorption soils that were not present at our study site (Cornish and Burgin 2005). Other field-based experiments have found that woody weed cover reduction through herbicide application increases native species presence, particularly when exotic species biomass is removed (Carreiro et al. 2020; Jucker et al. 2020).
The Eastern Riverine Forest is thought to have historically covered the riparian corridor along Nulla Nulla Creek containing certain fire-promoted species (SEED, accessed April 2024). While very little intact cover of this vegetation community exists, components of this community do remain and it is possible that a few fire-promoted species may occur in the seed bank (SEED; Macleay Valley planting guide; Thungghutti Natural Regeneration Team, pers. comm. 2024). Very little Western scientific information is available to advise optimal fire regimes for this vegetation community, however burning at intervals between 7 and 35 years has been suggested (Kenny et al. 2003). Historically, campsite locations at the junction of Nulla Nulla Creek and the Macleay River were burned at up to an annual frequency (Thungghutti Natural Regeneration Team, pers. comm. 2024). However, burn frequency varied depending on weather conditions and the type and amount of vegetation present. Some of the mature woody native vegetation on-site were species associated with the Eastern Riverine Forest. The Cultural burning practice used at this site kept flames away from sensitive species.
Many rainforest communities are considered fire-sensitive and too frequent or hot burns can prevent these communities from regenerating (Kenny et al. 2003; Le Breton et al. 2020). However, there is very little available information about the response to fire for individual rainforest-adapted species present in the Eastern Riverine Forest community. Lilli pilli (Acmena smithii) has some ability to resprout after fire, but its seeds are sensitive to desiccation and unlikely to survive fire (Ashton and Frankenberg 1976). Other species that Eastern Riverine Forest comprises are pyrogenic or fire resistant, including forest red gum (Eucalyptus tereticornis), blady grass (Imperata cylindrica) and blackwood (Acacia melanoxylon), of which the latter two species were present at the study site (Lewis et al. 2012; Cruz et al. 2017; Butler et al. 2021). Additionally, Thungghutti Cultural burning practitioners report eucalyptus and Acacia spp. regenerating after hotter riparian burns (Thungghutti Natural Regeneration Team, pers. comm. 2024).
In our study, the degree of heat transfer to the soil may not have created favourable conditions for the germination of native seeds. Germination of the local species, blackwood (Acacia melanoxylon), is triggered by moderately hot burn temperatures of approximately 80°C (Cruz et al. 2017). Soil temperatures at 10-cm depth reached a maximum of 47.1°C and surface temperatures exceeded 399°C. Therefore, it is possible that seeds in the upper 10 cm of soil achieved temperatures of 80°C in some areas and remained at elevated temperatures for at least 3 h. However, high soil heating at 10-cm depth suggests that the top 2–3 cm would have reached temperatures lethal to seeds (Tangney et al. 2020). Additionally, it is difficult to accurately infer soil temperatures at soil depths above 10 cm because temperatures during fires become decoupled from soil heat transfer except under extreme heating or prolonged heat radiation (Tangneny et al. 2020). Even if germination was triggered by fire, there may not have been suitable temperature or rainfall patterns for young seedlings to survive (Walters et al. 2004), or they may have been outcompeted by fast-growing annuals (Woinarski et al. 2004).
The native species blady grass (Imperata cylindrica) is pyrogenic and relies on fire to spread. Small stands of blady grass were present at the site. This species reproduces through rhizome fragments after fire and by seed (Holm 1977). Seed typically spreads 15 m from mature plants or further (MacDonald 2004). However, despite being a fire-loving species, we did not observe a substantial increase in blady grass cover after burning. This differs from studies outside of Australia where blady grass is a significant invasive species, known to outcompete native species and alter fire regimes (Holzmueller and Jose 2012; Butler et al. 2021). Further investigation into the composition of the seedbank in this region is needed to understand what capacity highly modified sites have for native species regeneration after fire, and what their soil and surface temperature thresholds are for triggering gemination. Further work is also needed on the conditions under which fire may produce mortality of weed and invasive species. To improve the biodiversity conditions at this site, and many other sites like it, it is likely that interventions, such as direct seeding or planting and further weed management are needed in the post-fire window of opportunity (Cuneo and Leishman 2014). Increasing native species presence can allow them to outcompete exotic propagules stored in the seed bank (Ainsworth and Mahr 2006).
Temperatures from our burn were similar to temperatures recorded for similar prescribed burns. Iverson et al. (2004) observed soil surface temperatures of 117°C on average and 381°C maximum during a prescribed burn. Granged et al. (2011) found that soil surface temperatures ranged between 142, 317, and 525°C for low, moderate, and high intensity burns, respectively. We aimed to conduct a low intensity, burn, however, some surface burn temperatures at our site exceeded 399°C, despite being conducted in a wet riparian environment. One soil sensor reached 47°C at 10-cm depth. It is likely that this sensor reached a high temperature because it was buried under a particularly large fuel load of dried lantana canes. Past research in banksia woodland found that mean soil temperatures during burns ranged between 20 and 30°C at 10-cm depth across areas where fine fuel loads had been manipulated to between 8 and 20 t/ha (Tangney et al. 2020). Penman and Towerton (2008) found that during ecological burning in a dry sclerophyll forest most of their sensors at 5-cm depth recorded temperatures below 40°C and only one sensor at 2-cm depth recorded a temperature above 100°C. They noted that the low temperatures recorded may be too low to trigger germination in fire-promoted species. However, further trials with sensors in the upper 5 cm of soil will be needed to determine whether similar temperatures are reached in highly modified riparian systems. Finally, it needs to be noted that fire-promoted species respond to a range of cues, not just heat (Thomas et al. 2003). This study did not investigate soil moisture or smoke-chemical responses to fire, or combinations of cues (e.g. Brown and Van Staden 1997; Thomas et al. 2003).
