Fighting the flames: site-specific effects determine species richness of Australian frogs after fire
Brittany A. Mitchell




A
B
C
D
Abstract
Fire has played an integral role in regulating patterns of biodiversity for millions of years. However, anthropogenic disturbance and climate change has altered fire activity – driving increases in both fire severity and scale. The effect fire now has on the persistence of biodiversity is poorly known, especially for frogs. Studies examining frog responses to fire usually have small sample sizes, focus upon small geographic areas and are based on low-severity fires, which can mean results are not applicable to high-severity fires, such as those expected under future climate change.
Our aims were to examine (1) persistence of frog species, measured by species richness, up to 18 months post-fire, and (2) the effects of varying fire severity on frog species richness and recovery, where we expected higher fire severity to lead to lower species richness after fire.
Using large-scale citizen science data from the Australian Museum’s FrogID project, coupled with remotely sensed fire data, we present a spatially and taxonomically broad analysis examining post-fire recovery responses for Australian frog species after the 2019/2020 ‘Black Summer’ bushfires.
We reveal no overall decrease in the species richness of Australian frogs both in the short- and long-term post-fire. Furthermore, species richness did not decline with increasing fire severity. Instead, species richness and its response to fire was highly site-specific.
We provide evidence that widespread and common Australian frog species have persisted post-fire in most sites and concluded that this is potentially due to their ability to shelter from fire adequately and/or La Niña-driven high rainfall offering conditions conducive to breeding activity and persistence.
We show how citizen science provides critical data for conservation, especially in response to unprecedented disturbance events, such as the 2019/2020 megafires. Our research also highlights the need for ongoing and targeted scientific monitoring, especially for less common or threatened species.
Keywords: citizen science, climate change, conservation, ecology, fire, frogs, remote-sensing, species richness.
Introduction
Fire has regulated patterns of biodiversity for millions of years, but recent anthropogenic disturbances are altering fire impacts on the persistence of biodiversity (Kelly et al. 2020). Anthropogenically mediated climate change has increased fire severity and duration and geographic scale across the globe (Moritz et al. 2012; Jolly et al. 2015), exemplified by recent fires in western USA and south-eastern Australia (Nolan et al. 2020; Ward et al. 2020). Changes in fire regime are driven in part by: climatic variables, extending periods of dry, hot and windy weather; vegetation changes due to unprecedented precipitation periods; and increased likelihood of ignitions (Williams et al. 2009; Moritz et al. 2012; Kelly et al. 2020).
Many species have adapted to specific fire regimes, so disruption can impact both populations and entire ecosystems (Kelly et al. 2020). As fire increases in severity, frequency and scale under climate change, it can directly increase mortality rates, reduce available resources and habitat and fragment populations (Ward et al. 2020), increasing extinction rates of biodiversity (Gill and Bradstock 1995). Despite this potentially deleterious outcome, responses to fire are poorly documented, particularly for understudied taxa like amphibians, invertebrates and reptiles (Dale et al. 2001; Pastro et al. 2014; dos Anjos et al. 2021).
Frogs are already experiencing population declines globally due to various anthropogenic threats (Houlahan et al. 2000; Campbell Grant et al. 2020). They are likely to be particularly susceptible to high mortality rates or population decline with fire, given their low vagility and reduced ability to flee fire fronts, reliance upon water sources and specific habitat requirements that may be degraded post-fire (Rowley et al. 2020). They may also be increasingly vulnerable to extinction due to increased inbreeding, lower population numbers and low immigration rates with fires (Potvin et al. 2017). The potential vulnerability of frogs to fire, and other key threats such as chytridiomycosis (Berger et al. 2016), make studying the response of frogs to fire critically important.
Most of our understanding of how frogs respond to fire is biased towards North American species (dos Anjos et al. 2021), with few investigations in Australia by comparison. However, effects on Australian species vary. Some studies show frog species are not obviously affected by fire (Bamford 1992; Lowe et al. 2013; Rowley et al. 2020), whereas others show some species experiencing population declines. For example, Litoria littlejohni (Daly and Craven 2007) and Geocrinia lutea (Driscoll and Roberts 1997) experienced declines, and one study even documented a local population extinction (Lemckert 2000). Despite the contributions of finer-scale or localised studies elucidating the effects of fire on Australian frogs, we lack evidence across larger spatial scales or for mega-fires, such as those experienced in 2019/2020, and those that are predicted to increase under future climate change (Lee et al. 2022). Further, such conflicting evidence for a few species limits our ability to extrapolate the impact of fires from previous research.
Fires can be unpredictable in space and time and consequently, assembling resources to study their effects on short notice can be difficult. With the rise of citizen science, it is possible to investigate impacts of fire over large spatial scales and on a time-sensitive basis (Kirchhoff et al. 2021). Indeed, citizen science was instrumental in providing preliminary data on short-term persistence of frogs across large spatial scales after the ‘Black Summer’ bushfires in Australia, with at least 45 out of 66 species detected, and all 33 species with more than five calling records pre-fire recorded calling post-fire (Rowley et al. 2020). However, this dataset was only short term, failed to sample range-restricted and rainforest species and focused on summer-breeding. This is particularly relevant because although some individuals may survive the immediate impact of a fire, they are persisting in a burnt landscape with fewer resources and potentially experiencing starvation, as well as an increased risk of predation (Nimmo et al. 2019; Dickman and McDonald 2020). Additionally, the impact of varied fire severity on frogs was not assessed.
In this study, we used large-scale citizen science data, coupled with two remotely sensed datasets from the extensive and high-severity 2019/20 Australian bushfire season to examine: (1) persistence of frog species, measured by species richness, 18 months post-fire across Australia; and (2) effects of varying fire severity on frog species richness and recovery in eastern Australia, where megafires were concentrated. More specifically, we hypothesised that fire would have a negative effect on species richness, particularly at higher severity levels.
Materials and methods
FrogID data
Frog location data were derived from the Australian Museum’s FrogID project, whereby users submit a 20–60-s audio recording of a frog advertisement call through the FrogID smartphone app, hereafter known as a ‘submission’ (Rowley et al. 2019). A submission can contain multiple frogs calling, forming ‘records’. Species calling in each submission are identified based upon call, location, habitat and time of year. Calls are compared with a large database of recordings from known species. Frog species that are difficult to identify are listened to by multiple people and occasionally analysed in sound analysis software. If uncertainty remains over the species identification, we classify the frog as ‘unidentified’, and the recording does not contribute to the FrogID dataset. FrogID is a relatively non-structured citizen science project, with the majority of records from more populated areas. However, the dataset includes records from over 35% of the country (using 0.5 decimal degree grid cells; Cutajar et al. 2022). These data from FrogID indicate breeding effort and habitat, reflected in metadata identifying time, date and location (latitude, longitude and an estimate of location accuracy). Data were filtered by location accuracy, with records over 3000 m discarded, because this measurement commonly means the app could not take the user’s location. Over 96% of records had an accuracy of less than 100 m.
