Fabaceae response to dimethoate insecticide application: investigation of germination, seedling growth and tolerance mechanisms
Karima Jalali


A
B
C
D
Abstract
Pesticides are widely used to control crop diseases and pest infestations. However, their excessive and unregulated use poses considerable environmental challenges.
To evaluate the effects of dimethoate on the germination and seedling growth of Vicia faba, V. sativa, Trigonella foenum-greacum, and Medicago truncatula.
Physiological and biochemical changes were studied under different dimethoate concentrations (0, 0.4, 0.8 and 1.2 g L−1).
Results showed that dimethoate significantly inhibited growth in all species, especially at 1.2 g L−1 of dimethoate. This negative effect was accompanied by reactive oxygen species (ROS) accumulation and lipid peroxidation (malondialdehyde, MDA) induction. Different behaviors were shown among the studied species. M. truncatula was the most sensitive, exhibiting toxicity even at the lowest concentration. In contrast, T. foenum-graecum showed remarkable tolerance, maintaining better growth at the highest dose compared to the two others species. The results of statistical analyses, including Pearson’s correlation and principal component analysis, support the use of fenugreek as a tolerant cover crop.
T. foenum-graecum’s tolerance was associated with a lower reduction in total soluble sugars (25%) and a significant increase in antioxidant enzyme activity, particularly superoxide dismutase (70%). These adaptations are likely to help T. foenum-graecum cope with oxidative stress and maintain growth despite pesticide exposure.
The results offer valuable decision support for using T. foenum-graecum as a cover crop in the field when using dimethoate in pest control at grapevine and citrus fields.
Keywords: antioxidant enzymes, cover crop, dimethoate, Fabaceae, SOD activity, stress, tolerance, total soluble sugar.
Introduction
Population growth has intensified food demand, driving the expansion of agricultural production and the widespread use of pesticides to sustain high crop yields (Lamichhane 2017; Strassemeyer et al. 2017). Without pesticides, the production of fruit, vegetables, and cereals would decline by 78%, 54%, and 32% respectively (Lamichhane 2017). Pesticides protect crops from pests and diseases, thereby increasing yields (Syafrudin et al. 2021; Angole et al. 2023). However, their uncontrolled use contaminates food, harms human health (Ghosh 2020) and degrades ecosystems (Sharma et al. 2019; Hashimi et al. 2020; Meena et al. 2020). One major consequence is soil degradation, because pesticide residues accumulate and disrupt soil health. Soil degradation not only results from pesticide accumulation but also from nutrient depletion, threatening agricultural sustainability. Soil acts as a pesticide sink, accumulating harmful chemicals that contribute to environmental degradation (Froger et al. 2023). Given its vital role in food security and environmental stability (Oh et al. 2014), preserving soil health is crucial for ecosystems and agriculture.
Plants rely on soil nutrients, especially nitrogen (N), which is essential for growth (Mahboob et al. 2023) and is mainly supplied through biological N fixation (BNF), a key agricultural and ecological process (Ampomah and Huss-Danell 2016). However, agrochemical-induced soil degradation can limit N availability, causing nutrient deficiencies (Hua et al. 2024; Quaggiotti et al. 2024). To mitigate N depletion, intercropping legumes within cropping systems and crop rotations enhances soil N through symbiosis with Rhizobium bacteria (Stagnari et al. 2017). This process improves soil fertility, while reducing reliance on pesticides (Yan et al. 2024) as well as on the use of synthetic N fertilizers (Xiao et al. 2018; Yang et al. 2022). When legumes are intercropped as cover crops, they further enhance agricultural sustainability, improving soil health, promoting nutrient sequestration and increasing water retention, all of which contribute to higher crop productivity (Stefan et al. 2021; Jalloh et al. 2023, 2024; Ramírez-Parra and De la Rosa 2023).
Among pesticides, dimethoate [active ingredient: dimethoate; C5H12NO3PS2; O,O-dimethyl S-methyl carbamoylmethyl phosphorodithioate; trade name: Dimethoate] (Singh et al. 2014) is recognized as one of the most widely applied systemic organophosphate insecticide (Martinuzzi et al. 2020). It is extensively used to control various plant diseases and insect pests (Oladapo et al. 2021). Dimethoate acts as an acetylcholinesterase inhibitor (AChE), causing nerve damage and organism death (Pawar et al. 2020).
In Mediterranean countries, such as Tunisia, Spain, and Italy, dimethoate is commonly used to protect olive cultivation (Tsatsakis and Tsakiris 2010). In Tunisia, its application extends beyond olive trees to also the control of diseases in vineyards and citrus, both of which are economically important crops. Its widespread use is informed by stakeholder surveys and informal discussions with farmers in the Cape Bon region, the country’s primary area for citrus and grapevine cultivation.
This agrochemical can negatively affect plant germination, growth, and photosynthetic pigments (Pandey and Kanaujia 2010; Mishra et al. 2011; Shakir et al. 2018; Singh et al. 2023), and can lead to the generation of reactive oxygen species (ROS), triggering oxidative stress that causes damage to vital macromolecules, including proteins, lipids, and DNA (Shakir et al. 2018; Hatamleh et al. 2022; Singh et al. 2023). Plants counteract this damage with antioxidant enzymes such as superoxide dismutase (SOD), catalase (CAT) and guaiacol peroxidase (GPOX), which play a crucial role in mitigating pesticide-induced oxidative stress (Jalali et al. 2018; Hatamleh et al. 2022). This study aimed to identify legume species suitable as soil cover in grapevine and citrus fields in Tunisia’s Cap Bon region, where dimethoate is being frequently applied. It evaluated the effect of the pesticide on the germination, growth, and stress responses of four legumes, namely, V. faba, V. sativa, T. foenum-graecum, and M. truncatula, so as to assess their tolerance and defense mechanisms.
Materials and methods
Plant materials
This study was conducted using Vicia faba L. (var. Saber 02), Trigonella foenum-graecum L., Vicia sativa L., and Medicago truncatula (var. Jemalong A17).
Pesticide treatments
To assess the effects of commonly used pesticide dimethoate on V. faba, T. foenum-graecum, V. sativa and M. truncatula legumes, three different concentrations of dimethoate were prepared.
The tested concentrations of dimethoate were the recommended dose (0.4 g L−1), two times higher than the recommended dose (0.8 g L−1) and three times higher than the recommended dose (1.2 g L−1).
Seed germination and treatment
Seeds of V. faba, V. sativa, and T. foenum-graecum were surface-sterilized with 0.1% mercuric chloride (HgCl2) solution for 1 min. M. truncatula seeds were additionally chemically scarified with sulfuric acid (H2SO4, 95%). All seeds were then thoroughly rinsed three times with sterile distilled water and pre-soaked for 3 h in distilled water. Following pre-soaking, seeds of each species were divided into four treatment groups, namely, distilled water (control) or dimethoate solutions at concentrations of 0.4, 0.8, and 1.2 g L−1.
For the germination test, 20 seeds of each species were evenly placed in Petri dishes (90-mm diamater) lined with two layers of moistened filter paper, with six replicates per treatment. Each dish received 20 mL of either distilled water (control) or the corresponding dimethoate solution. Dishes were incubated in a growth chamber at 25 ± 1°C in the dark for 5 days. Filter papers were kept moist by adding the appropriate treatment solution as needed. A seed was considered germinated when the radicle reached 2 mm in length. Germination percentage was recorded for each treatment after 5 days by using the following formula: (germinated seeds × 100) / total seeds planted.
