Call broadcast surveys monitor owls with more precision than passive surveys by citizen scientists or acoustic recording units
V. F. Sperring

A
B
C
D
E
Abstract
Population monitoring that effectively detects population changes is an important component of threatened species management. Call broadcast surveys are regularly used for robust monitoring of vocal or territorial species; however, they can be resource intensive. Passive monitoring approaches involving citizen scientists and acoustic recording units may be less costly, and consequently these methods are increasingly being advocated.
This study investigated the utility of three different monitoring approaches to detect population trends in the Critically Endangered Norfolk Island morepork Ninox novaeseelandiae undulata. It compared the effectiveness of call broadcast with that of passive citizen scientist and acoustic recording surveys.
Between November and December 2020, we trialled each technique on Norfolk Island. The coefficient of variation in the number of moreporks heard per survey, and the mean detectability at each site were calculated as the core metrics for each technique. The statistical power of each technique to detect population trends over time was also calculated to compare efficacy. An extensive island-wide call broadcast survey, roost searching, and GPS tracking program were used to establish an independent population estimate.
We established a population estimate of 25 individuals. The call broadcast approach had the highest precision and highest site detectability. This approach was also assessed as being capable of detecting a change of just two or three moreporks per year, after three years of monitoring. By contrast, approaches using passive citizen science surveys and acoustic recording units would require at least ten years of monitoring to detect similar population trends.
Call broadcast surveys had a greater capacity to achieve high detectability and precise monitoring, relative to the passive approaches.
The high precision of call broadcast appears to justify the higher resource requirements relative to other methods examined in this study, where the target species is territorial and extremely rare. Call broadcast surveys are recommended for long-term monitoring of Norfolk Island moreporks.
Keywords: call broadcast, citizen scientists, passive acoustic recording units, population monitoring, threatened species.
Introduction
Reliable population monitoring programs are an essential part of conservation management. Detection of fluctuations or stability in population size allows evaluation of the efficacy of management and informs the need for further intervention. Despite the importance of population monitoring, particularly for threatened species, monitoring is regularly overlooked or poorly executed in management programs (Nisbet 2007; Blanco et al. 2012; Lindenmayer et al. 2018). This is often due to financial and time constraints, or logistical challenges (Hauser et al. 2006; Lindenmayer et al. 2012; Legge et al. 2018). Monitoring small populations can be particularly challenging due to small available sample sizes and detectability limitations (Au et al. 1998; Clarke et al. 2003; Elliot et al. 2020). Designing monitoring programs for such species often involves complex decisions and trade-offs relating to methods and intensity (e.g. Legge et al. 2018; Southwell et al. 2024).
For effective population monitoring, it is important to establish clear goals (Lindenmayer and Likens 2018). Precise and accurate monitoring are frequently used interchangeably as monitoring goals; however, each has a distinct meaning (Sorrell et al. 2019). A precise estimate seeks to consistently replicate population indices with low variance as a means of detecting population trends (Gibbs et al. 1998). By contrast, an accurate estimate represents a value close to the true population size, while noting an exact size is often unattainable for wild populations (Gregory et al. 2004). Consequently, it can be relatively straightforward to estimate the variance between replicated counts as a means to assess precision, and difficult to directly assess the accuracy of any count method (Yoccoz et al. 2001). Furthermore, obtaining a precise population index is often less resource intensive and more critical for informing conservation decisions (Gregory et al. 2004). Here, we focus primarily on comparing the precision of estimates obtained from different monitoring methods.
A traditional monitoring approach for vocal species is call broadcast surveys (Marion et al. 1981; Hambler et al. 1993). Call broadcast involves playing audio recordings to attract and elicit a vocal response from a target species (Chrenková et al. 2017; Vrezec and Bertoncelj 2018). It is used to accurately detect population trends in many territorial species that respond to conspecific intruders, including species that are cryptic and present in low numbers (Lor and Malecki 2002; Conway and Gibbs 2005; Chrenková et al. 2017). However, there is evidence to suggest that call broadcast can distract subjects from essential breeding behaviours and may negatively affect pair bond status in some species (Appleby et al. 1999; Fair et al. 2010; Harris and Haskell 2013). For ethical reasons, this approach often requires experienced professionals to conduct the surveys, leading to high resource demands. Consequently, while call broadcast surveys can provide robust estimates of population trends, the approach may not be suitable for programs with significant time and budget constraints.