Remote sensing
To estimate time and extent of fire, we used multiple sources of remotely sensed data. First, we extracted data regarding the time of fire from the Digital Earth Australia (DEA) Hotspots resource (https://hotspots.dea.ga.gov.au/). We placed a 0.005° (approximately 550 m) buffer around each FrogID record and determined the highest temperature recorded within it, assuming that the highest temperature record coincided with the closest time when the fire front passed each location (Rowley et al. 2020). Records were then clipped to shapefiles of fire extent from the National Indicative Aggregated Fire Index (NIAFI) (http://www.environment.gov.au/fed/catalog/search/resource/details.page?uuid=%7B9ACDCB09-0364-4FE8-9459-2A56C792C743%7D) to ensure all records were from burnt regions. DEA/NIAFI data were split into pre-fire (from 2017 until date of fire), short-term post-fire (up to 13 April 2020) and long-term post-fire (after 13 April 2020 until 10 April 2022). To investigate the effect of fire severity, we used Fire Extent and Severity Mapping (FESM) data (https://datasets.seed.nsw.gov.au/dataset/fire-extent-and-severity-mapping-fesm) to allocate each FrogID record to a fire severity category (2 = low, 3 = moderate, 4 = high, 5 = extreme). Fire severity category 1 refers to areas that were burnt but the extent is unclear and not reliable. Due to unreliability, we excluded this category from our analysis. The FESM data have been generated from a collaboration with the NSW Rural Fire Service and the NSW Government Department of Planning, Industry, and Environment Remote Sensing and Regulatory Mapping team (who have developed a semiautomated approach to mapping fire extent and severity through a machine-learning framework based on Sentinel 2 satellite imagery). These data were restricted to New South Wales (NSW) and the Australian Capital Territory (ACT), where fires were highly concentrated. Comparable data were not available for other states or territories. We also note that FESM data are an estimate of fire severity based on training data and are broad-scale, and some errors in severity classification may occur (White and Gibson 2022). However, these errors are seen when distinguishing between low- and moderate-severity fires, and between high- and extreme-severity fires (White and Gibson 2022). Despite these potential uncertainties, FESM was the most logistically feasible dataset for our large-scale analysis and has been the preferred choice in numerous remote-sensing studies (Bilney et al. 2022; Beranek et al. 2023; Law et al. 2022, 2023). More information regarding the FESM dataset can be found here https://datasets.seed.nsw.gov.au/dataset/fire-extent-and-severity-mapping-fesm/resource/73b509a1-7674-45c2-adac-173ccd7d917a. For FESM derived data, we similarly filtered FrogID data into two categories: pre-fire and post-fire.
Species’ habitat preferences can also influence the likelihood they are susceptible to potential fires – for example, which refugia they are likely to shelter in, and their dependency on fire-sensitive habitat (Mahony et al. 2023). As such, we examined the composition of FrogID records before, short-term, and long-term after fire in three categories: their ecological grouping (terrestrial breeders, ephemeral pond, permanent water, permanent stream associated and bog or soak); their dependence on fire-sensitive vegetation (five categories: 0–20%, 21–40%, 41–60%, 61–80%, 81–100%); and likely refugia used to shelter from fire (burrow, hollow, under debris, under rocks, under leaf litter or topsoil, dense riparian vegetation and wetland).
Data analysis
All data analyses were conducted in R ver. 4.3.0 (R Core Team 2021), relying heavily on the tidyverse workflow (Wickham 2017). To account for sampling bias inherent to citizen science data, we created ‘field sampling sites’ by placing 1-km grids over Australia (5 km for DEA Hotspot/NIAFI data due to lower resolution) and only sampled grids (or sites) in fire-affected areas with both before and after records for frogs. This limited incorrect species richness counts (response variable of interest) in an area that may have only been sampled before fire and not after (either due to no frogs calling or absence of a citizen scientist recording). This also removed grids that were only sampled post-fire. Two additional measures to limit bias from any uneven sampling were implemented. The first was only grids with at least five submissions both before and after fire were included for species richness to control ‘between grid’ variation. This limited our sample size for number of grid/sites but provided greater confidence in any changes in species richness seen at each site. The second was to account for ‘within grid’ variation and ensured even-sampling pre- and post-fire within a grid. For example, if a grid had 18 submissions pre-fire and 32 submissions post-fire, only 18 submissions from post-fire were randomly sampled 10 times and then averaged to calculate species richness. Most post-fire grids were re-sampled because we had significantly more data post-fire (n = 9017) than pre-fire (n = 1733). Because we randomly sampled species richness to account for sampling biases before and after fire, the composition of the species changed among random samples. Therefore, our analysis focused on community-level metrics (i.e. species richness) as opposed to species-level metrics. However, we calculated the percentage of presence records of frog species across sampling grids in areas burnt in each severity level (low, moderate, high, extreme; Supplementary Tables S4–S7). For each grid, we calculated the Keith vegetation value, a highly detailed classification of vegetation types in Australia (Keith 2004). Our data fell into four main vegetation types: forest (including wet sclerophyll, dry sclerophyll, and rainforest); woodlands; scrubs/heath; and cleared.
To compare frog species richness before and after fire across Australia, we used two generalised additive models (GAMs) – one for DEA Hotspot/NIAFI data and one for FESM data (Wood 2017). For the DEA Hotspot/NIAFI model, the response variable was species richness, with two fixed effects: fire, and the number of samples (additional control for difference in sampling size between grids). Location was added as a bivariate smoothed term (k = 10). Similarly, for the FESM model, the response variable was change in species richness from before to after fire, with three fixed effects: severity of fire; vegetation type and the number of samples; and location (bivariate smoothed term, k = 10).
Results
Overall, 36 well sampled species (≥5 records before fire) called in the short-term after fire, and all 49 species previously recorded with FrogID in FESM burnt areas called long-term post-fire. These species were taxonomically diverse, representing three of five native frog families in Australia (Fig. 1). Frogs responded similarly in the NIAFI/DEA Hotspot data (Fig. S1). About 58% of the frog species ever detected in FESM burnt areas by FrogID (including those with <5 records) before the fire were calling after 3 months, increasing to 72% after 6 months, 92% after 12 months and 98% after 18 months, until all were calling by around 2 years post-fire (Fig. S2). Frogs with the highest number of records post-fire were of low conservation concern (categorised as ‘least concern’ by the IUCN). Seven threatened species, Crinia tinnula (VU), Litoria subglandulosa (VU), Litoria olongburensis (VU), Philoria pughi (EN), Philoria sphagnicola (EN), Mixophys iteratus (EN) and Litoria watsoni (EN), and one near-threatened species, Adelotus brevis, called long-term after fire but had low numbers of records (Fig. 1).