Seedling growth and dimethoate treatment
Germinated seeds were transferred to square plastic Petri dishes (120 × 120 × 17 mm; Greiner) filled with sterilized sand moistened with 20 mL of either distilled water or dimethoate solution. The seeds were covered with filter paper imbibed with the respective solution to ensure uniform exposure. The experiment was conducted under the following controlled environmental conditions: temperature, 25 ± 1°C; relative humidity, 60–70%; and a photoperiod of 16 h light and 8 h dark. For each treatment, six replicates were used. Each replicate consisted of 20 seeds per species. Seedling length was measured manually with a ruler. On Day 10, the seedlings were harvested and divided into two portions, namely, one for fresh biomass determination and the other for radicle length measurement, whereas the second portion was frozen in liquid N and stored at −80°C for physiological and biochemical analyses. Additionally, a subset of the samples was oven-dried at 60°C for 96 h until a constant weight was achieved, and, subsequently, ground for the determination of total soluble sugar content.
Determination of total soluble sugar content (TSS)
Total soluble sugars were determined by the anthrone sulfuric acid reagent by using L-glucose for the standard curve (Yemm and Willis 1954).
Dry root tissues (25 mg) were homogenized with 5 mL of 80% (v/v) ethanol, incubated at 70°C and centrifuged for 30 min at 3000g, twice. The amount is expressed as milligrams per gram dry weight (mg g−1 DW) through the calibration curve.
Determination of lipid peroxidation (malondialdehyde, MDA)
For MDA content analysis, fresh root tissues (0.5 g) were crushed and homogenized with 1 mL of 5% trichloroacetic acid (TCA) solution (Karabal et al. 2003). The homogenate was then centrifuged at 10.000g for 15 min at 4°C. Next, a 1 mL aliquot of the supernatant was added to 1 mL of a solution containing 20% TCA and 0.5% thiobarbituric acid (TBA). This mixture was incubated at 95°C for 30 min and then cooled immediately in an ice bath. After cooling and centrifuging at 10.000g for 5 min, the specific absorbance of extracts was recorded at 532 nm. Non-specific absorption at 600 nm was recorded at 600 nm and then subtracted from the 532 nm readings. MDA concentration was quantified using the extinction coefficient of 155 mM−1 cm−1.
Extraction of total protein content and determination of antioxidant enzyme activities
Proteins and various antioxidant enzyme activities were investigated for biochemical analysis. Protein content was determined using bovine serum albumin as a standard (Bradford 1976). Fresh tissues (0.5 g) were ground at 4°C in mortar with 2% (w/w) polyvinyl-polypyrrolidone (PVP) and 1 mL phosphate buffer (50 mM; pH 7.8) containing 0.2 mM ethylenediaminetetraacetic (EDTA), 1 mM phenylmethylsulfonyl fluoride (PMSF), as a protease inhibitor, and 0.1% (v/v) Triton X-100. The extract was centrifuged at 12.000g for 20 min at 4°C and the supernatant was used to determine enzyme activities.
Superoxide dismutase (SOD) activity was assessed using the photochemical nitrobluetetrazolium chloride (NBT) (Beauchamp and Fridovich 1971). The reaction mixture contained 50 mM K2HPO4/KH2PO4 buffer (pH 7.8), 2 mM riboflavin, 13 mM methionine, 75 mM NBT and enzyme extract. One unit of SOD activity was expressed as the amount of enzyme required to inhibit the reduction rate of NBT by 50% at 25°C.
Catalase (CAT) activity was measured by following the decline of absorbance at 240 nm caused by the decomposition of H2O2 during 1 min (ε = 36 M−1 cm−1) (Anderson et al. 1995). The assay mixture was composed of 50 mM potassium phosphate buffer (pH 7.0), 10 mM H2O2, and 5 μL extract in a total volume of 1 mL.
The activity of GPOX was assessed by the measurement of the kinetic evolution of tetraguaiacol formation from guaiacol (9 mM) at 470 nm during 1 min (ε = 26.6 mM−1 cm−1) in the presence of 19 mM H2O2, which was added to initiate the reaction (Anderson et al. 1995).
Statistical analysis
All statistical analyses were conducted using R (ver. 4.3.2; R Core Team 2023). Linear models were fitted for each response variable by using the stats package in R, with species (S), treatment (T), and their interaction (T:S) as fixed effects. Model assumptions were evaluated through the Shapiro–Wilk test of residual normality (Shapiro and Wilk 1965) and Levene’s tests of variance homogeneity (Levene 1960), with both being implemented in the rstatix package (ver. 0.7.2, https://cran.r-project.org/web/packages/rstatix/index.html). These assumptions were met for all variables except germination percentage (GP). Significant effects in two-way ANOVA (α = 0.05) were followed by post hoc pairwise comparisons by using Tukey-adjusted P-values for contrasts via the emmeans package (ver. 1.9.0) (Lenth 2023). The multcomp package (ver. 1.4) (Hothorn et al. 2008) was used to generate compact letter displays for all pairwise comparisons. For GP, we performed aligned rank transformation ANOVA by using the ARTool package (ver. 0.11.1) (Kay et al. 2021), followed by ART-C contrasts (Elkin et al. 2021) for post hoc testing. Results showed that the T:S effect was significant for all studied variables (Table 1). Correlation analyses were conducted using Pearson’s product–moment correlation (Pearson 1895) for normally distributed variables and Spearman’s rank correlation (Spearman 1904) for analyses involving GP, with P-values adjusted using Bonferroni correction. The resulting correlation matrix was visualized using the corrplot package (ver. 0.95) (Wei and Simko 2021). Multivariate patterns were examined through principal component analysis using FactoMineR (ver. 2.11) (Lê et al. 2008), with results being visualized via factoextra (ver. 1.0.7) (Kassambara and Mundt 2020). All graphical representations were created using ggplot2 (ver. 3.5.1) (Wickham 2016), with color optimization through the colorspace package (ver. 2.1-1) (Zeileis et al. 2020).
Item | GP | RL | RDW | TSS | MDA | SOD | CAT | GPOX | |
---|---|---|---|---|---|---|---|---|---|
Treatment (T) | 47.37*** | 600.52*** | 48.32*** | 881.85*** | 152.83*** | 226.68*** | 1422.06*** | 1132.15*** | |
Species (S) | 56.30*** | 147.37*** | 3.57* | 96.34*** | 30.96*** | 509.97*** | 2.63n.s. | 1927.15*** | |
T:S | 7.34*** | 15.96*** | 3.76** | 66.24*** | 2.21* | 2.17* | 21.21*** | 60.65*** |
GP, germination percentage (%); RL, root length (cm); RDW, root dry weight (mg), TSS, total soluble sugar (mg g−1 DW); MDA, malondialdehyde content (μmol g−1 FW); SOD, superoxide dismutase activity (USOD mg−1 proteins), CAT, catalase activity (μmol H2O2 min−1 mg−1 proteins) and GPOX, guaiacol peroxidase activity (nmol H2O2 min−1 mg−1 proteins).
*P < 0.05; **P < 0.01; ***P < 0.001; n.s., non-significant, P > 0.05.
Results
Effects of dimethoate on seed germination and root length
The germination percentage of all tested species progressively declined with an increasing dimethoate concentration, with M. truncatula exhibiting complete inhibition across all tested concentrations. This extreme sensitivity during germination not only caused a drastic reduction in its germination rates but also necessitated its exclusion from subsequent seedling stage analyses due to its markedly poor performance.
Under control conditions (0 g L−1) and at 0.4 g L−1 dimethoate, both T. foenum-graecum and V. sativa exhibited germination rates greater than 90%. In contrast, V. faba did not achieve 80% germination at the same concentration of 0.4 g L−1 dimethoate (Fig. 1a).
Effect of dimethoate on (a) germination percentage, (b) root dry weight, (c) radicle length, and (d) total soluble sugar content in 10-day-old T. foenum-graecum, V. faba, and V. sativa seedlings. (a) Boxplots represent the median of six replicates, with error bars indicating the 95% confidence interval of the median. (b–d) Boxplots display the median (horizontal line), interquartile range (box), individual data points (six replicates), and means (diamonds). Groups sharing the same letter are not significantly different (Tukey-adjusted contrasts, α = 0.05).