Conclusions
Our study found that a combination of low intensity burning and herbicide treatment may be effective in reducing woody weed cover in riparian sites in the short term. We have quantified the temperature of a low intensity burn conducted in a moist riparian environment. Creating dry biomass through treated woody weeds was necessary for creating the conditions where a burn could take place due to wet conditions, exacerbated by a La Niña year. The presence of the low-flammability exotic species broad-leaved paspalum further impeded the burn. One advantage of using fire is that it can be applied across a much larger area and more quickly than manual vegetation treatments and can be used in combination with other techniques such as herbicide application. However, it is unlikely that a single treatment with fire and herbicide will be enough to eliminate woody weeds in novel riparian ecosystems in the long-term. Our study found that few native species regenerated after burning, and woody weeds began to return after a 3-month post-burn window. Additionally, burning creates a disturbance that may be exploited by species that pose biodiversity risks. Therefore, integrated long-term interventions combining reduction of weedy biomass with native species restoration initiated within a 3-month window will be essential for any management project utilising fire in highly modified riparian systems. Further interventions could include trialling repeated burns at different temperatures, replicating historic Indigenous burn regimes. Native biodiversity could also be improved by direct seeding, tube stock planting, and follow up burning and herbicide treatments to reduce weedy biomass. Community-led projects utilising local expertise in low intensity burning including Cultural burning techniques hold significant promise as a way to implement integrated rehabilitation plans on-the-ground.
Data availability
The data that support the findings of this study are available from the corresponding author upon reasonable request.
Declaration of Funding
This research was funded by Australian Research Council Linkage grant (LP190100314) led by Kirstie Fryirs with industry partners Landcare Australia and Hunter-Central Rivers Local Land Services. Elisha Duxbury is supported by a project-funded Macquarie University Research Excellence Scholarship (MQRES) and Higher Degree Research funds.
Acknowledgements
We thank Danelle Agnew, Nuosha Zhang, our numerous volunteers, Michael Fyffe, and North Coast Local Land Services for help with fieldwork. We thank Kenneth Major for advice on low intensity burning and Cultural knowledge. We thank Karen Marais at Macquarie University for help with plant identification. We thank two anonymous reviewers for their comments on this paper. We acknowledge the Traditional Custodians of the land and rivers upon which this research was carried out, the Thungghutti Nation, and pay our respects to their elders past and present.
References
Ashton DH, Frankenberg J (1976) Ecological studies of Acmena smithii (Poir.) Merrill and Perry with special reference to Wilson’s Promontor. Australian Journal of Botany 24(4), 453-487.
| Crossref | Google Scholar |
Auld T, O’Connell M (1991) Predicting patterns of post‐fire germination in 35 eastern Australian Fabaceae. Australian Journal of Ecology 16(1), 53-70.
| Crossref | Google Scholar |
Bebawi FF, Campbell SD (2000) Effects of fire on germination and viability of rubber vine Cryptostegia grandiflora seeds. Australian Journal of Experimental Agriculture 40(7), 949-957.
| Crossref | Google Scholar |
Bebawi FF, Campbell SD (2002) Impact of early and late dry-season fires on plant mortality and seed banks within riparian and subriparian infestations of rubber vine Cryptostegia grandiflora. Australian Journal of Experimental Agriculture 42(1), 43-48.
| Crossref | Google Scholar |
BoM (Bureau of Meteorology) (2024) Climate Data Online. Australian Government. Retrieved from http://www.bom.gov.au/climate/data [April 2024]
Bixby RB, Cooper SD, Gresswell RD, Brown LE, Dahm CN, Dwire KA (2015) Fire effects on aquatic ecosystems: an assessment of the current state of science. Freshwater Science 34, 1340-1350.
| Crossref | Google Scholar |
Bradstock RA, Auld TD (1995) Soil temperatures during experimental bushfires in relation to fire intensity: consequences for legume germination and fire management in south-eastern Australia. Journal of Applied Ecology 32(1), 76-84.
| Crossref | Google Scholar |
Brancatelli GIE, Yezzi AL, Zalba SM (2024) Fire as a management tool for invasive woody plants in natural environments: a systematic review. Biological Conservation 293, 110602.
| Crossref | Google Scholar |
Brooks ML, D’Antonio CM, Richardson DM, Grace JB, Keeley JE, DiTomaso JM, Hobbs RJ, Pellant M, Pyke D (2004) Effects of invasive alien plants on fire regimes. BioScience 54(7), 677-688.
| Crossref | Google Scholar |
Brooks ME, Kristensen K, van Benthem KJ, Magnusson A, Berg CW, Nielsen A, Skaug HJ, Maechler M, Bolker BM (2017) glmmTMB balances speed and flexibility among packages for zero-inflated generalized linear mixed modeling. The R Journal 9, 378-400.
| Crossref | Google Scholar |
Brown NAC, Van Staden J (1997) Smoke as a germination cue: a review. Plant Growth Regulation 22(2), 115-124.
| Crossref | Google Scholar |
Brown AG, Tooth S, Bullard JE, Thomas DSG, Chiverrell RC, Plater AJ, Murton J, Thorndycraft VR, Tarolli P, Rose J, Wainwright J, Downs P, Aalto R (2017) The geomorphology of the Anthropocene: emergence, status and implications. Earth Surface Processes and Landforms 42(1), 71-90.
| Crossref | Google Scholar |
Butler OM, Lewis T, Chen C (2021) Do soil chemical changes contribute to the dominance of blady grass (Imperata cylindrica) in surface fire-affected forests? Fire 4(2), 23.
| Crossref | Google Scholar |
Carreiro MM, Fuselier LC, Waltman M (2020) Efficacy and nontarget effects of glyphosate and two organic herbicides for invasive Woody Vine Control. Natural Areas Journal 40(2), 129-141.