Well sampled frog species with high numbers of FrogID submissions (n ≥ 5) calling before fire (blue), short-term after fire (yellow) and longer-term after fire (orange) using New South Wales Fire Extent and Severity Mapping (FESM) data. All species were found to be calling in the long-term. Red dots indicate threatened and near-threatened species. Horizontal axis is truncated due to high numbers of records for some species. N = unique number of sites.
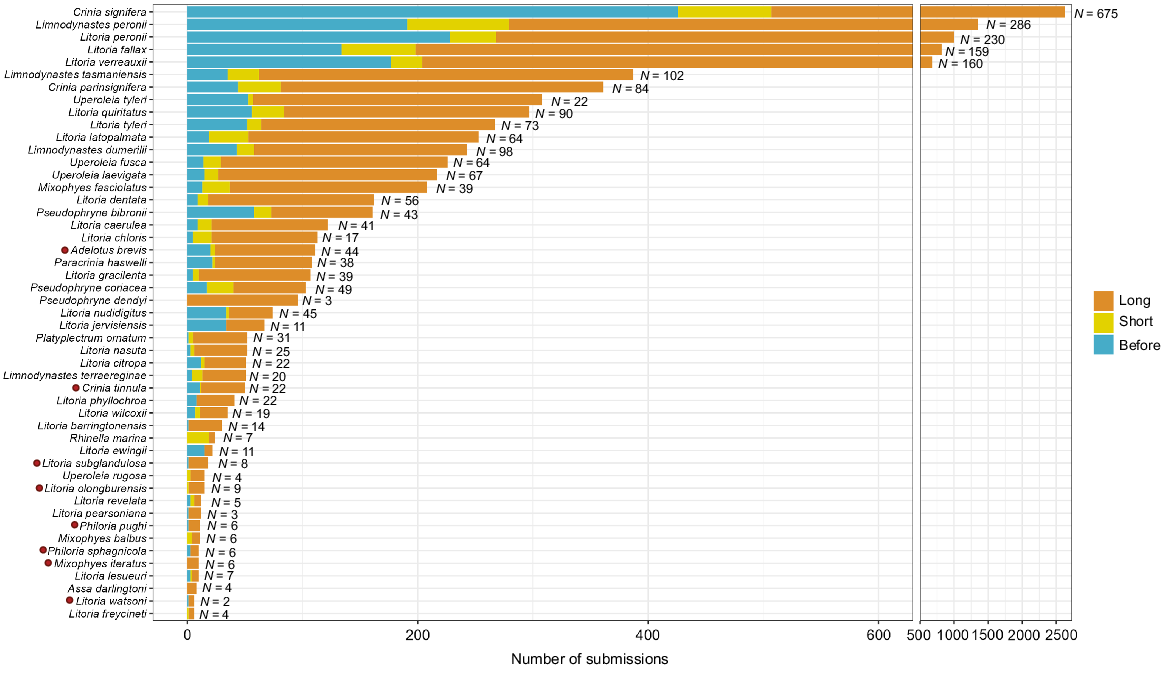
Frogs from all ecological groups (terrestrial, ephemeral pond, permanent pond, stream associated, terrestrial and bog/soak) were present after fire, with comparably proportional records between groups for before, short- and longer-term after fire (Fig. S3). However, frog species associated with permanent waterbodies were the best represented in the dataset, and there were low number of records for frogs associated with bogs/soaks and those that relied upon both terrestrial and ephemeral ponds (Fig. S3). Frogs that had a low (0–20%) dependence on fire-sensitive vegetation were best represented in our data, and those with a high dependence were present post-fire but had a low number of records (Fig. S4). The most common frog species in our dataset were those that found refuge under debris during fire; however, frogs from all refugia groupings were present post-fire, albeit in lower numbers (Fig. S5). Mean species richness did not significantly change post-fire compared with pre-fire (estimate = −0.130, z = −0.986, P = 0.324; Fig. 2). Instead, changes in species richness were site specific, increasing for nine sites, remaining unchanged for four sites and decreasing for six sites post-fire (Fig. 3; Table S1). Although species richness was, on average, higher in low-severity burn areas, species richness did not significantly differ with varying fire severity level, even after accounting for vegetation type and sample size (P > 0.05; Table 1, Fig. 4). Similarly for DEA Hotspot/NIAFI data, changes in species richness were site-specific (Table S2). Specific changes to species and certain ecological groups varied across severity levels (Tables S3–S7).
(a) Overall species richness for bushfire-affected areas before and after fire for DEA Hotspot/NIAFI datasets encompassing New South Wales and Victoria. (b) FESM fire severity dataset encompassing only New South Wales. Slope of lines represent direction of change in species richness for each sampling site (either increase, decrease, or no change).