An alternative is to conduct surveys passively, in the sense of simply recording the natural vocalisations (or other signs of presence) of a species, without use of playback to elicit a response. Such approaches may require less specialist equipment and skills, and may lend themselves to citizen science programs where volunteers (citizen scientists) are trained to collect data on species presence (Adler et al. 2020). Citizen science approaches are often cost-effective, allow collection of large quantities of data, and promote community engagement (Conrad and Hilchey 2011; Bonney et al. 2016). Citizen scientists have been used to investigate species richness as well as the occurrence of common and threatened species across large and remote areas (Chandler et al. 2017; Farhadinia et al. 2018; Benshemesh et al. 2020). Non-expert contributions, however, are generally expected to reduce monitoring precision and the power to detect population trends (Westgate et al. 2015; Kamp et al. 2016; though see Viola et al. 2022). Whether structured citizen science programs can precisely monitor small populations warrants further investigation.
Acoustic recording units (ARU) are another tool that can be used for passive monitoring. The use of ARUs has increased substantially in recent years due to the improved efficiency in collecting large volumes of data over large spatial and temporal scales (Ribeiro et al. 2017; Zwerts et al. 2021). ARUs can be stationed within a landscape and programmed to record audio on a predetermined schedule. ARU-derived datasets can then be analysed with automated call recognition software or volunteers to determine the presence or absence of target species (Wolfgang and Haines 2016; Marsland et al. 2019). With advances in machine learning techniques, and increasingly affordable and accessible technology, this technique provides another relatively low-cost and time-efficient monitoring tool (Hill et al. 2018). ARUs are well-suited for assessing the distribution of vocal species and have been used to detect trends in some species including bats, marine mammals, and owls (Jaramillo-Legorreta et al. 2017; Wood et al. 2020; Zwerts et al. 2021; Kotila et al. 2023). The ability of this approach to detect population trends in threatened bird populations has been trialled but species-specific research is required (Gibb et al. 2019; Pérez-Granados and Schuchmann 2021).
The Norfolk Island morepork (Ninox novaeseelandiae undulata) is listed as Critically Endangered under the Australian EPBC Act owing to its very small and declining population (Sperring et al. 2021). It is one of the threatened species addressed in the Norfolk Island Region Threatened Species Recovery Plan (Commonwealth of Australia 2024) and one of the focuses of conservation management on the island. The species is territorial with a distinct call profile relative to the broader forest bird community on Norfolk Island. With a population estimate between just 25 and 50 individuals (Sperring et al. 2021), a monitoring program with high power to detect small changes in population trends is essential. Here, we compare the capacity for call broadcast as well as passive citizen scientist and ARU surveys to detect trends in this small population. Further, we seek to estimate the population size, and determine the most appropriate monitoring method for the Norfolk Island morepork, to inform the ongoing conservation program for this small Ninox hawk-owl.
Materials and methods
Site description
Norfolk Island is a small oceanic island (3460 ha) in the south-west Pacific Ocean. Norfolk Island National Park (~450 ha) comprises contiguous vegetation, most of which is native forest. Additional areas of wooded cover in the park comprise thickets of invasive woody weeds, most notably cherry guava (Psidium cattleyanum) and African olive (Olea europaea cuspidata). The remaining land area is largely utilised for urban settlement, agriculture, an airport, and small forested reserves, and is hereafter referred to as human-modified landscape (Director of National Parks 2010). The island supports the global population of the Norfolk Island morepork, with this small Ninox hawk-owl displaying an island-wide distribution (Sperring et al. 2024a). Nevertheless, records and indeed morepork territories are concentrated within the National Park (Sperring et al. 2024b). Three monitoring methods were trialled across Norfolk Island between November and December 2020 (Fig. 1). Monitoring occurred over 6-weeks during the peak vocal activity period to minimise changes in the probability of detection due to interannual variability (Zuberogoitia et al. 2011).
Acoustic recording units (ARU) (square, n = 13), citizen science (circle, n = 20), and call broadcast (cross, n = 25) survey sites for Norfolk Island moreporks across Norfolk Island, western Pacific Ocean. Darker shading on this map represents woody vegetation, lighter shading represents cleared areas and white areas indicate the presence of infrastructure.