| Crossref | Google Scholar |
Catford JA, Jansson R (2014) Drowned, buried and carried away: effects of plant traits on the distribution of native and alien species in riparian ecosystems. The New Phytologist 204(1), 19-36.
| Crossref | Google Scholar | PubMed |
Cohen T, Suesse T, Reinfelds I, Zhang N, Fryirs K, Chisholm L (2022) The re-greening of east coast Australian rivers: an unprecedented riparian transformation. Science of The Total Environment 810, 151309.
| Crossref | Google Scholar | PubMed |
Corenblit D, Davies NS, Steiger J, Gibling MR, Bornette G (2014) Considering river structure and stability in the light of evolution: feedbacks between riparian vegetation and hydrogeomorphology. Earth Surface Processes and Landforms 40(2), 189-207.
| Crossref | Google Scholar |
Cornish PS, Burgin S (2005) Residual effects of glyphosate herbicide in ecological restoration. Restoration Ecology 13(4), 695-702.
| Crossref | Google Scholar |
Cruz O, García-Duro J, Casal M, Reyes O (2017) Can the mother plant age of Acacia melanoxylon (Leguminosae) modulate the germinative response to fire? Australian Journal of Botany 65(6, 7), 593-600.
| Crossref | Google Scholar |
Cuneo P, Leishman MR (2014) Recovery after African Olive invasion: can a ‘bottom-up’ approach to ecological restoration work? Ecological Management and Restoration 16(1), 33-40.
| Crossref | Google Scholar |
Debuse VJ, Lewis T (2014) Long-term repeated burning reduces Lantana camara regeneration in a dry eucalypt forest. Biological Invasions 16(12), 2697-2711.
| Crossref | Google Scholar |
Douglas MM, Setterfield SA, McGuinness K, Lake PS (2015) The impact of fire on riparian vegetation in Australia’s tropical savanna. Freshwater Science 34(4), 1351-1365.
| Crossref | Google Scholar |
Duxbury E, Fryirs K, Leishman MR (2024) Benchmarking riparian vegetation quality in recovering rivers: implications for management of novel ecosystems. Environmental Management 75, 221-239.
| Crossref | Google Scholar | PubMed |
Enright NJ, Fontaine JB, Bowman DMJS, Bradstock RA, Williams RJ (2015) Interval squeeze: altered fire regimes and demographic responses interact to threaten woody species persistence as climate changes. Frontiers in Ecology and the Environment 13(5), 265-272.
| Crossref | Google Scholar |
Firesticks Alliance Indigenous Corporation (2019) What is cultural burning? Available at http://www.firesticks.org.au/about/cultural-burning/ [accessed 25 January 25]
Fos M, Esteban D, Sanchis E (2024) effect of glyphosate on germination and seedling development of four native plants of dunes in Spain. European Journal of Environmental Sciences 14(1), 43-48.
| Crossref | Google Scholar |
Franklin DC, Prior LD, Hogarth NJ, McMahon CR (2009) Bamboo, fire and flood: consequences of disturbance for the vegetative growth of a clumping, clonal plant. Plant Ecology 208(2), 319-332.
| Crossref | Google Scholar |
Fryirs KA, Brierley GJ (2016) Assessing the geomorphic recovery potential of rivers: forecasting future trajectories of adjustment for use in management. Wiley Interdisciplinary Reviews Water 3(5), 727-748.
| Crossref | Google Scholar |
Fryirs K, Chessman B, Rutherfurd I (2013) Progress, problems and prospects in Australian river repair. Marine and Freshwater Research 64(7), 642-654.
| Crossref | Google Scholar |
Fryirs KA, Brierley GJ, Hancock F, Cohen TJ, Brooks AP, Reinfelds I, Cook N, Raine A (2018) Tracking geomorphic recovery in process-based river management. Land Degradation and Development 29(9), 3221-3244.
| Crossref | Google Scholar |
Fryirs KA, Zhang N, Duxbury E, Ralph T (2022) Rivers up in smoke: Impacts of Australia’s 2019-2020 megafires on riparian systems. International Journal of Wildland Fire 31(7), 720-727.
| Crossref | Google Scholar |
Gentle CB, Duggin JA (1997) Interference of Choricarpia leptopetala by Lantana camara with nutrient enrichment in mesic forests on the Central Coast of NSW. Plant Ecology 136(2), 205-211.
| Crossref | Google Scholar |
Gott B (2005) Aboriginal fire management in south-eastern Australia: aims and frequency. Journal of Biogeography 32(7), 1203-1208.
| Crossref | Google Scholar |
Graham M, Taylor K (2018) Fire, Weeds and the Native Vegetation of New South Wales. Hotspots Fire. Available at https://hotspotsfireproject.org.au/download/fire-weeds-and-native-vegetation-of-nsw.pdf
Granged AJP, Jordán A, Zavala LM, Muñoz-Rojas M, Mataix-Solera J (2011) Short-term effects of experimental fire for a soil under eucalyptus forest (SE Australia). Geoderma 167-168, 125-134.
| Crossref | Google Scholar |
Hankins DL (2013) The effects of indigenous prescribed fire on riparian vegetation in central California. Ecological Processes 2(1), 24.
| Crossref | Google Scholar |
Hartig F (2016) ‘DHARMa: residual diagnostics for hierarchical (multi-level/mixed) regression models.’ R package version 0.1. 0. Available at https://cran.r-project.org/web/packages/DHARMa/vignettes/DHARMa.html
Hobbs RJ, Arico S, Aronson J, Baron JS, Bridgewater P, Cramer VA, Epstein PR, Ewel JJ, Klink CA, Lugo AE, Norton D, Ojima D, Richardson DM, Sanderson EW, Valladares F, Vilà M, Zamora R, Zobel M (2006) Novel ecosystems: theoretical and management aspects of the new ecological world order. Global Ecology and Biogeography 15(1), 1-7.