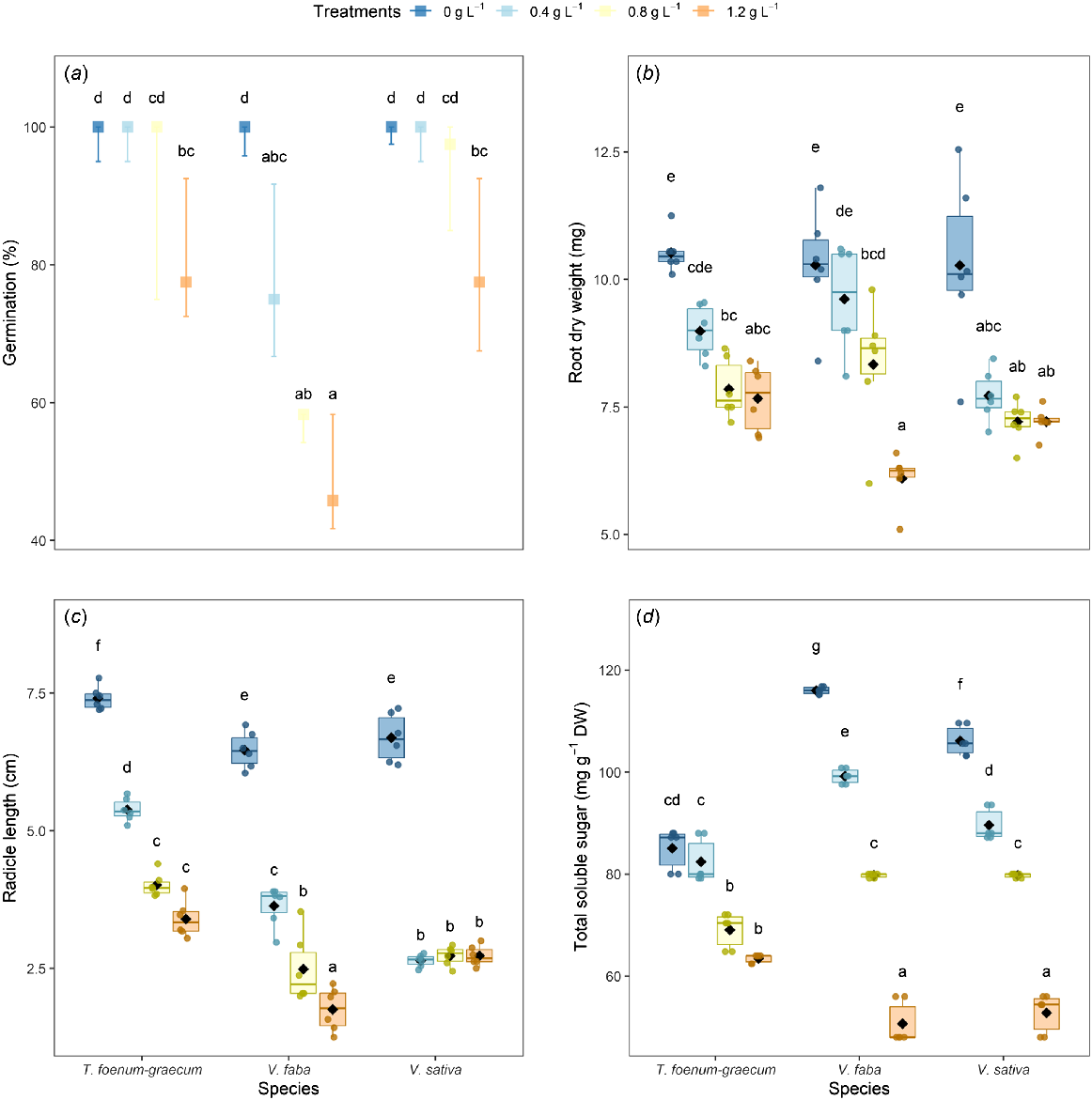
At the highest dimethoate concentration of 1.2 g L−1, T. foenum-graecum showed the least significant reduction in germination percentage (17%), followed by V. sativa (20%), whereas V. faba showed a more significant reduction of 50% than did the respective control groups.
In terms of seedling growth, specifically root length and root dry weight, our results showed that dimethoate induced a significant reduction in these parameters in all species, especially at the higher concentration of 1.2 g L−1. The least reduction in root elongation and root dry weight was observed in T. foenum-graecum, with decreases of 53% and 27% respectively, compared with the control. In comparison, V. sativa showed reductions of 59% and 30%, and V. faba showed the most pronounced reductions of 72% and 40% respectively (Fig. 1b, c).
Effects of dimethoate on total soluble sugar content (TSS)
TSS showed a progressive decrease with an increasing dimethoate concentration (Fig. 1d). The degree of reduction was species-dependent, highlighting differences in metabolic resistance to pesticide-induced stress. In T. foenum-graecum, TSS decreased by 18% at 0.8 g L−1 and by 25% at 1.2 g L−1, indicating a relatively higher tolerance to dimethoate. Conversely, V. faba showed a marked sensitivity with a 31% decrease in TSS at 0.8 g L−1 and a 56% decrease at 1.2 g L−1. Similarly, V. sativa showed a reduction of 24% at 0.8 g L−1 and 50% at 1.2 g L−1.
Effects of dimethoate on lipid peroxidation
One of the major biological processes associated with the activity of reactive oxygen species (ROS) is lipid peroxidation, which serves as an indicator of oxidative stress. MDA, a primary by-product of lipid peroxidation and a major metabolite that reacts with thiobarbituric acid (TBA), was significantly elevated in the roots of all three legume species exposed to dimethoate, particularly at the higher concentrations of 0.8 g L−1 and 1.2 g L−1 (Fig. 2a).
Effect of dimethoate on (a) malondialdehyde (MDA) content, and the activities of (b) superoxide dismutase (SOD), (c) catalase (CAT), and (d) guaiacol peroxidase (GPOX) in the roots of 10-day-old T. foenum-graecum, V. faba, and V. sativa seedlings. (a–d) Boxplots show the median (horizontal line), interquartile range (box), individual data points (six replicates), and means (diamonds). Groups sharing the same letter are not significantly different (Tukey-adjusted contrasts, α = 0.05).