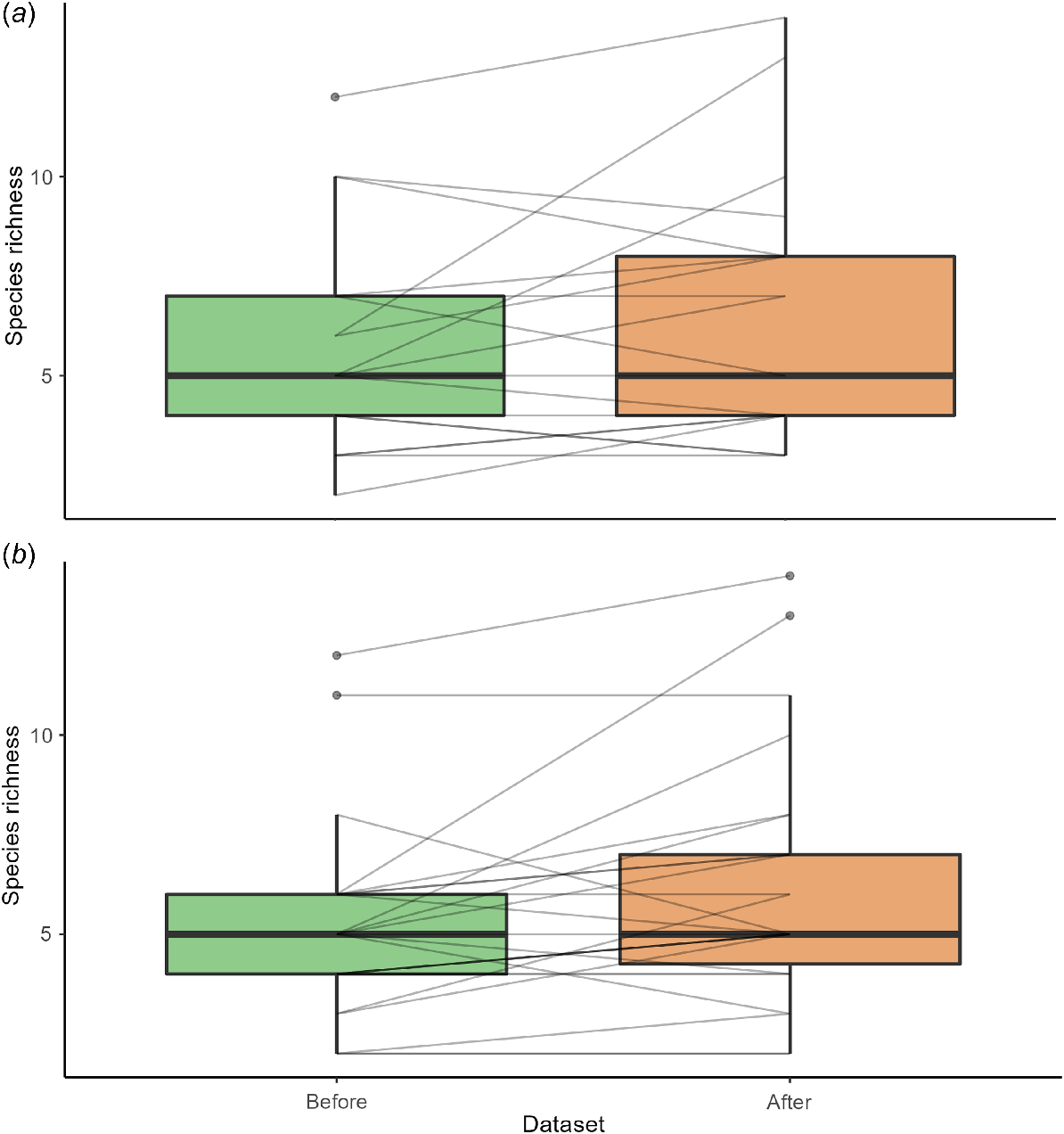
Change in species richness per DEA/NIAFI site in southeast Australia. Species richness increased in some sites and decreased in others post-fire. Some sites did not change species richness. Shaded areas indicate fire zones.
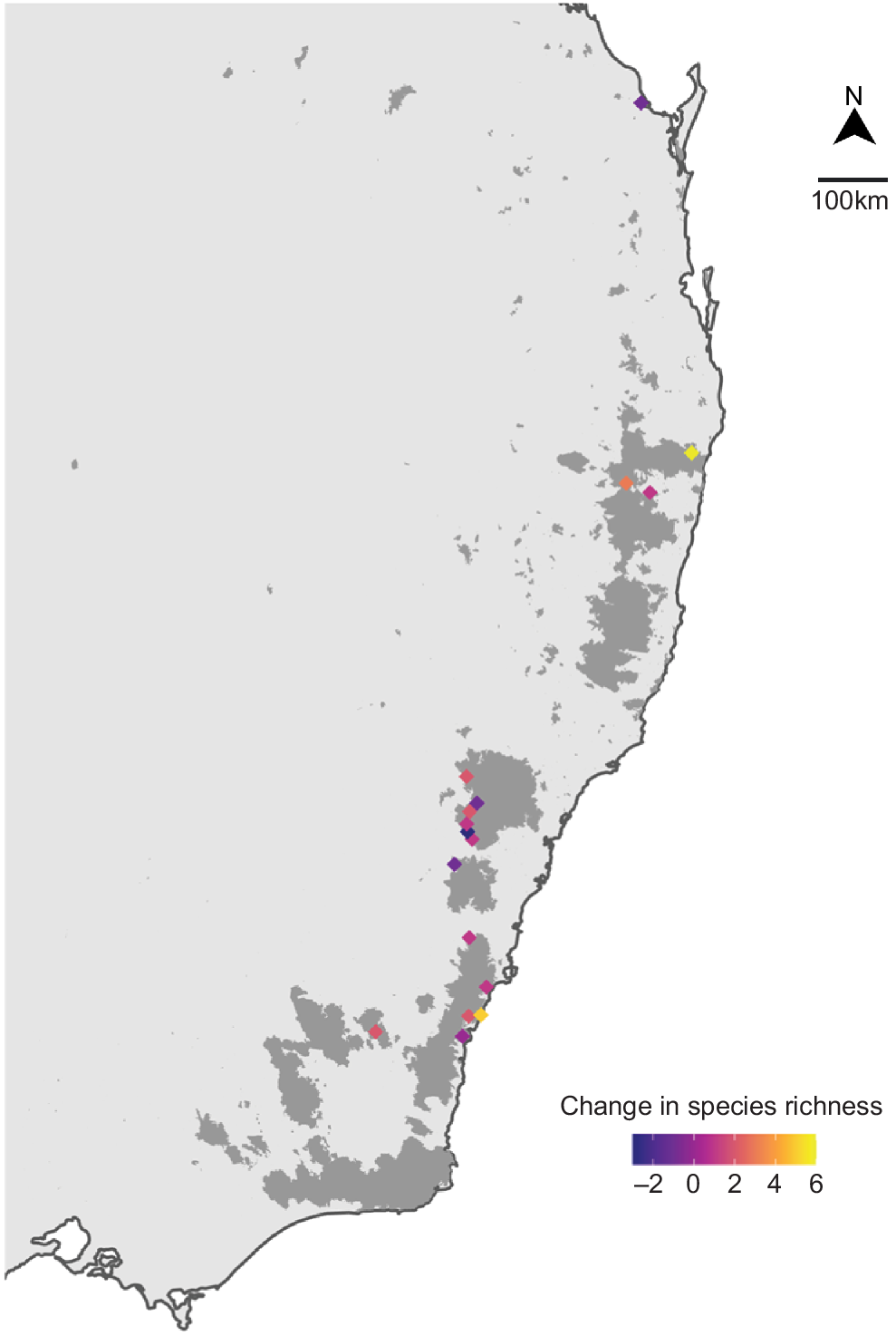
Estimate | t-value | P | ||
---|---|---|---|---|
Extreme (intercept) | 1.959 | 0.806 | 0.432 | |
High | −1.701 | −0.653 | 0.523 | |
Moderate | −0.294 | −0.116 | 0.909 | |
Low | −0.773 | −0.261 | 0.797 | |
Forest | 0.017 | 0.018 | 0.986 | |
Scrub/Heath | −0.188 | −0.051 | 0.960 | |
Woodland | 1.613 | 0.633 | 0.536 | |
N | −0.014 | −0.717 | 0.483 |
d.f. | F-value | P | ||
---|---|---|---|---|
s(lng, lat) | 2 | 1.048 | 0.374 |
Species richness per site under four different fire severity categories (low, moderate, high and extreme) in New South Wales. Species richness overall did not significantly change before and after fire between the different levels of fire severity. Shaded areas indicate fire zones. N represents the number of sites within each fire severity category.