| Crossref | Google Scholar |
Holzmueller EJ, Jose S (2012) Response of the invasive grass Imperata cylindrica to disturbance in the southeastern forests, USA. Forests 3(4), 853-863.
| Crossref | Google Scholar |
Iverson LR, Yaussy DA, Rebbeck J, Hutchinson TF, Long RP, Prasad AM (2004) A comparison of thermocouples and temperature paints to monitor spatial and temporal characteristics of landscape-scale prescribed fires. International Journal of Wildland Fire 13(3), 311.
| Crossref | Google Scholar |
Jucker T, Long V, Pozzari D, Pedersen D, Fitzpatrick B, Yeoh PB, Webber BL (2020) Developing effective management solutions for controlling stinking passionflower (Passiflora foetida) and promoting the recovery of native biodiversity in Northern Australia. Biological Invasions 22(9), 2737-2748.
| Crossref | Google Scholar |
Keeley JE, Baer-Keeley M, Fotheringham CJ (2005) Alien plant dynamics following fire in mediterranean-climate California shrublands. Ecological Applications 15(6), 2109-2125.
| Crossref | Google Scholar |
Laterra P, Ortega EZ, Ochoa MDC, Vignolio OR, Fernández ON (2006) Interactive influences of fire intensity and vertical distribution of seed banks on post-fire recolonization of a tall-tussock grassland in Argentina. Austral Ecology 31(5), 608-622.
| Crossref | Google Scholar |
Le Breton TD, Natale S, French K, Gooden B, Ooi MKJ (2020) Fire-adapted traits of threatened shrub species in riparian refugia: implications for fire regime management. Plant Ecology 221(1), 69-81.
| Crossref | Google Scholar |
Lenth RV, Bolker B, Buerkner P, Giné-Vázquez I, Herve M, Jung M, Love J, Miguez F, Riebl H, Singmann H (2016) ‘Estimated Marginal Means, aka Least-Squares Means.’ (R CRAN) Available at https://github.com/rvlenth/emmeans
Lewis T, Taylor D, Swift S, Debuse V (2012) Factors influencing early restoration progress of a Eucalyptus tereticornis open forest on former agricultural land. Pacific Conservation Biology 18(4), 263-281.
| Crossref | Google Scholar |
Mabbott R, Fryirs K (2022) Geomorphic and vegetative river recovery in a small coastal catchment of New South Wales, Australia: implications for flow hydrology and river management. Geomorphology 413, 108334.
| Crossref | Google Scholar |
MacDonald GE (2004) Cogon grass (Imperata cylindrica) - biology, ecology, and management. Critical Reviews in Plant Sciences 23(5), 367-380.
| Crossref | Google Scholar |
Mandle L, Bufford JL, Schmidt IB, Daehler CC (2011) Woody exotic plant invasions and fire: reciprocal impacts and consequences for native ecosystems. Biological Invasions 13(8), 1815-1827.
| Crossref | Google Scholar |
McDonald T, Williams J (2009) A perspective on the evolving science and practice of ecological restoration in Australia. Ecological Management and Restoration 10(2), 113-125.
| Crossref | Google Scholar |
New South Wales Department of Climate Change, Energy, the Environment and Water (NSW DCCEEW) (2010) NPWS Fire History - Wildfires and Prescribed Burns, accessed from The Sharing and Enabling Environmental Data (SEED) Portal. Available at https://datasets.seed.nsw.gov.au/dataset/fire-history-wildfires-and-prescribed-burns-1e8b6 [accessed 4 December 2024]
New South Wales Department of Primary Industries (NSW DPI) ‘NSW WeedWise.’ Blackberry (Rubus fruticosus species aggregate) (NSW Goverment) Reviewed 2025. Available at https://weeds.dpi.nsw.gov.au/Weeds/Details/18
New South Wales Department of Primary Industries (NSW DPI) ‘NSW WeedWise.’ Madeira vine (Anredera cordifolia) (NSW Goverment) Reviewed 2025. Available at https://weeds.dpi.nsw.gov.au/Weeds/Details/83
New South Wales Department of the Environment (NSW DPE) (2020) River styles in NSW. A consistent method to characterise the types of rivers. Available at https://water.dpie.nsw.gov.au/science-data-and-modelling/surface-water/monitoring-changes/river-styles-in-nsw
New South Wales Local Land Services (LLS) (2022) North Coast Regional Strategic Weed Management Plan 2023-2027. Available at https://www.lls.nsw.gov.au/help-and-advice/weeds-and-plant-diseases/regional-strategic-weed-management-plans
O’Donnell J, Fryirs K, Leishman MR (2015) Can the regeneration of vegetation from riparian seed banks support biogeomorphic succession and the geomorphic recovery of degraded river channels? River Research and Applications 31(7), 834-846.
| Crossref | Google Scholar |
O’Donnell J, Fryirs KA, Leishman MR (2016) Seed banks as a source of vegetation regeneration to support the recovery of degraded rivers: a comparison of river reaches of varying condition. Science of The Total Environment 542, 591-602.
| Crossref | Google Scholar | PubMed |
Osunkoya OO, Perrett C, Fernando C, Clark C, Raghu S (2013) Modeling population growth and site specific control of the invasive Lantana camara L. (Verbenaceae) under differing fire regimes. Population Ecology 55(2), 291-303.
| Crossref | Google Scholar |
Penman TD, Towerton AL (2008) Soil temperatures during autumn prescribed burning: implications for the germination of fire responsive species? International Journal of Wildland Fire 17(5), 572-578.
| Crossref | Google Scholar |
Petty AM, Douglas MM (2009) Scale relationships and linkages between woody vegetation communities along a large tropical floodplain river, north Australia. Journal of Tropical Ecology 26(1), 79-92.
| Crossref | Google Scholar |
Pettit NE, Naiman RJ (2007) Fire in the Riparian Zone: characteristics and ecological consequences. Ecosystems 10(5), 673-687.