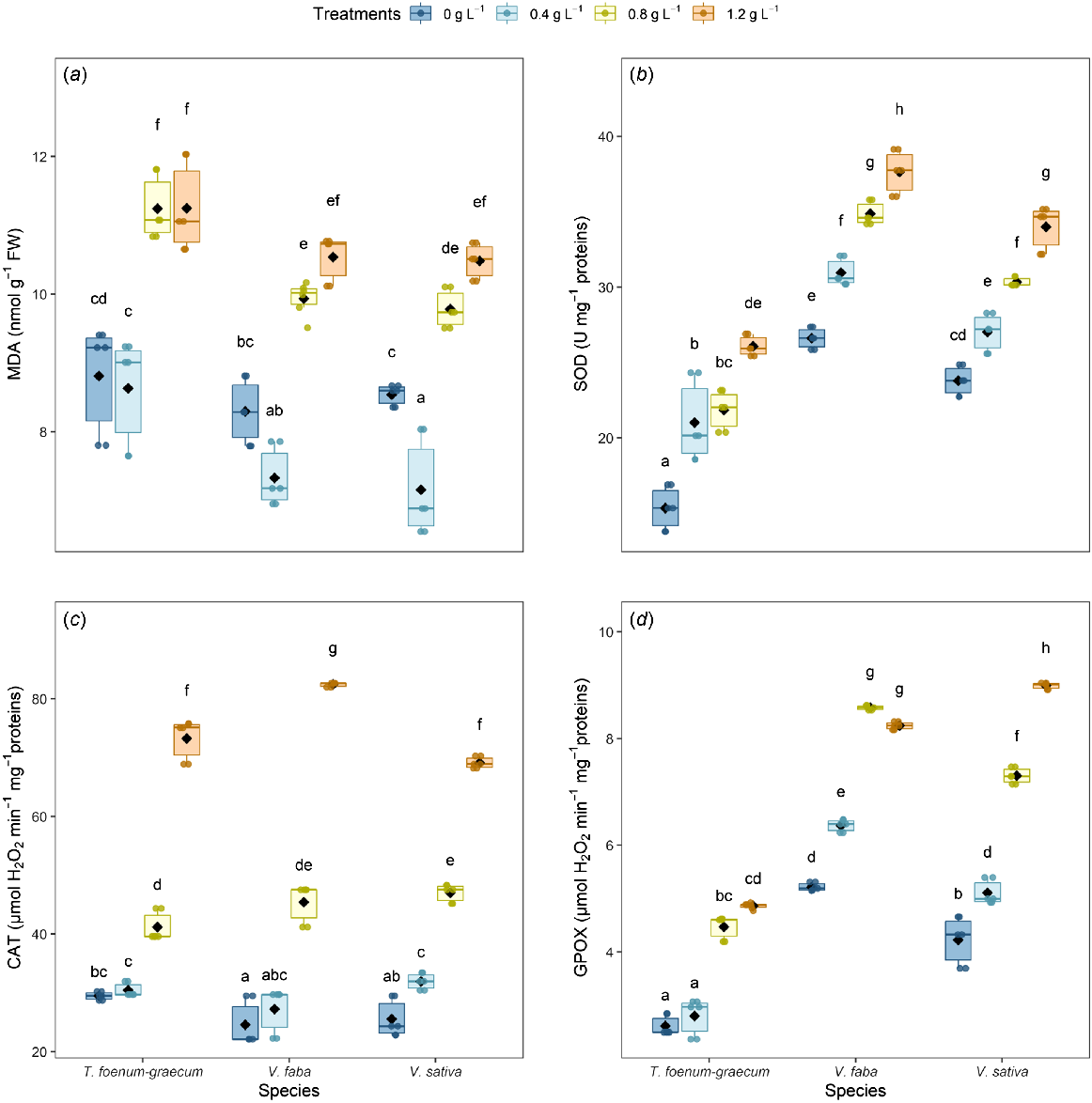
At a lower concentration (0.4 g L−1), MDA concentration showed a slight decrease in V. faba and V. sativa, while remaining stable in T. foenum-graecum compared with the control. However, at the highest concentration of dimethoate (1.2 g L−1), an increase in MDA content was observed, with increases of 35%, 29% and 35% in V. faba, V. sativa and T. foenum-graecum respectively.
Antioxidant enzymes variation in dimethoate-treated seedlings
Antioxidant enzymes are crucial for the mitigation of oxidative stress. At the seedling stage, the activities of SOD, CAT and GPOX in the three legume species studied showed a significant increase in response to increasing concentrations of dimethoate (Fig. 2b–d). Specifically, at a concentration of 0.4 g L−1, only minor variations were observed in all activities tested for the three species, with the exception of SOD in T. foenum-graecum, which showed a significant induction of about 37%. At the highest concentration of 1.2 g L−1, SOD activity was significantly stimulated (Fig. 2b), with T. foenum-graecum showing an increase of 70%, compared with increases of 41% and 43% in V. faba and V. sativa respectively. In addition, CAT and GPOX activities were significantly increased compared with their controls (Fig. 2c, d), with the most pronounced increase in CAT activity being observed in V. faba, which showed a more than three-fold increase, whereas GPOX activity was significantly higher in V. sativa.
Pearson correlation analysis of growth, and physiological and biochemical traits of V. faba, V. sativa and T. foenum-graecum
The Pearson correlation analysis results showed that all investigated growth parameters (GP, RL and RDW) exhibited a strong positive correlation among themselves. Nevertheless, GP and RL were negatively correlated with SOD (r = −0.7 and r = −0.78 respectively), GPOX (r = −0.67 and r = −0.76 respectively) and CAT (r = −0.63 and r = −0.66 respectively) activities. Additionally, TSS was highly positively correlated with GP (r = 0.52), RL (r = 0.63) and RDW (r = 0.71) and negatively correlated with MDA concentration (r = −0.76), and SOD, GPOX and CAT activities (r = −0.37, r = −0.43 and r = −0.87 respectively) (Supplementary Fig. S1).
Principal component analysis (PCA)
To evaluate the pesticide tolerance capacity of V. faba, V. sativa, and T. foenum-graecum, we conducted a PCA on eight studied variables measured at each dimethoate concentration (0 g L−1, 0.4 g L−1, 0.8 g L−1, and 1.2 g L−1). The PCA results indicated that the first two principal components (PCs) explained more than 66% of the total variance across all treatment levels (Fig. 3a–c). The correlations between the investigated variables and the PCs are detailed in Table 2.
Principal component analysis (PCA) of traits in T. foenum-graecum, V. faba, and V. sativa seedlings under dimethoate treatments: 0.4 g L−1 (a), 0.8 g L−1 (b), and 1.2 g L−1 (c). GP, germination percentage (%); RL, root length (cm); DW, root dry weight (mg); TSS, total soluble sugars (mg g−1 DW); MDA, malondialdehyde content (μmol g−1 FW); SOD, superoxide dismutase activity (U SOD mg−1 proteins); CAT, catalase activity (μmol H2O2 min−1 mg−1 proteins); GPOX, guaiacol peroxidase activity (nmol H2O2 min−1 mg−1 proteins).