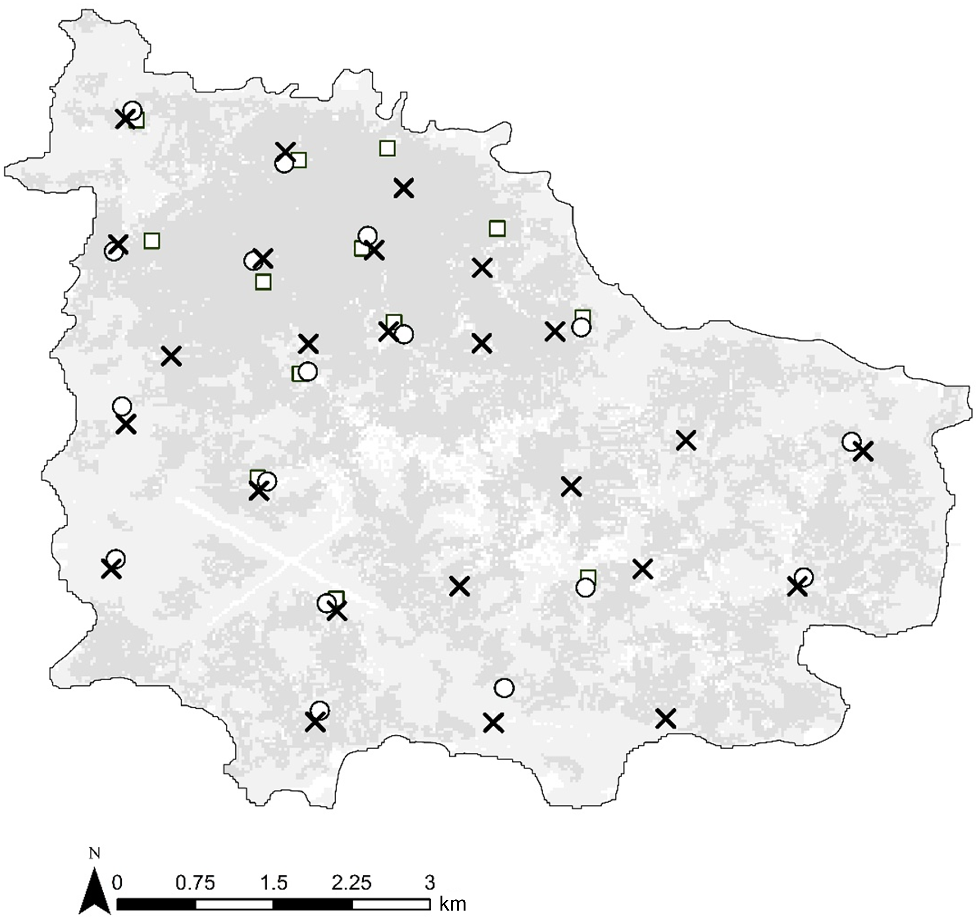
Call broadcast
Twenty-five listening sites were established across Norfolk Island. Sites within the National Park were at least 1 km apart and sites outside the National Park at least 1.5 km apart. These a priori distances were based on preliminary passive and call broadcast surveys that indicated moreporks can be heard from ~1.4 km away under calm conditions within the more open human-modified landscape, but typically no further than 1 km away within the National Park due to its continuous wooded vegetation (F. Sperring, unpubl. data). Specific sites were selected using a random stratified approach. First, random sites at least 1 km from all other sites were generated using GIS software. These sites were then adjusted first to the nearest accessible site to allow fieldworkers routine access via vehicle or on foot. Second, selected sites were confirmed to support an open understorey or canopy as dense closed thickets, predominately of the invasive weed cherry guava, are suspected to be unsuitable for the morepork (Sperring et al. 2021). All final selected sites were within 500 m of their original randomly generated location.
All 25 sites were monitored on the same night within a 2-h window starting 30 min after sunset following the recommendations of Pryde et al. (2020). All surveyors had prior biodiversity monitoring experience and were categorised as experts. Each site was monitored by one of five experts for 10 min. Sites were surveyed once per week for a total of five survey nights, though the survey period ultimately spanned a period of 6 weeks as severe weather delayed one planned round of surveys. For closely related Ninox hawk-owls, three nights has been considered the minimum required to achieve 95% confidence of site-specific absence with similar survey methods (Cooke et al. 2017). As part of this trial, we sought to exceed that previously established minimum requirement, while the maximum number of survey nights was constrained by the resourcing capacity of land managers.