| Crossref | Google Scholar |
Platt WJ, Entrup AK, Babl EK, Coryell-Turpin C, Dao V, Hebert JA, Labarbera CD, Noto JFL, Ogundare SO, Stamper LK, Timilsina N (2015) Short-term effects of herbicides and a prescribed fire on restoration of a shrub-encroached pine savanna. Restoration Ecology 23(6), 909-917.
| Crossref | Google Scholar |
Radford IJ, Grice AC, Abbott BN, Nicholas DM, andWhiteman L (2008) Impacts of changed fire regimes on tropical riparian vegetation invaded by an exotic vine. Austral Ecology 33(2), 151-167.
| Crossref | Google Scholar |
Raizada P, Raghubanshi AS (2010) Seed germination behaviour of Lantana camara in response to smoke. Tropical Ecology 51(2), 347-352.
| Google Scholar |
Richardson DM, Holmes PM, Esler KJ, Galatowitsch SM, Stromberg JC, Kirkman SP, Pyšek P, Hobbs RJ (2007) Riparian vegetation: degradation, alien plant invasions, and restoration prospects. Diversity and Distributions 13(1), 126-139.
| Crossref | Google Scholar |
Ross T, Srivastava SK, Shapcott A (2023) Investigating the relationship between fire severity and post-fire vegetation regeneration and subsequent fire vulnerability. Forests 14(2), 222.
| Crossref | Google Scholar |
SEED (Sharing and Enabling Environmental Data Portal). NSW 1500K Simplified Surface Geology. Retrieved from https://datasets.seed.nsw.gov.au/dataset/nsw-1500k-simplified-surface-geology [accessed April 2024]
SEED (Sharing and Enabling Environmental Data Portal). NSW State Vegetation Type Map. Retrieved from https://datasets.seed.nsw.gov.au/dataset/nsw-state-vegetation-type-map [accessed April 2024]
Silvério DV, Brando PM, Balch JK, Putz FE, Nepstad DC, Oliveira-Santos C, Bustamante MMC (2013) Testing the Amazon savannization hypothesis: Fire effects on invasion of a neotropical forest by native cerrado and exotic pasture grasses. Philosophical Transactions of the Royal Society B: Biological Sciences 368(1619), 20120427.
| Crossref | Google Scholar | PubMed |
Tangney R, Merritt DJ, Callow JN, Fontaine JB, Miller BP (2020) Seed traits determine species' responses to fire under varying soil heating scenarios. Functional Ecology 34(9), 1967-1978.
| Crossref | Google Scholar |
Thomas PB, Morris EC, Auld TD (2003) Interactive effects of heat shock and smoke on germination of nine species forming soil seed banks within the Sydney region. Austral Ecology 28(6), 674-683.
| Crossref | Google Scholar |
Thomson DM, Bonapart AD, King RA, Schultz EL, Startin CR (2020) Long-term monitoring of a highly invaded annual grassland community through drought, before and after an unintentional fire. Journal of Vegetation Science 31(2), 307-318.
| Crossref | Google Scholar |
Underwood AJ (1991) Beyond baci: experimental designs for detecting human environmental impacts on temporal variations in natural populations. Marine and Freshwater Research 42(5), 569-587.
| Crossref | Google Scholar |
Vivian-Smith G, Lawson BE, Turnbull I, Downey PO (2007) The biology of Australian weeds. 46. Anredera cordifolia (Ten.) Steenis. Plant Protection Quarterly 22(1), 2-10 Available at https://www.scopus.com/inward/record.uri?eid=2-s2.0-33947420036andpartnerID=40andmd5=ed5774208e1650bce22bd0ebe7013e2f.
| Google Scholar |
Walters M, Midgley JJ, Somers MJ (2004) Effects of fire and fire intensity on the germination and establishment of Acacia karroo, Acacia nilotica, Acacia luederitzii and Dichrostachys cinerea in the field. BMC Ecology 4, 3.
| Crossref | Google Scholar | PubMed |
Wohl E (2019) Forgotten legacies: understanding and mitigating historical human alterations of river corridors. Water Resources Research 55(7), 5181-5201.
| Crossref | Google Scholar |
Woinarski JCZ, Risler J, Kean L (2004) Response of vegetation and vertebrate fauna to 23 years of fire exclusion in a tropical Eucalyptus open forest, Northern Territory, Australia. Austral Ecology 29(2), 156-176.
| Crossref | Google Scholar |
Yeates AG, Schooler SS (2011) Influence of Lantana camara and its removal on tree dynamics in a recently burned wet Sclerophyll forest in Northern NSW. Ecological Management and Restoration 12(3), 236-241.
| Crossref | Google Scholar |
Yibarbuk D, Whitehead PJ, Russell-Smith J, Jackson D, Godjuwa C, Fisher A, Cooke P, Choquenot D, Bowman DMJS (2001) Fire ecology and Aboriginal land management in central Arnhem Land, northern Australia: a tradition of ecosystem management. Journal of Biogeography 28(3), 325-343.
| Crossref | Google Scholar |
Zhang N, Fryirs K (2023) Trends in post-1950 riparian vegetation recovery in coastal catchments of NSW Australia: implications for remote sensing analysis, forecasting and river management. Earth Surface Processes and Landforms 48(11), 2152-2170.
| Crossref | Google Scholar |
Appendix 1.Percentage cover of vegetation pre- and post-treatment and site species list.