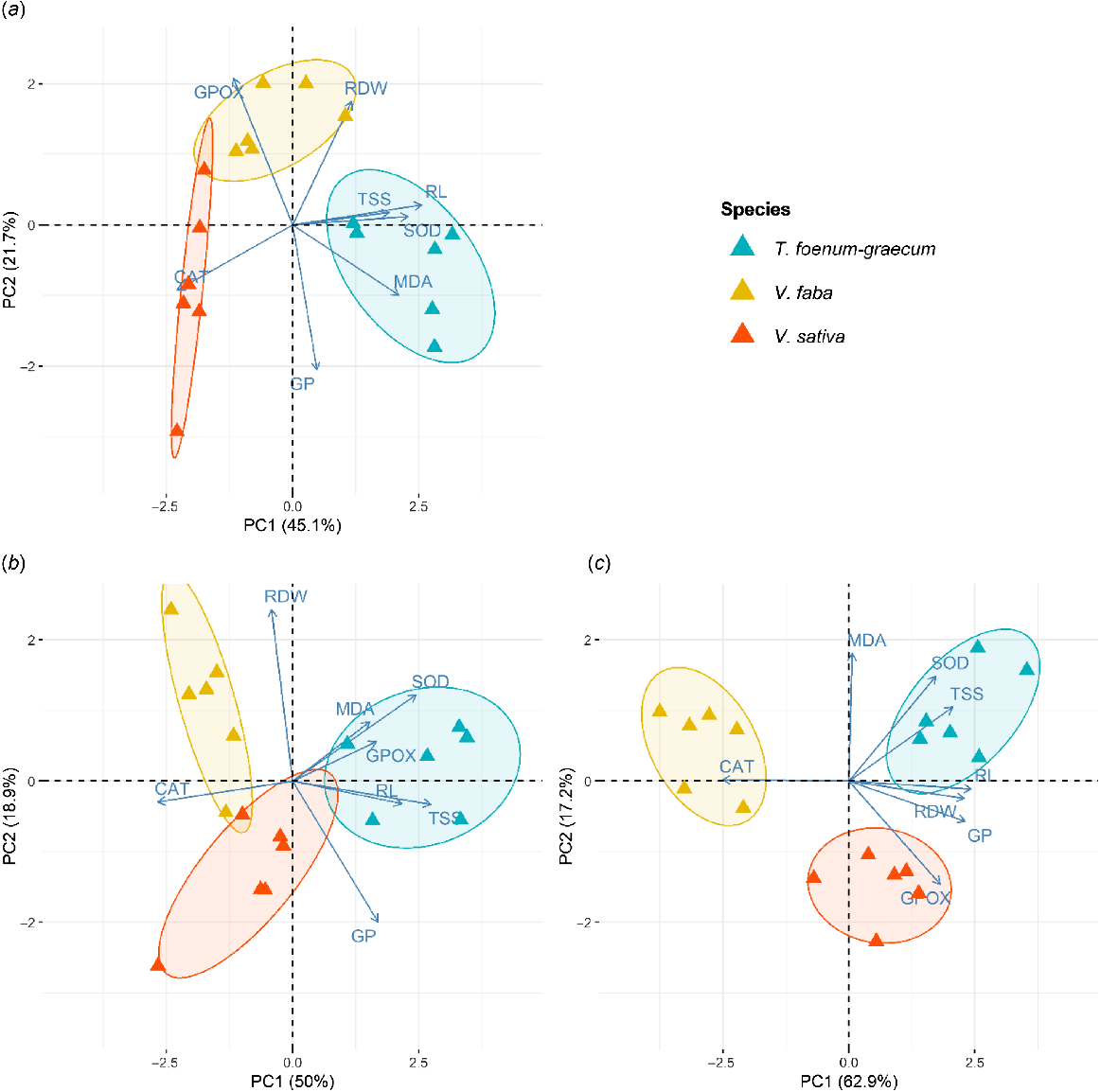
Variable | PC1 correlation | PC2 correlation | |||||||||||
---|---|---|---|---|---|---|---|---|---|---|---|---|---|
0.4 g L−1 | 0.8 g L−1 | 1.2 g L−1 | 0.4 g L−1 | 0.8 g L−1 | 1.2 g L−1 | ||||||||
r | P | r | P | r | P | r | P | r | P | r | P | ||
GP | – | – | 0.58 | 0.01 | 0.89 | <0.001 | −0.73 | <0.001 | −0.68 | 0.001 | – | – | |
RL | 0.91 | <0.001 | 0.74 | <0.001 | 0.94 | <0.001 | – | – | – | – | – | – | |
RDW | – | – | – | – | 0.89 | <0.001 | 0.62 | 0.005 | 0.83 | <0.001 | – | – | |
TSS | 0.68 | 0.001 | 0.94 | <0.001 | 0.80 | <0.001 | – | – | – | – | – | – | |
MDA | 0.75 | 0.003 | 0.52 | 0.02 | – | – | – | – | – | – | 0.70 | 0.001 | |
SOD | 0.82 | <0.001 | 0.84 | <0.001 | 0.67 | 0.002 | – | – | – | – | 0.57 | 0.01 | |
CAT | −0.82 | <0.001 | −0.92 | <0.001 | −0.97 | <0.001 | – | – | – | – | – | – | |
GPOX | – | – | 0.57 | 0.01 | 0.70 | 0.001 | 0.74 | <0.001 | – | – | −0.56 | 0.01 |
GP, germination percentage (%); RL, root length (cm); RDA, root dry weight (mg); TSS, total soluble sugar (mg g−1 DW); MDA, malondialdehyde content (μmol g−1 FW); SOD, superoxide dismutase activity (USOD mg−1 proteins); CAT, catalase activity (μmol H2O2 min−1 mg−1 proteins); and GPOX, guaiacol peroxidase activity (nmol H2O2 min−1 mg−1 proteins).
At the lowest dimethoate concentration (0.4 g L−1), the PCA biplot explained 66.8% of the total variance. The first axis (PC1 = 45.1%) showed strong correlations with TSS, root RL, and SOD activity, and the second axis (PC2 = 21.7%) was associated with CAT and GPOX activities. Analysis of the score plot showed three distinct groups of species seedlings. Group 1, situated on the right side of the score plot, positively correlated with PC1 and negatively with PC2, and consisted of T. foenum-graecum, characterized by the highest TSS content, SOD induction, and RL. Group 2 was positioned at the upper side of the score plot, negatively correlated with PC1 and positively with PC2, comprising V. faba, which was distinguished by the highest GPOX activity and the lowest GP. Group 3, located on the left side of the score plot, included V. sativa, marked by the highest induction of CAT and the lowest RDW.
At the highest dimethoate concentration (1.2 g L−1), the PCA biplot explained 80.1% of the total variance. Analysis of the score plot showed three groups. Group 1, in the upper-right quadrant, positively correlated with PC1 and negatively with PC2, included T. foenum-graecum, which displayed the highest TSS concentrations, RL, SOD increase, as well as improved GP and RDW. Group 2, located on the left side of the score plot, consisted of V. faba, which exhibited the highest increase in CAT activity. Finally, Group 3, positioned in the lower right quadrant, comprised V. sativa, distinguished by the highest GPOX activity.
Overall, the PCA analysis demonstrated a clear separation among the investigated species. Notably, the biplots for each treatment, even at the highest dimethoate concentration, indicated that T. foenum-graecum was consistently located on the right side of PC1, exhibiting superior values for TSS, SOD activity, and growth indices. This legume displayed greater tolerance to pesticide-induced stress compared to V. faba and V. sativa.
Discussion
In this study, our results demonstrated that dimethoate treatment significantly reduced germination percentage across tested legume species, with M. truncatula exhibiting extreme sensitivity. Germination was completely inhibited at all tested concentrations, highlighting its vulnerability during early developmental stages. This complete germination failure necessitated its exclusion from further seedling-stage analyses because of poor performance. For the other species, dimethoate exhibited toxicity at concentrations ranging from 0.8 g L−1 to 1.2 g L−1, further emphasizing the potential risks of excessive pesticide exposure to non-target plants.
Our findings regarding the reduction in biometric and growth parameters observed in V. faba and V. sativa, and to a lesser degree in T. foenum-graecum, are consistent with findings from similar studies showing that various pesticides inhibit germination in species such as Lycopersicon esculentum and Zea mays (Coskun et al. 2015; Shakir et al. 2016). We observed a significant decrease in root length and dry weight as the dimethoate concentration increased, an effect previously noted in species such as Solanum melongena and L. esculentum (Singh et al. 2023) and Momordica charantia (Mishra et al. 2009). To explain the adverse effects of pesticides on seedlings and plant growth, several hypotheses have been proposed. For example, the observed decrease in germination and growth parameters at high dimethoate concentrations may result from one of the primary toxic effects of this insecticide, which can disrupt essential physiological and biochemical processes (Mishra et al. 2009). Additionally, pesticides can inhibit enzymes such as hydroxyl phenyl pyruvate dehydrogenase, essential for cell division and expansion in meristematic tissues (Parween et al. 2011; Yildiztekin et al. 2015).
A key consequence of pesticide-induced stress is the reduction in total soluble sugars (TSS), as observed in our study, particularly in root tissues at the highest dimethoate dose of 1.2 g L−1. Similar reductions have been reported for pesticides such as diazinon, imidacloprid, and mancozeb (Hatamleh et al. 2022), copper sulfate (Jalali et al. 2018), emamectin, alpha-cypermethrin, imidacloprid, and lambda-cyhalothrin (Shakir et al. 2016). The decline is likely to result from impaired chlorophyll biosynthesis, reducing photosynthetic efficiency and sugar production (Hatamleh et al. 2022).