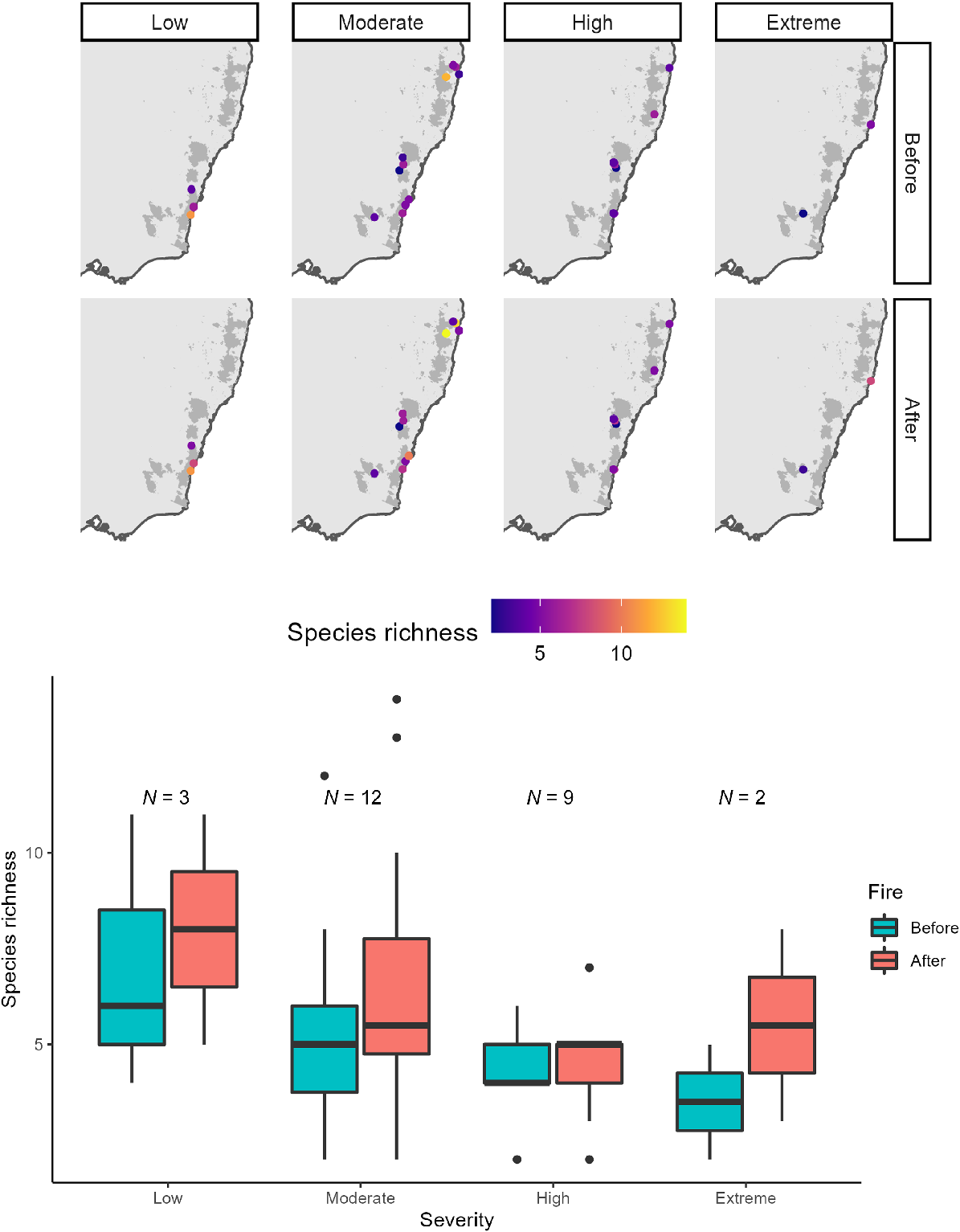
Discussion
We present a geographically and taxonomically broad analysis examining post-fire responses for frog species across south-eastern Australia – a continent increasingly affected by climate change and consequently increasing fire severity and extent (Bureau of Meteorology, CSIRO 2020; Lawrence et al. 2022). Encouragingly, Australian frog species richness did not decline overall after fire even with increasing fire severity, against our original hypothesis. Species richness increased in some survey areas but decreased in other areas, suggesting site-specific effects.
These variations in frog species richness at site level is consistent with other studies, identifying some increases (Brown et al. 2011; Allingham and Harvey 2013; Grundel et al. 2015; Mester et al. 2015) but also decreases after fire (Cano and Leynaud 2010; Constible et al. 2016). Such dichotomous responses occur in Australian frogs, with no discernible effects of fire detected for Heleioporus eyrei, Litoria olongburensis, L. freycineti or Crinia tinnula, but a negative impact was documented for both Litoria littlejohni (Daly and Craven 2007) and Geocrinia lutea (Driscoll and Roberts 1997). Furthermore, Beranek et al. (2023) found that amphibian metacommunities in the southern regions of NSW were negatively impacted by high fire severity, whereas an effect was not detected in the northern region. Generalist species may respond positively to fire (e.g. Anaxyrus spp.; dos Anjos et al. (2021), compared with specialist species (e.g. Phyllodytes luteolus; (Papp and Papp 2000; dos Anjos et al. 2021). Our generalist/common species (Crinia signifera, Litoria peronii, Limnodynastes peronii) had the most records compared with more specialist species with few records (for example, our threatened species). Persistence in post-fire landscapes reflects evolutionary history (see Beranek et al. 2023), ecological interactions, time since last burn, availability of local waterbodies and available shelter (dos Anjos et al. 2021). Some frogs can hide in burrows, ant-mounds and even bromeliads during fires (Moreira et al. 2009; Nomura et al. 2009; dos Anjos et al. 2021). Australian frogs take shelter in hollows, under bark, in dense vegetation, within deep soil cracks or within reeds such as Lomandra spp. (Mahony et al. 2023). Changes in diversity are likely to be considerably less sensitive than changes in abundance, and fire may impact frog population size, which may not be captured in our dataset (Hillebrand et al. 2018; Antão et al. 2020). It would also be useful to examine what factors drive adaptiveness to fire regimes in different frog species and what makes some species more susceptible. Our analysis was not able to necessarily differentiate between persistence and recolonisation, because some species presence post-fire could be due to quickly recolonising burnt areas rather than persistence through the fire period. Fine-scaled in-situ research, however, could contribute to better understanding these differences after future fire events.