Burned | Unburned | ||||||||||||||||
---|---|---|---|---|---|---|---|---|---|---|---|---|---|---|---|---|---|
Baseline | Pre-burn | 3 months | 12 months | Baseline | Pre-burn | 3 months | 12 months | ||||||||||
Midstorey | Mean | s.e. | Mean | s.e. | Mean | s.e. | Mean | s.e. | Mean | s.e. | Mean | s.e. | Mean | s.e. | Mean | s.e. | |
Total cover | 64% | 6% | 27% | 5% | 21% | 6% | 23% | 5% | 59% | 7% | 28% | 6% | 36% | 7% | 35% | 7% | |
Proportion exotic cover | 66% | 7% | 45% | 8% | 27% | 8% | 52% | 9% | 57% | 8% | 37% | 8% | 41% | 8% | 37% | 9% | |
Shrub cover | 59% | 10% | 20% | 5% | 14% | 6% | 12% | 5% | 54% | 9% | 10% | 4% | 16% | 5% | 23% | 8% | |
Exotic species richness | 1.6 | 0.2 | 4 | 0.3 | 0.4 | 0.1 | 0.7 | 0.1 | 1.3 | 0.2 | 2.5 | 0.2 | 0.9 | 0.2 | 0.8 | 0.2 | |
Madeira vine | 3% | 2% | 1.4% | 0.6% | 0.1% | 0.1% | 0.4% | 0.3% | 14% | 5% | 5.0% | 1.2% | 5% | 3% | 0.5% | 0.4% | |
Lantana | 10% | 5% | 2.7% | 1.0% | 0.1% | 0.1% | 0.4% | 0.3% | 8% | 4% | 1.6% | 0.7 | 0.2% | 0.2% | 0.3% | 0.3% | |
Blackberry | 3% | 3% | 0.7% | 0.6% | 0% | 0% | 0.05% | 0.04% | 0% | 0% | 0% | 0% | 0% | 0% | 0% | 0% | |
Groundcover | Mean | s.e. | Mean | s.e. | Mean | s.e. | Mean | s.e. | NA | NA | Mean | s.e. | Mean | s.e. | |||
Total cover | 73% | 7% | NA | NA | 83% | 4% | 89% | 3% | 86% | 6% | NA | NA | 67% | 7% | 69% | 6% | |
Total species richness | 2 | 0.3 | NA | NA | 8.3 | 0.5 | 5.3 | 0.5 | 2 | 0.2 | NA | NA | 3.4 | 0.4 | 3.3 | 0.4 | |
Exotic species richness | 1.6 | 0.2 | NA | NA | 7.2 | 0.4 | 4.6 | 0.4 | 1.4 | 0.1 | NA | NA | 2.5 | 0.3 | 2.6 | 0.4 | |
Annual herb cover | 1% | 1% | NA | NA | 40% | 5% | 16% | 3% | 1% | 0.8% | NA | NA | 2% | 0.6% | 2% | 0.1% | |
Broad-leaved paspalum | 33% | 8% | NA | NA | 17% | 6% | 3% | 3% | 13% | 6% | NA | NA | 9% | 5% | 6% | 4% | |
Madeira vine | 2% | 1% | NA | NA | 0.03% | 0.03% | 0% | 0% | 0.3% | 0.2% | NA | NA | 0.7% | 0.4% | 1% | 0.7% | |
Lantana | 0.07% | 0.07% | NA | NA | 0% | 0% | 0.03% | 0.03% | 0% | 0% | NA | NA | 0% | 0% | 0% | 0% | |
Bare ground | 8% | 5% | NA | NA | 5% | 2% | 1% | 0. % | 13% | 6% | NA | NA | 2% | 2% | 1% | 5% |
Baseline surveys were conducted before any treatments had taken place. Preburn surveys were conducted 2 weeks after herbicide application. The 3- and 12-month surveys were conducted after both burning and herbicide were applied.
Burned | Unburned | ||||||||||||
---|---|---|---|---|---|---|---|---|---|---|---|---|---|
Survey | Baseline | Pre-burn | 3-month | 6-month | 9-month | 12-month | Baseline | Pre-burn | 3-month | 6-month | 9-month | 12-month | |
Percent cover | Percent cover | ||||||||||||
Ageratum houstonianum A | 0 | 0 | 0 | 0 | 0 | 0 | 0 | 0 | 0 | 0 | 0 | 4 | |
Amaranthus spinosus A | 0 | 0 | 0 | 0 | 0 | 0 | 0 | 0 | 0 | 1 | 0 | 0 | |
Ambrosia artemisiifolia A | 0 | 0 | 9 | 21 | 6 | 0 | 0 | 0 | 0 | 4 | 0 | 0 | |
Andropogon virginicus | 0 | 0 | 0 | 0 | 0 | 5 | 0 | 0 | 0 | 0 | 0 | 0 | |
Anredera cordifolia A | 14 | 9 | 9 | 17 | 4 | 5 | 36 | 3 | 14 | 20 | 25 | 6 | |
Aphananthe philippinensis | 0 | 0 | 0 | 0 | 1 | 100 | 0 | 0 | 0 | 3 | 0 | 0 | |