Malondialdehyde (MDA), a lipid peroxidation marker, indicates oxidative damage from pesticide exposure (Shingote et al. 2020). Our study confirmed increased MDA concentration in all species under dimethoate treatment, consistent with reports linking organophosphate pesticides to early membrane damage (Srivastava et al. 2014). Similar increases were observed in Triticum aestivum after chlorsulfuron exposure and in M. charantia leaves on dimethoate treatment (Song et al. 2007; Mishra et al. 2009), S. melongena, and L. esculentum after dimethoate and chlorpyrifos exposure (Singh et al. 2023), highlighting its oxidative impact on plant tissues.
Plants counter oxidative stress through an antioxidant defense system that mitigates ROS-induced damage (Hatamleh et al. 2022). Key enzymes such as SOD, CAT, and GPOX play a crucial role in scavenging ROS (Singh et al. 2023). In our study, their activities significantly increased across all species, especially at the highest dimethoate concentration, reflecting a strong antioxidant response. This aligns with previous findings on pesticide-induced enzymatic upregulation (Fatma et al. 2018; Jalali et al. 2018; Hatamleh et al. 2022; Singh et al. 2023) and highlights the importance of antioxidant defenses in improving dimethoate tolerance.
Furthermore, our results showed that T. foenum-graecum exhibited greater tolerance to dimethoate contamination at the seedling stage than did V. faba and V. sativa. This superior resilience was associated with a smaller reduction in TSS content and a more pronounced induction of antioxidant enzymes, particularly SOD. These findings are consistent with numerous studies showing the ability of fenugreek T. foenum-graecum to germinate and thrive under harsh conditions, including heavy metal exposure (Elleuch et al. 2013; Melki et al. 2022), and drought stress (Sadeghzadeh-ahari et al. 2010). Therefore, T. foenum-graecum represents a promising candidate for use as a cover crop, capable of promoting growth even in challenging environments.
Recent studies further support this potential, demonstrating that intercropping Hordeum vulgare with T. foenum-graecum increased total biomass, protein content, and carbohydrate content (Bitarafan et al. 2019; Toukabri et al. 2021). PCA analyses provided a comprehensive summary of the data and corroborated these findings, namely T. foenum-graecum showed the least reduction in germination, root growth and TSS content, together with the highest SOD activity, particularly at high dimethoate concentrations.
The choice of dimethoate concentrations (0.4 g L−1) in this study was based on stakeholder surveys and informal discussions with farmers in the Cape Bon region, Tunisia’s primary citrus and grapevine cultivation area. To assess plant responses under field-relevant conditions, we tested the recommended dose of dimethoate (0.4 g L−1), as well as twice (0.8 g L−1) and three times (1.2 g L−1) the recommended dose. The majority of Tunisian farmers do not strictly adhere to good phytosanitary practices, particularly in terms of the number of applications per agricultural season, treatment frequency, and the pre-harvest interval (Bouagga and Chaabane 2015; Bouagga et al. 2019a). For instance, Tunisian vine growers primarily rely on chemical control for managing pests such as insects, mites, and phytopathogenic fungi, with pesticide applications ranging from 10 to 24 per season (Bouagga et al. 2019b).
Given this agricultural context, pesticide applications in Tunisian viticulture and citrus orchards are predominantly preventive, often being applied before disease establishment. Farmers frequently adjust the frequency and dosage of treatments based on climatic conditions and pest pressure to maintain optimal crop protection. However, due to the economic and strategic importance of vineyards and citrus orchards, this intensive pesticide use has led to residue accumulation that frequently exceeds established maximum residue limits (MRLs). Within this framework, the concentrations tested in our study, 0.8 g L−1 (twice the recommended dose) and 1.2 g L−1 (three times the recommended dose) are representative of the exposure levels that plants commonly experience in real agricultural settings.
On the basis of our plant responses, T. foenum-graecum demonstrated the highest resilience to dimethoate contamination, maintaining superior growth and higher TSS content than did V. faba and V. sativa. At the recommended application rate of dimethoate (0.4 g L−1), all species germinated successfully with minimal reductions in growth and TSS concentration; meanwhile, T. foenum-graecum exhibited the strongest tolerance. Its enhanced SOD activity is likely to contribute to its superior stress tolerance. At higher dimethoate concentrations (0.8–1.2 g L−1), growth reductions became more pronounced across all species, yet T. foenum-graecum maintained better performance than did V. faba and V. sativa. Its resilience appears to be due to a robust antioxidant defense and minimal TSS reduction, helping mitigate oxidative stress and maintain physiological stability. Whereas over-application of dimethoate should be avoided because of its detrimental effects on both the environment and crop growth, T. foenum-graecum remains a viable option in fields where elevated concentrations of dimethoate residues are unavoidable. Overall, our findings suggest that T. foenum-graecum might constitute the relevant choice for farmers who usually use the dimethoate treatment.
Conclusions
This study concludes that all tested concentrations of dimethoate are highly toxic to M. truncatula seeds, even at the lowest dose. Consequently, this species was excluded from our study. At higher concentrations of dimethoate, T. foenum-graecum successfully germinated and exhibited a slight reduction in plant growth and TSS content compared with V. faba and V. sativa. This tolerance to dimethoate is likely to be associated with the enhanced activity of antioxidant enzyme systems, particularly SOD. Furthermore, PCA results support the selection of T. foenum-graecum seedlings as tolerant to dimethoate contamination.
On the basis of our results, discussed with other reports, we can conclude that fenugreek seedlings could be a suitable candidate for use as a cover crop in fields where dimethoate is applied for pest control, such as grapevine and citrus fields.
Moreover, the observed increase in SOD activity may serve as an early indicator of an adaptive response to chemical stress. Future research is still required to investigate the mechanisms of insecticide tolerance/sensitivity (molecular study of antioxidant response) and the possibility of using tolerant plants for animal diets (evaluating the contamination risks within harvested plants).
Data availability
Data sharing is not applicable because no new data were generated or analyzed during this study.
References
Ampomah OY, Huss-Danell K (2016) Genetic diversity of rhizobia nodulating native Vicia spp. in Sweden. Systematic and Applied Microbiology 39, 203-210.
| Crossref | Google Scholar |
Anderson MD, Prasad TK, Stewart CR (1995) Changes in isozyme profiles of catalase, peroxidase, and glutathione reductase during acclimation to chilling in mesocotyls of maize seedlings. Plant Physiology 109, 1247-1257.
| Crossref | Google Scholar |
Angole M, Malinga GM, Tanga CM, Subramanian S, Cheseto X, Egonyu JP (2023) Effect of desert locust control on non-target edible termites in eastern Uganda. Journal of Insects as Food and Feed 9, 1235-1246.
| Crossref | Google Scholar |
Beauchamp C, Fridovich I (1971) Superoxide dismutase: improved assays and an assay applicable to acrylamide gels. Analytical Biochemistry 44, 276-287.
| Crossref | Google Scholar |
Bitarafan Z, Jensen SM, Liu F, Andreasen C (2019) Intercropping fenugreek (Trigonella foenum-graecum L.) and barley (Hordeum vulgare L.) with and without biochar: tests along a competition gradient. Journal of Agronomy and Crop Science 205, 99-107.
| Crossref | Google Scholar |
Bouagga A, Chaabane H, Toumi K, Mougou Hamdane A, Nasraoui B, Joly L (2019a) Pesticide residues in Tunisian table grapes and associated risk for consumer’s health. Food Additives and Contaminants: Part B Surveillance 12, 135-144.
| Crossref | Google Scholar | PubMed |
Bouagga A, Chtioui W, Nasraoui B, Chaabane H (2019b) Pest management knowledge and practices of grapevine farmers in northern of Tunisia. Journal of New Sciences 67(2), 4191-4197.