Once established at each site, surveyors listened for a 2-min period before playing 2 min of morepork calls broadcast on a UE Wonderboom Portable Bluetooth speaker. A further 2 min of listening was conducted before a final 2-min bout of call broadcast. The survey concluded with a further 2 min of listening and spotlighting to generate a total survey period of 10 min per site (see also Cooke et al. 2017). Spotlighting was used during the last 2 min to ensure any moreporks that had approached during the survey period without calling might be detected. At each site, calls were played at a volume where another researcher could hear them from ~500 m away, following equipment-specific trials with two fieldworkers. Survey protocol continued regardless of whether a morepork was heard calling. Individual moreporks were distinguished based on the direction and distance of their call, and the total number of moreporks that called in the 10-min period was recorded.
Passive surveys by citizen scientists
An additional 20 passive monitoring sites were established across the island, each at least 1.5 km from any other site. To determine site locations, community members were first asked to express interest in monitoring from their residence, and three private residences were chosen. The remaining sites were located at call broadcast monitoring sites, or at a more accessible location for the general community. Six sites were located within the National Park. All sites were random, relative to known morepork territories or roosting sites. Over an 8-week period, all sites were monitored once per week for a total of seven nights of monitoring. As with call broadcast, surveys did not proceed in one week due to severe weather. All sites were monitored simultaneously by volunteers from the community, commencing 30 min after sunset for a duration of 1 h. Volunteers were provided with one training session to aid accurate data collection. The number of moreporks calling in each of four 15-min periods was recorded at each site to minimise the chance of double-counting moreporks that had moved to a new location. Fifty volunteers participated in the citizen science monitoring approach and only one site on one night was missed over the duration of the trial (3% of total surveys). Citizen science surveys were not conducted on the same day as call broadcast surveys.
Passive acoustic recording units
Sixteen AudioMoth ARUs were established across Norfolk Island from 2 November 2020 to 21 December 2020. Thirteen recorders successfully recorded data with these being used in the subsequent analysis. Detection distance was expected to be up to 250 m from recording devices (Pryde and Greene 2016). Sites were selected using a random stratified approach. First, random sites at least 2 km from all other sites were generated. These sites were adjusted first to the nearest location away from potential noise pollution, second, to sites that were positioned on the upper half of a slope (i.e. not in a gully or valley floor) and third, to sites with an open understorey or canopy (Duchac et al. 2020). All final selected sites were within 500 m of their original randomly generated location. Where the specified selection criteria were met, sites established for citizen science or call broadcast monitoring were also selected for ARUs. Devices were set to record each night for 1 h from 6 to 7 pm (approximately 1 h before sunset when preliminary surveys established calling to start), 9–10 pm and 12–1 am local time. Recordings were imported into AviaNZ automated call recognition software, developed for the detection of New Zealand moreporks (N. n. novaeseelandiae) which have a very similar call (Marsland et al. 2019). Each 1-h recording was partitioned into four 15-min surveys to reduce the probability that moreporks that may have moved between sites were counted twice. Within recordings, all positive detections that were made using the automated call recognition software were also verified manually.
Population size estimate
A whole-island population estimate was derived by combining GPS tracking between October 2019 and May 2021 (see Sperring et al. 2024a), extensive island-wide call broadcast surveys, and roost searching. Given the intensive nature of the fieldwork and that many individuals were banded and GPS tracked, this provided a reliable estimate of all known territories across the island. As non-territorial, non-calling moreporks may not be detected, an additional 10% buffer was added to the estimate of territory-holding individuals to provide both a conservative and defensible total population estimate (Rohner 1997; Severinghaus 2000).
Data analyses
The mean and the coefficient of variation of the number of moreporks heard per survey was calculated for each method. The term ‘survey’ refers to the sum of all sites monitored within the same time-period (i.e. one survey per night for citizen science and expert monitoring, three surveys per night for passive acoustic monitoring). Detectability of moreporks at each site was calculated for each method as the proportion of surveys per site that detected a morepork. Sites with no detections throughout the monitoring period were excluded from the detectability calculation as it was assumed that there were no moreporks present at these sites. Kruskal–Wallis and Pairwise Wilcox tests were implemented using the package ‘dplyr’ to compare the number of moreporks heard per survey and site detectability between methods (Wickham et al. 2023). A chi-square test was used to compare the proportion of sites with and without detections for each method. Necessary statistical assumptions for each test were checked and met. Unless otherwise stated, all analyses were implemented in R and significance was tested at the 0.05 level (R Core Team 2023).