Araujia sericifera A | 3 | 2 | 3 | 2 | 1 | 1 | 2 | 5 | 5 | 5 | 5 | 14 | |
Bidens pilosa A | 30 | 0 | 16 | 13 | 15 | 2 | 10 | 0 | 5 | 10 | 9 | 3 | |
Bidens subalternans A | 0 | 0 | 0 | 0 | 0 | 0 | 0 | 0 | 1 | 0 | 0 | 0 | |
Bromus catharticus A | 0 | 0 | 0 | 0 | 0 | 0 | 0 | 0 | 1 | 0 | 0 | 0 | |
Calochlaena dubia | 0 | 5 | 6 | 0 | 0 | 0 | 29 | 48 | 45 | 21 | 25 | 27 | |
Cardamine hirsuta A | 6 | 0 | 2 | 0 | 0 | 0 | 0 | 0 | 0 | 0 | 0 | 0 | |
Casuarina cunninghamiana | 0 | 0 | 0 | 0 | 0 | 0 | 0 | 0 | 36 | 0 | 0 | 0 | |
Cinnamomum camphora A | 48 | 34 | 0 | 0 | 0 | 0 | 0 | 0 | 0 | 0 | 0 | 0 | |
Cirsium vulgare A | 0 | 0 | 14 | 7 | 11 | 19 | 0 | 0 | 0 | 7 | 0 | 2 | |
Commelina cyanea | 0 | 0 | 1 | 0 | 0 | 0 | 0 | 0 | 3 | 1 | 0 | 0 | |
Crassocephalum crepidioides A | 0 | 0 | 3 | 0 | 2 | 0 | 0 | 0 | 0 | 0 | 0 | 0 | |
Cyclospermum leptophyllum A | 0 | 0 | 5 | 2 | 0 | 0 | 0 | 0 | 1 | 1 | 0 | 0 | |
Cyperaceae sp. | 0 | 0 | 6 | 0 | 0 | 0 | 0 | 0 | 0 | 0 | 0 | 0 | |
Cyperus brevifolius A | 0 | 0 | 0 | 0 | 5 | 0 | 0 | 0 | 4 | 0 | 0 | 0 | |
Cyperus eragrostis A | 0 | 0 | 0 | 0 | 0 | 0 | 0 | 0 | 0 | 1 | 0 | 0 | |
Cyperus gracilis | 0 | 0 | 0 | 0 | 0 | 0 | 0 | 0 | 0 | 0 | 4 | 0 | |
Cyperus polystachios | 0 | 0 | 0 | 0 | 0 | 4 | 0 | 0 | 0 | 0 | 0 | 0 | |
Cyperus sesquiflorus A | 0 | 0 | 6 | 0 | 27 | 0 | 0 | 0 | 0 | 0 | 0 | 0 | |
Cyperus sp. | 0 | 0 | 0 | 5 | 4 | 7 | 0 | 0 | 0 | 3 | 1 | 0 | |
Cyperus sphaeroideus | 0 | 0 | 0 | 0 | 7 | 0 | 0 | 0 | 0 | 1 | 0 | 0 | |
Desmodium sp. | 0 | 0 | 0 | 0 | 0 | 1 | 0 | 0 | 0 | 0 | 0 | 0 | |
Desmodium uncinatum A | 0 | 0 | 1 | 8 | 1 | 0 | 0 | 0 | 0 | 0 | 0 | 0 | |
Dichondra repens | 0 | 0 | 0 | 1 | 1 | 0 | 0 | 0 | 0 | 0 | 0 | 0 | |
Einadia trigonos | 0 | 0 | 0 | 0 | 55 | 0 | 0 | 0 | 2 | 2 | 1 | 11 | |
Erigeron bonariensis A | 0 | 0 | 12 | 10 | 3 | 3 | 0 | 0 | 1 | 9 | 3 | 4 | |
Fimbristylis dichotoma | 0 | 0 | 0 | 0 | 4 | 0 | 0 | 0 | 0 | 0 | 0 | 0 | |
Gomphocarpus physocarpus | 0 | 0 | 1 | 1 | 2 | 1 | 0 | 5 | 1 | 0 | 3 | 2 | |
Hydrocotyle acutiloba | 0 | 0 | 2 | 0 | 1 | 0 | 0 | 0 | 0 | 2 | 0 | 0 | |
Hydrocotyle sp. | 1 | 0 | 0 | 0 | 2 | 0 | 0 | 0 | 0 | 0 | 0 | 0 | |
Hydrocotyle tripartita | 0 | 0 | 1 | 0 | 0 | 0 | 0 | 0 | 0 | 0 | 0 | 0 | |
Hypochaeris sp. A | 0 | 0 | 0 | 0 | 0 | 1 | 0 | 0 | 0 | 0 | 0 | 0 | |
Hypolepis muelleri | 0 | 0 | 0 | 0 | 3 | 2 | 41 | 33 | 34 | 8 | 18 | 19 | |
Imperata cylindrica | 6 | 0 | 0 | 3 | 0 | 0 | 0 | 0 | 0 | 0 | 0 | 0 | |
Juncaceae sp. | 0 | 0 | 0 | 0 | 1 | 0 | 0 | 0 | 0 | 0 | 0 | 0 | |
Juncus sp. | 0 | 0 | 3 | 0 | 3 | 2 | 0 | 0 | 0 | 0 | 0 | 0 | |
Juncus ustaisis | 0 | 0 | 0 | 0 | 0 | 0 | 0 | 0 | 8 | 0 | 0 | 0 | |
Kennedia rubicunda | 0 | 0 | 1 | 0 | 3 | 0 | 0 | 0 | 0 | 0 | 0 | 0 | |
Lantana camara A | 61 | 33 | 3 | 29 | 23 | 4 | 64 | 18 | 9 | 1 | 6 | 14 | |
Ligustrum sinense A | 8 | 11 | 53 | 27 | 5 | 0 | 0 | 0 | 10 | 10 | 12 | 51 | |
Lobelia purpurascens | 0 | 0 | 1 | 0 | 0 | 1 | 11 | 0 | 4 | 13 | 2 | 2 | |
Lysimachia arvensis A | 0 | 0 | 3 | 0 | 0 | 0 | 0 | 0 | 0 | 0 | 0 | 0 | |
Maclura cochinchinensis | 0 | 0 | 0 | 0 | 0 | 0 | 61 | 8 | 9 | 13 | 5 | 15 | |