| Google Scholar |
Bradford MM (1976) A rapid and sensitive method for the quantification of microgram quantities of protein utilizing the principle of protein-dye binding. Analytical Biochemistry 72, 248-254.
| Crossref | Google Scholar |
Coskun Y, Kilic S, Duran RE (2015) The effects of the insecticide pyriproxyfen on germination, development and growth responses of maize seedlings. Fresenius Environmental Bulletin 24, 278-284.
| Google Scholar |
Elkin LA, Kay M, Higgins JJ, Wobbrock JO (2021) An aligned rank transform procedure for multifactor contrast tests. In ‘UIST 2021 – Proceedings of the 34th Annual ACM Symposium on User Interface Software and Technology’. (Association for Computing Machinery: New York, NY, USA) 10.1145/3472749.3474784
Elleuch A, Chaâbene Z, Grubb DC, Drira N, Mejdoub H, Khemakhem B (2013) Morphological and biochemical behavior of fenugreek (Trigonella foenum-graecum) under copper stress. Ecotoxicology and Environmental Safety 98, 46-53.
| Crossref | Google Scholar | PubMed |
Fatma F, Kamal A, Srivastava A (2018) Exogenous application of salicylic acid mitigates the toxic effect of pesticides in Vigna radiata (L.) Wilczek. Journal of Plant Growth Regulation 37, 1185-1194.
| Crossref | Google Scholar |
Froger C, Jolivet C, Budzinski H, Pierdet M, Caria G, Saby NPA, Arrouays D, Bispo A (2023) Pesticide residues in french soils: occurrence, risks, and persistence. Environmental Science and Technology 57, 7818-7827.
| Crossref | Google Scholar |
Ghosh SK (2020) Evaluation of safe insecticides against sucking pests, jassid (Amrasca bigutula bigutula Ishida) and aphid (Aphis gossypii Glov.) infesting chilli (Capsicum annum L.) crop. Journal of Entomology and Zoology Studies 8, 1428-1433.
| Google Scholar |
Hashimi MH, Hashimi R, Ryan Q (2020) Toxic effects of pesticides on humans, plants, animals, pollinators and beneficial organisms. Asian Plant Research Journal 5, 37-47.
| Crossref | Google Scholar |
Hatamleh AA, Danish M, Al-Dosary MA, El-Zaidy M, Ali S (2022) Physiological and oxidative stress responses of Solanum lycopersicum (L.) (tomato) when exposed to different chemical pesticides. RSC Advances 12, 7237-7252.
| Crossref | Google Scholar | PubMed |
Hothorn T, Bretz F, Westfall P (2008) Simultaneous inference in general parametric models. Biometrical Journal 50, 346-363.
| Crossref | Google Scholar | PubMed |
Hua D, Chen WS, Rao RY, Chen XF, Chen HH, Lai NW, Yang LT, Ye X, Chen LS (2024) Nitrogen-deficient leaves and roots can keep high abilities to scavenge reactive oxygen species and methylglyoxal, and protect them against oxidative damage in Citrus sinensis seedlings. Scientia Horticulturae 325, 112709.
| Crossref | Google Scholar |
Jalali K, Nouairi I, Kallala N, M’Sehli W, Zribi K, Mhadhbi H (2018) Germination, seedling growth, and antioxidant activity in four legume (Fabaceae) species under copper sulphate fungicide treatment. Pakistan Journal of Botany 50, 1599-1606.
| Google Scholar |
Jalloh AA, Yusuf AA, Khamis F, Subramanian S, Mutyambai DM (2023) Soil legacies in maize-edible legume intercropping alter maize growth and reduce Spodoptera frugiperda larval feeding. Frontiers in Agronomy 5, 1300545.
| Crossref | Google Scholar |
Jalloh AA, Khamis FM, Yusuf AA, Subramanian S, Mutyambai DM (2024) Long-term push–pull cropping system shifts soil and maize-root microbiome diversity paving way to resilient farming system. BMC Microbiology 24, 92.
| Crossref | Google Scholar | PubMed |
Karabal E, Yücel M, Öktem HA (2003) Antioxidant responses of tolerant and sensitive barley cultivars to boron toxicity. Plant Science 164, 925-933.
| Crossref | Google Scholar |
Kassambara A, Mundt F (2020) factoextra: Extract and Visualize the Results of Multivariate Data Analyses. R package version 1.0.7. Available at https://CRAN.R-project.org/package=factoextra
Kay M, Elkin L, Higgins J, Wobbrock J (2021) ARTool: Aligned Rank Transform for Nonparametric Factorial ANOVAs. R package version 0.11.1. Available at https://github.com/mjskay/ARTool
Lamichhane JR (2017) Pesticide use and risk reduction in European farming systems with IPM: an introduction to the special issue. Crop Protection 97, 1-6.
| Crossref | Google Scholar |
Lê S, Josse J, Husson F (2008) FactoMineR: an R package for multivariate analysis. Journal of Statistical Software 25, 1-18.
| Crossref | Google Scholar |
Lenth RV (2023) emmeans: estimated marginal means, aka least-squares means. R package version 1.9.0. Available at https://cran.r-project.org/package=emmeans
Mahboob W, Yang G, Irfan M (2023) Crop nitrogen (N) utilization mechanism and strategies to improve N use efficiency. Acta Physiologiae Plantarum 45, 52.
| Crossref | Google Scholar |
Martinuzzi CS, Attademo AM, Peltzer PM, Mac Loughlin TM, Marino DJG, Lajmanovich RC (2020) Comparative toxicity of two different dimethoate formulations in the Common Toad (Rhinella arenarum) Tadpoles. Bulletin of Environmental Contamination and Toxicology 104, 35-40.
| Crossref | Google Scholar | PubMed |
Meena RS, Kumar S, Datta R, Lal R, Vijayakumar V, Brtnicky M, Sharma MP, Yadav GS, Jhariya MK, Jangir CK, Pathan SI, Dokulilova T, Pecina V, Marfo TD (2020) Impact of agrochemicals on soil microbiota and management: a review. Land 9, 34.
| Crossref | Google Scholar |
Melki F, Talbi Zribi O, Jeder S, Louati F, Nouairi I, Mhadhbi H, Zribi K (2022) Cadmium and lead excess differently affect growth, photosynthetic activity and nutritional status of Trigonella foenum-graecum L. Crop & Pasture Science 73, 969-980.
| Crossref | Google Scholar |
Mishra V, Srivastava G, Prasad SM (2009) Antioxidant response of bitter gourd (Momordica charantia L.) seedlings to interactive effect of dimethoate and UV-B irradiation. Scientia Horticulturae 120, 373-378.
| Crossref | Google Scholar |
Mishra V, Mishra P, Srivastava G, Prasad SM (2011) Effect of dimethoate and UV-B irradiation on the response of antioxidant defense systems in cowpea (Vigna unguiculata L.) seedlings. Pesticide Biochemistry and Physiology 100, 118-123.
| Crossref | Google Scholar |
Oh K, Cao T, Li T, Cheng H (2014) Study on application of phytoremediation technology in management and remediation of contaminated soils. Journal of Clean Energy Technologies 2, 216-220.
| Crossref | Google Scholar |
Oladapo BO, Ekundayo EA, Ekundayo FO, Gbaye OA (2021) Effect of lambda-cyhalothrin and dimethoate on the growth response of cowpea plants and the surrounding soil. Annals of Science and Technology 6, 1-13.
| Crossref | Google Scholar |
Pandey AK, Kanaujia KR (2010) Effect of different insecticides, fungicides and herbicides on growth, sporulation and germination of fungi (Metarhizium anisopliae). The Indian Journal of Agricultural Sciences 80, 265-268.