To determine the statistical power of each monitoring technique to detect population trends in the Norfolk Island morepork population, we used MONITOR (v11.0.2) (Gibbs et al. 1998; Gibbs 2001). MONITOR generates many simulated sets of count data based on a monitoring program defined by the user. Through multiple trials, MONITOR evaluates how often the program detects trends of varying strength. The proportion of trials in which the trend significantly differed from zero is used to estimate power. For each monitoring method, a simple regression design type with log-normal measure and total variance was used. We also used a deterministic/regression trend type and a constant coefficient of variation to mean relationship. Simulations were run with 10,000 iterations and two tails with an alpha level of 0.05. Counts less than zero were truncated and count values were rounded. We calculated the percentage trend in population size (positive and negative) detectable at the 0.9 power level for 3, 5 and 10 years of monitoring; varying the number of survey nights per year as a measure of intensity (call broadcast: 4, 5, and 6 nights; citizen science: 4, 7, and 12 nights; ARU: 26, 56, and 84 nights). We combined the percentage trend in population size results with the lowest population size estimate generated from the more intensive multi-year fieldwork to calculate the minimum detectable annual change in the number of moreporks.
Results
Twenty-two unique Norfolk Island moreporks were identified across Norfolk Island. The total population size was estimated at 25 moreporks. The population was mostly concentrated within the National Park but at least six moreporks including two pairs were found occupying territory entirely within the human-modified landscape.
Detectability
Survey method had a significant effect on the number of moreporks heard per survey (Kruskal–Wallis chi-squared = 104.4, P < 0.01). Call broadcast detected significantly more individuals than both passive methods (citizen science: P < 0.01 and ARU: P < 0.01) and had the smallest coefficient of variation (Fig. 2). Detectability at each site was also significantly influenced by survey method (Kruskal–Wallis chi-squared = 16.3, P < 0.01), with higher detectability at call broadcast sites relative to ARU and citizen science sites (P < 0.01 and P < 0.05). Citizen science sites also had higher detectability relative to ARU (P = 0.03) (Fig. 3). The proportion of sites with detections did not differ significantly among survey methods (call broadcast = 0.68, citizen science = 0.75, ARU = 0.69) (χ2(2, 45) = 0.28, P = 0.87).
Boxplot showing the number of Norfolk Island moreporks detected by call per survey for each monitoring method. The median and interquartile range are shown for each method, whiskers indicate the spread of data, and outliers are marked as individual points. X denotes the mean number of moreporks heard per survey. Values above each boxplot represent the coefficient of variation expressed as a percentage.
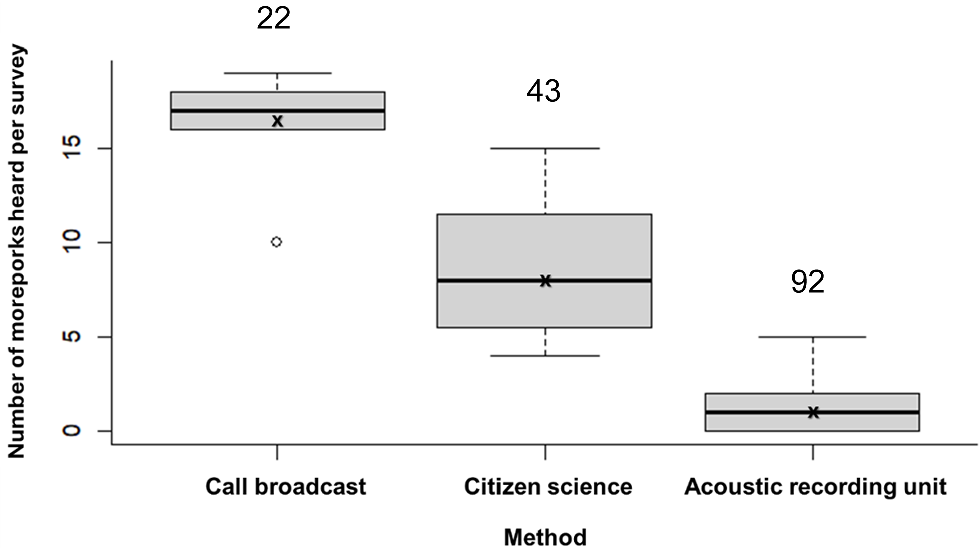
Boxplot showing site detectability of Norfolk Island moreporks for each monitoring method. The median and interquartile range are shown for each method, whiskers indicate the spread of data, and outliers are marked as individual points. X denotes mean detectability. Different letters above boxplots indicate significant differences between all methods. The number of surveys for each method = 5 (call broadcast), 28 (citizen science), and 312 (acoustic recording units).