Maekawaea rhytidophylla | 0 | 0 | 0 | 2 | 0 | 0 | 0 | 0 | 0 | 2 | 0 | 0 | |
Mallotus philippensis | 0 | 0 | 0 | 0 | 0 | 2 | 0 | 0 | 0 | 6 | 4 | 0 | |
Medicago polymorpha A | 0 | 0 | 0 | 1 | 1 | 0 | 0 | 0 | 0 | 0 | 0 | 0 | |
Melinis repens A | 0 | 0 | 0 | 0 | 14 | 0 | 0 | 0 | 0 | 0 | 0 | 0 | |
Oxalis corniculata A | 0 | 0 | 3 | 0 | 5 | 3 | 0 | 0 | 1 | 1 | 2 | 0 | |
Oxalis sp. A | 0 | 0 | 1 | 5 | 3 | 3 | 0 | 0 | 0 | 2 | 0 | 2 | |
Paspalum mandiocanum A | 74 | 0 | 56 | 78 | 90 | 77 | 82 | 0 | 67 | 72 | 83 | 60 | |
Passiflora subpeltata A | 2 | 11 | 1 | 19 | 2 | 1 | 0 | 0 | 0 | 1 | 1 | 0 | |
Phytolacca octandra A | 0 | 0 | 0 | 0 | 5 | 0 | 0 | 0 | 0 | 0 | 3 | 0 | |
Plantago lanceolata A | 0 | 0 | 4 | 0 | 0 | 0 | 0 | 0 | 0 | 0 | 0 | 0 | |
Poa labillardierei | 0 | 0 | 0 | 0 | 0 | 0 | 0 | 0 | 0 | 30 | 0 | 0 | |
Pteridium esculentum | 23 | 13 | 15 | 36 | 30 | 25 | 0 | 52 | 0 | 44 | 7 | 17 | |
Ranunculus sessiliflorus | 0 | 0 | 1 | 0 | 0 | 0 | 0 | 0 | 0 | 0 | 0 | 0 | |
Rubus anglocandicans A | 29 | 3 | 8 | 4 | 0 | 5 | 0 | 0 | 0 | 0 | 0 | 0 | |
Rubus rosifolius | 25 | 0 | 18 | 2 | 25 | 4 | 0 | 0 | 0 | 0 | 0 | 0 | |
Rubus sp. | 0 | 0 | 0 | 0 | 0 | 0 | 0 | 0 | 1 | 0 | 0 | 0 | |
Rumex brownii | 0 | 0 | 0 | 1 | 0 | 4 | 0 | 0 | 0 | 0 | 0 | 0 | |
Rumex sp. | 5 | 0 | 26 | 2 | 2 | 0 | 15 | 0 | 0 | 1 | 0 | 0 | |
Senecio madagascariensis A | 0 | 0 | 4 | 3 | 5 | 3 | 0 | 0 | 2 | 1 | 0 | 11 | |
Senna septemtrionalis A | 0 | 0 | 0 | 0 | 0 | 0 | 9 | 10 | 24 | 0 | 22 | 0 | |
Setaria sphacelata A | 53 | 0 | 17 | 4 | 26 | 28 | 0 | 0 | 0 | 0 | 12 | 0 | |
Sida rhombifolia A | 5 | 0 | 0 | 2 | 1 | 1 | 0 | 0 | 0 | 0 | 0 | 0 | |
Sigesbeckia orientalis | 0 | 0 | 11 | 23 | 0 | 0 | 0 | 0 | 4 | 10 | 14 | 0 | |
Solanum americanum A | 0 | 0 | 0 | 0 | 0 | 0 | 0 | 0 | 0 | 0 | 5 | 0 | |
Solanum chenopodioides A | 0 | 0 | 0 | 3 | 9 | 0 | 0 | 0 | 0 | 18 | 3 | 1 | |
Solanum mauritianum A | 48 | 38 | 12 | 26 | 14 | 18 | 51 | 35 | 38 | 18 | 32 | 25 | |
Solanum nigrum A | 0 | 0 | 11 | 15 | 6 | 1 | 0 | 0 | 2 | 6 | 2 | 10 | |
Solanum seaforthianum A | 0 | 0 | 0 | 0 | 0 | 1 | 0 | 0 | 0 | 0 | 0 | 0 | |
Solanum sp. | 0 | 0 | 0 | 1 | 1 | 2 | 0 | 0 | 0 | 0 | 0 | 3 | |
Solanum viarum A | 0 | 0 | 0 | 3 | 1 | 0 | 3 | 0 | 0 | 7 | 11 | 2 | |
Sonchus asper A | 0 | 0 | 2 | 0 | 0 | 0 | 0 | 0 | 3 | 0 | 0 | 0 | |
Sonchus oleraceus A | 0 | 0 | 8 | 1 | 0 | 0 | 0 | 0 | 0 | 0 | 0 | 0 | |
Stachys lanata A | 0 | 0 | 12 | 16 | 13 | 4 | 0 | 0 | 0 | 0 | 0 | 0 | |
Stellaria media A | 2 | 0 | 3 | 0 | 1 | 6 | 0 | 0 | 1 | 0 | 0 | 0 | |
Tagetes minuta A | 0 | 0 | 4 | 0 | 23 | 0 | 0 | 0 | 3 | 0 | 11 | 0 | |
Tradescantia fluminensis A | 44 | 0 | 14 | 49 | 5 | 16 | 48 | 0 | 3 | 21 | 25 | 58 | |
Trifolium repens A | 0 | 0 | 1 | 0 | 0 | 0 | 0 | 0 | 0 | 0 | 0 | 0 | |
Urtica incisa | 0 | 0 | 0 | 0 | 0 | 0 | 5 | 0 | 3 | 0 | 2 | 9 | |
Verbena caracasana A | 0 | 0 | 0 | 40 | 4 | 13 | 0 | 0 | 0 | 4 | 2 | 0 | |
Verbena incompta A | 2 | 0 | 14 | 3 | 3 | 13 | 0 | 0 | 2 | 6 | 3 | 3 | |
Verbena sp. A | 0 | 0 | 0 | 0 | 1 | 1 | 0 | 0 | 0 | 0 | 0 | 3 | |
Xanthium occidentale A | 0 | 0 | 9 | 0 | 0 | 0 | 0 | 0 | 6 | 0 | 0 | 0 |