Although our surveys were widespread, they are unlikely to include all frog species present. Time of year and weather, in particular, influence frog calling activity (Thompson et al. 2022), with some species only detectable under specific conditions (e.g. Litoria brevipalmata are rarely detected except after recent heavy rainfall). In addition, range-restricted species that occur in remote areas are less well sampled via the FrogID project and other citizen science projects. Other survey methods, such as targeted scientific surveys or passive acoustic monitoring (Beranek et al. 2023), may be necessary to elucidate the effects of fire on these species. This may explain why, despite considerable data collection, there were still relatively few sites with high fire impact (n = 11; Fig. 4). Other species may remain undetected if surveys were not conducted at the right time of year (e.g. Pseudophryne bibronii are most active in autumn). Moreover, we had relatively few records for many threatened species (in some cases none), limiting our ability to test their responses after the 2019/20 bushfires. This may be due to low survey effort in the habitat of many of the threatened frog species in the fire zone (i.e. relatively remote rainforest habitats). Thus, our analysis is complementary to targeted, ‘on the ground’ surveys for threatened species to quantify their responses post-fire (Beranek et al. 2023). Nonetheless, the high levels of sampling overall by citizen scientists post-fire increases confidence in areas where we see sites with decreased species richness. For example, less than half of the species were detected at a site in the Blue Mountains (NSW) (seven before to only three species post-fire), despite over three times more sampling effort post-fire. The site-specific changes our data reveal will inform identification of priority zones for future surveys to determine the full effects of fire on frog populations. For instance, these field-based surveys will be necessary to detect breeding success and to assess less readily detectable impacts from ecological data, such as genetic erosion (Potvin et al. 2017).
Moreover, unlike most Australian bushfire seasons, the subsequent onset of La Niña (September 2020) provided additional rainfall to the burnt landscape (Bureau of Meteorology 2020) – offering conditions likely more conducive for frog breeding activity and persistence. Thus, we emphasise that our results should be interpreted with caution: future results may differ if we see similar bushfire activity under more erratic El Niño Southern Oscillation (ENSO)/Indian Ocean Dipole (IOD) cycles due to climate change (Cai et al. 2009, 2013; Fasullo et al. 2018). For instance, an overall decrease in species richness may occur under El Niño conditions because necessary requirements for breeding recovery (such as water availability) may not be met. Furthermore, this study does not consider the interactions with other stressors and extreme events predicted under climate change, such as drought (King et al. 2017) or disease (Hossack et al. 2013). Thus, future research integrating these additional stressors with fire would be of great interest.
In conservation biology, we require extensive yet timely data to make informed decisions (Kirchhoff et al. 2021). After the severe bushfires, the global pandemic made collection by professional biologists problematic (Corlett et al. 2020). As a result, species priority lists for post-fire monitoring were estimated based on expert advice in the absence of the most up-to-date data. However, our study identifies the importance of citizen science in providing critical data for conservation, especially in response to unprecedented fires, and highlights how citizen science may also be relevant for other disturbances. It provided useful data on the effects of fire severity and extent, which did not significantly affect species richness of frogs overall in either the short- or long-term after fire. We emphasise the need for ongoing citizen science data collection and targeted scientific monitoring, especially for species less well-sampled, via citizen science and for threatened species.
Data availability
Not all data can be made Open Access due to data sensitivity/privacy of the underlying recordings and localities of threatened or otherwise sensitive species (Rowley and Callaghan 2020), but most data (i.e. with exact localities of sensitive species buffered) are made available to the public on an annual basis (Rowley and Callaghan 2020). Code used within our study will be made available in a Zenodo repository. Further data can also be requested from the Australian Museum.
Declaration of funding
This research was funded in part by Project No. GA-2000224 from the Australian Government Department of Agriculture, Water and Environment’s ‘Wildlife and Habitat Bushfire Recovery Program 2019–2020’. Furthermore, this research has been supported by an Australian Government Research Training Program (RTP) Scholarship.
Acknowledgements
We would like to thank the Citizen Science Grants of the Australian Government and the Impact Grants program of IBM Australia for providing funding and resources to help build the initial FrogID app; the generous donors who have provided funding for the project along with AGL as Partner; the NSW Biodiversity Conservation Trust as Supporting Partner; the Museum and Art Gallery of the Northern Territory, Museums Victoria, Queensland Museum, South Australian Museum, Tasmanian Museum and Art Gallery, and Western Australian Museum as FrogID partner museums; the many Australian Museum staff and volunteers who make up the FrogID team; and, most importantly, the thousands of citizen scientists across Australia who have volunteered their time to record frogs.
References
Allingham SM, Harvey M (2013) Effects of different fire regimes on amphibian communities in the Nyika National Park, Malawi. Current Herpetology 32, 1-8.
| Crossref | Google Scholar |
Antão LH, Pöyry J, Leinonen R, Roslin T (2020) Contrasting latitudinal patterns in diversity and stability in a high-latitude species-rich moth community. Global Ecology and Biogeography 29, 896-907.
| Crossref | Google Scholar |
Bamford MJ (1992) The impact of fire and increasing time after fire upon heleioporus eyrei, limnodynastes dorsalis and myobatrachus gouldii (Anura: Leptodactylidae) in Banksia woodland near Perth, Western Australia. Wildlife Research 19, 169-178.
| Crossref | Google Scholar |
Beranek CT, Hamer AJ, Mahony SV, Stauber A, Ryan SA, Gould J, Wallace S, Stock S, Kelly O, Parkin T, Weigner R, Daly G, Callen A, Rowley JJL, Klop-Toker K, Mahony M (2023) Severe wildfires promoted by climate change negatively impact forest amphibian metacommunities. Diversity and Distributions 29, 785-800.
| Crossref | Google Scholar |
Berger L, Roberts AA, Voyles J, Longcore JE, Murray KA, Skerratt LF (2016) History and recent progress on chytridiomycosis in amphibians. Fungal Ecology 19, 89-99.
| Crossref | Google Scholar |
Bilney RJ, Kambouris PJ, Peterie J, Dunne C, Makeham K, Kavanagh RP, Gonsalves L, Law B (2022) Long-term monitoring of an endangered population of Yellow-bellied Glider Petaurus australis on the Bago Plateau, New South Wales, and its response to wildfires and timber harvesting in a changing climate. Australian Zoologist 42, 592-607.
| Crossref | Google Scholar |
Brown DJ, Baccus JT, Means DB, Forstner MRJ (2011) Potential positive effects of fire on juvenile amphibians in a southern USA pine forest. Journal of Fish and Wildlife Management 2, 135-145.
| Crossref | Google Scholar |
Bureau of Meteorology (2020) La Niña underway in the tropical Pacific. Climate Driver Update archive. Available at http://www.bom.gov.au/climate/enso/wrap-up/archive/20200929.archive.shtml [Accessed 16 September 2021]
Bureau of Meteorology, CSIRO (2020) State of the Climate Report. Available at https://www.csiro.au/en/research/environmental-impacts/climate-change/State-of-the-Climate/Previous/State-of-the-Climate-2020
Cai W, Cowan T, Raupach M (2009) Positive Indian Ocean Dipole events precondition southeast Australia bushfires. Geophysical Research Letters 36, L19710.