| Google Scholar |
Parween T, Jan S, Mahmooduzzafar, Fatma T (2011) Alteration in nitrogen metabolism and plant growth during different developmental stages of green gram (Vigna radiata L.) in response to chlorpyrifos. Acta Physiologiae Plantarum 33, 2321-2328.
| Crossref | Google Scholar |
Pawar AP, Sanaye SV, Shyama S, Sreepada RA, Dake AS (2020) Effects of salinity and temperature on the acute toxicity of the pesticides, dimethoate and chlorpyrifos in post-larvae and juveniles of the whiteleg shrimp. Aquaculture Reports 16, 100240.
| Crossref | Google Scholar |
Pearson K (1895) Notes on regression and inheritance in the case of two parents. Proceedings of the Royal Society of London 58, 240-242.
| Google Scholar |
Quaggiotti S, Buzzicotti L, Koch KE, Guan JC, Trevisan S, Varotto S, Ruperti B, Ravazzolo L (2024) Strigolactone roles in maize tolerance to low nitrogen involve shifts in acquisition and partitioning of protein, sulfur, and iron. Plant and Soil 505, 41-64.
| Crossref | Google Scholar |
Ramírez-Parra E, De la Rosa L (2023) Designing novel strategies for improving old legumes: an overview from common vetch. Plants 12, 1275.
| Crossref | Google Scholar | PubMed |
Sadeghzadeh-ahari D, Hassandokht MR, Kashi AK, Amri A, Alizadeh KH (2010) Genetic variability of some agronomic traits in the Iranian Fenugreek landraces under drought stress and non-stress conditions. Plant Science 4, 12-20.
| Google Scholar |
Shakir SK, Kanwal M, Murad W, Zia ur Rehman, Shafiq ur Rehman, Daud MK, Azizullah A (2016) Effect of some commonly used pesticides on seed germination, biomass production and photosynthetic pigments in tomato (Lycopersicon esculentum). Ecotoxicology 25, 329-341.
| Crossref | Google Scholar | PubMed |
Shakir SK, Irfan S, Akhtar B, Shafiq ur Rehman, Daud MK, Taimur N, Azizullah A (2018) Pesticide-induced oxidative stress and antioxidant responses in tomato (Solanum lycopersicum) seedlings. Ecotoxicology 27, 919-935.
| Crossref | Google Scholar | PubMed |
Shapiro SS, Wilk MB (1965) An analysis of variance test for normality (complete samples). Biometrika 52, 591-611.
| Crossref | Google Scholar |
Sharma A, Kumar V, Thukral AK, Bhardwaj R (2019) Responses of plants to pesticide toxicity: an overview. Planta Daninha 37, e019184291.
| Crossref | Google Scholar |
Shingote PR, Kawar PG, Pagariya MC, Muley AB, Babu KH (2020) Isolation and functional validation of stress tolerant EaMYB18 gene and its comparative physio-biochemical analysis with transgenic tobacco plants overexpressing SoMYB18 and SsMYB18. 3 Biotech 10, 225.
| Crossref | Google Scholar | PubMed |
Singh VP, Kumar J, Singh S, Prasad SM (2014) Dimethoate modifies enhanced UV-B effects on growth, photosynthesis and oxidative stress in mung bean (Vigna radiata L.) seedlings: implication of salicylic acid. Pesticide Biochemistry and Physiology 116, 13-23.
| Crossref | Google Scholar | PubMed |
Singh P, Singh M, Singh SK, Prasad SM (2023) Application of soil amendments mitigates phytotoxic effects on Solanum melongena L. and Lycopersicon esculentum L. seedlings exposed to chlorpyrifos and dimethoate pesticides. Environmental Science and Pollution Research 30, 59891-59908.
| Crossref | Google Scholar | PubMed |
Song NH, Yin XL, Chen GF, Yang H (2007) Biological responses of wheat (Triticum aestivum) plants to the herbicide chlorotoluron in soils. Chemosphere 68, 1779-1787.
| Crossref | Google Scholar | PubMed |
Spearman C (1904) The proof and measurement of association between two things. The American Journal of Psychology 15, 72-101.
| Crossref | Google Scholar |
Srivastava PK, Singh VP, Prasad SM (2014) Low and high doses of UV-B differentially modulate chlorpyrifos-induced alterations in nitrogen metabolism of cyanobacteria. Ecotoxicology and Environmental Safety 107, 291-299.
| Crossref | Google Scholar | PubMed |
Stagnari F, Maggio A, Galieni A, Pisante M (2017) Multiple benefits of legumes for agriculture sustainability: an overview. Chemical and Biological Technologies in Agriculture 4, 2.
| Crossref | Google Scholar |
Stefan L, Hartmann M, Engbersen N, Six J, Schöb C (2021) Positive effects of crop diversity on productivity driven by changes in soil microbial composition. Frontiers in Microbiology 12, 660749.
| Crossref | Google Scholar | PubMed |
Strassemeyer J, Daehmlow D, Dominic AR, Lorenz S, Golla B (2017) SYNOPS-WEB, an online tool for environmental risk assessment to evaluate pesticide strategies on field level. Crop Protection 97, 28-44.
| Crossref | Google Scholar |
Syafrudin M, Kristanti RA, Yuniarto A, Hadibarata T, Rhee J, Al-Onazi WA, Algarni TS, Almarri AH, Al-Mohaimeed AM (2021) Pesticides in drinking water-a review. International Journal of Environmental Research and Public Health 18, 468.
| Crossref | Google Scholar | PubMed |
Toukabri W, Ferchichi N, Hlel D, Jadlaoui M, Kheriji O, Mhamdi R, Trabelsi D (2021) Response of intercropped barley and fenugreek to mono- and co-inoculation with Sinorhizobium meliloti F42 and Variovorax paradoxus F310 under contrasting agroclimatic regions. Archives of Microbiology 203, 1657-1670.
| Crossref | Google Scholar | PubMed |
Tsatsakis AM, Tsakiris IN (2010) Fenthion, dimethoate and other pesticides in olive oils of organic and conventional cultivation. In ‘Olives and olive oil in health and disease prevention’. (Eds VR Preedy, RR Watson) pp. 415–424. (Academic Press) 10.1016/B978-0-12-374420-3.00046-2
Wei T, Simko V (2021) corrplot: visualization of a correlation matrix. R package version 0.92. Available at https://github.com/taiyun/corrplot
Xiao J, Yin X, Ren J, Zhang M, Tang L, Zheng Y (2018) Complementation drives higher growth rate and yield of wheat and saves nitrogen fertilizer in wheat and faba bean intercropping. Field Crops Research 221, 119-129.
| Crossref | Google Scholar |
Yan E, Munier-Jolain N, Martin P, Carozzi M (2024) Intercropping on french farms: reducing pesticide and N fertiliser use while maintaining gross margins. European Journal of Agronomy 152, 127036.
| Crossref | Google Scholar |
Yang J, Lan L, Jin Y, Yu N, Wang D, Wang E (2022) Mechanisms underlying legume–rhizobium symbioses. Journal of Integrative Plant Biology 64, 244-267.
| Crossref | Google Scholar | PubMed |
Yemm EW, Willis AJ (1954) The estimation of carbohydrates in plant extracts by anthrone. The Biochemical Journal 57, 508-514.
| Crossref | Google Scholar |
Yildiztekin M, Kaya C, Tuna AL, Ashraf M (2015) Oxidative stress and antioxidative mechanisms in tomato (Solanum lycopersicum L.) plants sprayed with different pesticides. Pakistan Journal of Botany 47, 717-721.
| Google Scholar |
Zeileis A, Fisher JC, Hornik K, Ihaka R, McWhite CD, Murrell P, Stauffer R, Wilke CO (2020) Colorspace: a toolbox for manipulating and assessing colors and palettes. Journal of Statistical Software 96, 1-49.
| Crossref | Google Scholar |