| Crossref | Google Scholar |
Cai W, Zheng X-T, Weller E, Collins M, Cowan T, Lengaigne M, Yu W, Yamagata T (2013) Projected response of the Indian Ocean Dipole to greenhouse warming. Nature Geoscience 6, 999-1007.
| Crossref | Google Scholar |
Campbell Grant EH, Miller DAW, Muths E (2020) A Synthesis of evidence of drivers of amphibian declines. Herpetologica 76, 101-107.
| Crossref | Google Scholar |
Cano PD, Leynaud GC (2010) Effects of fire and cattle grazing on amphibians and lizards in northeastern Argentina (Humid Chaco). European Journal of Wildlife Research 56, 411-420.
| Crossref | Google Scholar |
Constible JM, Gregory PT, Anholt BR (2016) Patterns of distribution, relative abundance, and microhabitat use of anurans in a boreal landscape influenced by fire and timber harvest. Écoscience 8, 462-470.
| Crossref | Google Scholar |
Corlett RT, Primack RB, Devictor V, Maas B, Goswami VR, Bates AE, Koh LP, Regan TJ, Loyola R, Pakeman RJ, Cumming GS, Pidgeon A, Johns D, Roth R (2020) Impacts of the coronavirus pandemic on biodiversity conservation. Biological Conservation 246, 108571.
| Crossref | Google Scholar | PubMed |
Cutajar TP, Portway CD, Gillard GL, Rowley JJL (2022) Australian frog atlas: species’ distribution maps informed by the FrogID dataset. Technical Reports of the Australian Museum online 36, 1-48.
| Crossref | Google Scholar |
Dale VH, Joyce LA, McNulty S, Neilson RP, Ayres MP, Flannigan MD, Hanson PJ, Irland LC, Lugo AE, Peterson CJ, Simberloff D, Swanson FJ, Stocks BJ, Wotton BM (2001) Climate change and forest disturbances. BioScience 51, 723-734.
| Crossref | Google Scholar |
Daly G, Craven P (2007) Monitoring populations of Heath Frog Litoria littlejohni in the Shoalhaven region on the south coast of New South Wales. Australian Zoologist 34, 165-172.
| Crossref | Google Scholar |
Dickman C, McDonald T (2020) Some personal reflections on the present and future of Australia’s fauna in an increasingly fire-prone continent. Ecological Management & Restoration 21, 86-96.
| Crossref | Google Scholar |
dos Anjos AG, Solé M, Benchimol M (2021) Fire effects on anurans: what we know so far? Forest Ecology and Management 495, 119338.
| Crossref | Google Scholar |
Driscoll DA, Roberts JD (1997) Impact of fuel-reduction burning on the frog Geocrinia lutea in southwest Western Australia. Australian Journal of Ecology 22, 334-339.
| Crossref | Google Scholar |
Fasullo JT, Otto-Bliesner BL, Stevenson S (2018) ENSO’s changing influence on temperature, precipitation, and wildfire in a warming climate. Geophysical Research Letters 45, 9216-9225.
| Crossref | Google Scholar |
Grundel R, Beamer DA, Glowacki GA, Frohnapple KJ, Pavlovic NB (2015) Opposing responses to ecological gradients structure amphibian and reptile communities across a temperate grassland–savanna–forest landscape. Biodiversity and Conservation 24, 1089-1108.
| Crossref | Google Scholar |
Hillebrand H, Blasius B, Borer ET, Chase JM, Downing JA, Eriksson BK, Filstrup CT, Harpole WS, Hodapp D, Larsen S, Lewandowska AM, Seabloom EW, van de Waal DB, Ryabov AB (2018) Biodiversity change is uncoupled from species richness trends: Consequences for conservation and monitoring. Journal of Applied Ecology 55, 169-184.
| Crossref | Google Scholar |
Hossack BR, Lowe WH, Ware JL, Corn PS (2013) Disease in a dynamic landscape: Host behavior and wildfire reduce amphibian chytrid infection. Biological Conservation 157, 293-299.
| Crossref | Google Scholar |
Houlahan JE, Findlay CS, Schmidt BR, Meyer AH, Kuzmin SL (2000) Quantitative evidence for global amphibian population declines. Nature 404, 752-755.
| Crossref | Google Scholar |
Jolly WM, Cochrane MA, Freeborn PH, Holden ZA, Brown TJ, Williamson GJ, Bowman DMJS (2015) Climate-induced variations in global wildfire danger from 1979 to 2013. Nature Communications 6, 7537.
| Crossref | Google Scholar |
Kelly LT, Giljohann KM, Duane A, Aquilué N, Archibald S, Batllori E, Bennett AF, Buckland ST, Canelles Q, Clarke MF, Fortin M-J, Hermoso V, Herrando S, Keane RE, Lake FK, McCarthy MA, Morán-Ordóñez A, Parr CL, Pausas JG, Penman TD, Regos A, Rumpff L, Santos JL, Smith AL, Syphard AD, Tingley MW, Brotons L (2020) Fire and biodiversity in the Anthropocene. Science 370, eabb0355.
| Crossref | Google Scholar |
King AD, Karoly DJ, Henley BJ (2017) Australian climate extremes at 1.5 °C and 2 °C of global warming. Nature Climate Change 7, 412-416.
| Crossref | Google Scholar |
Kirchhoff C, Callaghan CT, Keith DA, Indiarto D, Taseski G, Ooi MKJ, Le Breton TD, Mesaglio T, Kingsford RT, Cornwell WK (2021) Rapidly mapping fire effects on biodiversity at a large-scale using citizen science. Science of The Total Environment 755, 142348.
| Crossref | Google Scholar | PubMed |
Law BS, Gonsalves L, Burgar J, Brassil T, Kerr I, O’Loughlin C (2022) Fire severity and its local extent are key to assessing impacts of Australian mega-fires on koala (Phascolarctos cinereus) density. Global Ecology and Biogeography 31, 714-726.
| Crossref | Google Scholar |
Law BS, Madani G, Lloyd A, Gonsalves L, Hall L, Sujaraj A, Brassil T, Turbill C (2023) Australia’s 2019–20 mega-fires are associated with lower occupancy of a rainforest-dependent bat. Animal Conservation 26, 103-114.
| Crossref | Google Scholar |
Lawrence J, Mackey B, Chiew F, Costello MJ, Hennessy K, Lansbury N, Nidumolu UB, Pecl G, Rickards L, Tapper N, Woodward A, Wreford A (2022) Australasia. In ‘Climate change 2022: impacts, adaptation, and vulnerability. Contribution of working group II to the sixth assessment report of the intergovernmental panel on climate change’. (Eds HO Pörtner, DC Roberts, M Tignor, ES Poloczanska, K Mintenbeck, A Alegría, M Craig, S Langsdorf, S Löschke, V Möller, A Okem, B Rama) pp. 1581–1688. (Cambridge University Press)
Lee JS, Cornwell WK, Kingsford RT (2022) Rainforest bird communities threatened by extreme fire. Global Ecology and Conservation 33, e01985.
| Crossref | Google Scholar |
Lemckert FL (2000) Observations on the effects of fire on the Hip-pocket Frog, Assa darlingtoni. Herpetofauna 30, 51-52.
| Google Scholar |
Lowe K, Castley JG, Hero J-M (2013) Acid frogs can stand the heat: amphibian resilience to wildfire in coastal wetlands of eastern Australia. International Journal of Wildland Fire 22, 947-958.
| Crossref | Google Scholar |
Mahony MJ, Hines HB, Lemckert F, Newell D, Roberts JD, Rowley JJL, Scheele BC, West M, Rumpff L, Legge SM, Van Leeuwen S, Wintle BA, Woinarski JCZ (2023) The impacts of the 2019-20 wildfires on Australian frogs. In ‘Australia’s megafires: biodiversity impacts and lessons from 2019-2020’. (Eds L Rumpff, SM Legge, S van Leeuwen, BA Wintle, JCZ Woinarski) pp. 167–181. (CSIRO Publishing) Available at www.publish.csiro.au
Mester B, Szalai M, Méro TO, Puky M, Lengyel S (2015) Spatiotemporally variable management by grazing and burning increases marsh diversity and benefits amphibians: a field experiment. Biological Conservation 192, 237-246.
| Crossref | Google Scholar |
Moreira LA, Fenolio DB, Silva HLR, da Silva NJ, Jr (2009) A preliminary list of the Herpetofauna from termite mounds of the cerrado in the Upper Tocantins river valley. Papéis Avulsos de Zoologia 49, 183-189.
| Crossref | Google Scholar |
Moritz MA, Parisien M-A, Batllori E, Krawchuk MA, van Dorn J, Ganz DJ, Hayhoe K (2012) Climate change and disruptions to global fire activity. Ecosphere 3, art49.
| Crossref | Google Scholar |
Nimmo DG, Avitabile S, Banks SC, Bliege Bird R, Callister K, Clarke MF, Dickman CR, Doherty TS, Driscoll DA, Greenville AC, Haslem A, Kelly LT, Kenny SA, Lahoz-Monfort JJ, Lee C, Leonard S, Moore H, Newsome TM, Parr CL, Ritchie EG, Schneider K, Turner JM, Watson S, Westbrooke M, Wouters M, White M, Bennett AF (2019) Animal movements in fire-prone landscapes. Biological Reviews 94, 981-998.
| Crossref | Google Scholar | PubMed |
Nolan RH, Boer MM, Collins L, Resco de Dios V, Clarke H, Jenkins M, Kenny B, Bradstock RA (2020) Causes and consequences of eastern Australia’s 2019–20 season of mega-fires. Global Change Biology 26, 1039-1041.
| Crossref | Google Scholar | PubMed |
Nomura F, Rossa-Feres DC, Langeani F (2009) Burrowing behavior of Dermatonotus muelleri (Anura, Microhylidae) with reference to the origin of the burrowing behavior of Anura. Journal of Ethology 27, 195-201.
| Crossref | Google Scholar |
Papp MG, Papp COG (2000) Decline of the population of the treefrog Phyllodytes luteolus after fire. Herpetological Review 31, 93.
| Google Scholar |
Pastro LA, Dickman CR, Letnic M (2014) Fire type and hemisphere determine the effects of fire on the alpha and beta diversity of vertebrates: a global meta-analysis. Global Ecology and Biogeography 23, 1146-1156.
| Crossref | Google Scholar |
Potvin DA, Parris KM, Smith Date KL, Keely CC, Bray RD, Hale J, Hunjan S, Austin JJ, Melville J (2017) Genetic erosion and escalating extinction risk in frogs with increasing wildfire frequency. Journal of Applied Ecology 54, 945-954.
| Crossref | Google Scholar |
R Core Team (2021) ‘R: a language and environment for statistical computing.’ (R Foundation for Statistical Computing) Available at https://www.r-project.org/
Rowley JJL, Callaghan CT (2020) The FrogID dataset: Expert-Validated occurrence records of Australia’s frogs collected by citizen scientists. ZooKeys 912, 139-151.
| Crossref | Google Scholar |
Rowley JJL, Callaghan CT, Cutajar T, Portway C, Potter K, Mahony S, Trembath DF, Flemons P, Woods A (2019) FrogID: citizen scientists provide validated biodiversity data on frogs of Australia. Herpetological Conservation and Biology 14, 155-170.
| Google Scholar |
Rowley JJL, Callaghan CT, Cornwell WK (2020) Widespread short-term persistence of frog species after the 2019–2020 bushfires in eastern Australia revealed by citizen science. Conservation Science and Practice 2, e287.
| Crossref | Google Scholar |
Thompson MM, Rowley JJL, Poore AGB, Callaghan CT (2022) Citizen science reveals meteorological determinants of frog calling at a continental scale. Diversity and Distributions 28, 2375-2387.
| Crossref | Google Scholar |
Ward M, Tulloch AIT, Radford JQ, Williams BA, Reside AE, Macdonald SL, Mayfield HJ, Maron M, Possingham HP, Vine SJ, O’Connor JL, Massingham EJ, Greenville AC, Woinarski JCZ, Garnett ST, Lintermans M, Scheele BC, Carwardine J, Nimmo DG, Lindenmayer DB, Kooyman RM, Simmonds JS, Sonter LJ, Watson JEM (2020) Impact of 2019–2020 mega-fires on Australian fauna habitat. Nature Ecology & Evolution 4, 1321-1326.
| Crossref | Google Scholar | PubMed |
White LA, Gibson RK (2022) Comparing fire extent and severity mapping between Sentinel 2 and Landsat 8 satellite sensors. Remote Sensing 14, 1661.
| Crossref | Google Scholar |
Wickham H (2017) tidyverse: Easily install and load the ‘tidyverse’. Available at https://cran.r-project.org/package=tidyverse
Williams RJ, Bradstock RA, Cary GJ, Enright NJ, Gill MA, Leidloff AC, Lucas C, Whelan R, Andersen AN, Bowman DMJS, Clarke PJ, Cook GD, Hennessy K, York A (2009) Interactions between climate change, fire regimes and biodiversity in Australia – a preliminary assessment. Report to the Department of Climate Change and Department of the Environment, Heritage and Arts, Canberra, Australia.
Wood SN (2017) ‘Generalized additive models: an introduction with R.’ 2nd edn. pp. 1–476. (Chapman and Hall/CRC) doi:10.1201/9781315370279