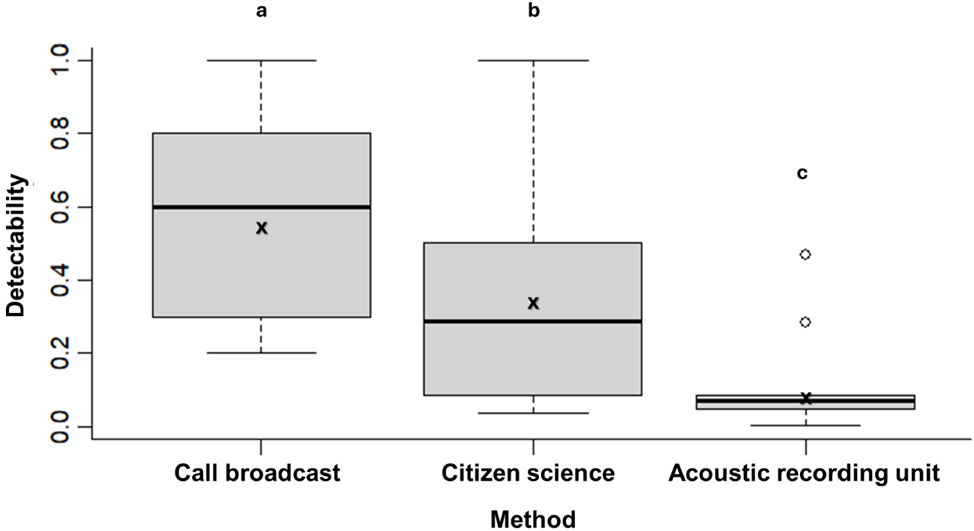
Power analysis
In the power analysis simulations, call broadcast surveys could detect a change of just two moreporks (8% change in population) after 3 years of monitoring, demonstrating high statistical power to detect small changes in population. By contrast, both passive approaches were found to have low statistical power. After 3 years of monitoring, citizen science and ARU-based strategies could at best detect an annual change of 9 and 10 moreporks (27% and 30% change in population), respectively (Table 1). It would require 10 years of monitoring before these strategies could detect an annual population change of two moreporks (Table 2).
Survey nights | Number of moreporks | |||
---|---|---|---|---|
Call broadcast | Citizen science | ARU | ||
Low | 3 | 19 | 17 | |
Moderate | 3 | 12 | 11 | |
High | 2 | 10 | 9 |
Years | Call broadcast (%) | Citizen Science (%) | ARU (%) | |||||||
---|---|---|---|---|---|---|---|---|---|---|
Low | Moderate | High | Low | Moderate | High | Low | Moderate | High | ||
Three | 11 | 9 | 8 | 55 | 36 | 30 | 50 | 33 | 27 | |
Five | 5 | 4 | 4 | 22 | 16 | 14 | 22 | 12 | 12 | |
Ten | 2 | 2 | 2 | 8 | 6 | 5 | 8 | 5 | 5 |
Discussion
At 25 individuals, the Norfolk Island morepork population can be characterised as tiny, highlighting the importance of a survey method that has high power to detect changes of just a few individuals. The call broadcast approach that was trialled in this study was more effective than the passive approaches and had a high probability of detecting population changes. The passive citizen science and ARU strategies exhibited lower precision, site detectability and power to detect population trends than the call broadcast approach. The proportion of sites with morepork detections was similar across all techniques. Neither of the two passive approaches tested in this study were sufficiently precise for long-term monitoring of a small population of moreporks.
Call broadcast surveys detected approximately half of the total population estimate with high precision. Like other owl species, small Ninox hawk-owls are highly vocal and territorial, utilising calls for mate attraction, courtship, and territory defence (Olsen and Hayes 2002; Brighten 2015). Individuals will often approach and respond vocally to unfamiliar calls near their territory, including call broadcast (Olsen and Hayes 2002; Brighten 2015; Cooke et al. 2017). Call broadcast surveys therefore increase detectability relative to passive listening, consequently increasing the power to detect population trends when survey effort remains the same (Allen et al. 2004; Vrezec and Bertoncelj 2018; Zuberogoitia et al. 2020). While call broadcast may influence breeding behaviours, there is little evidence to date to suggest this results in reduced breeding success (Fair et al. 2010; Harris and Haskell 2013; Watson et al. 2019). Therefore, the benefit of precise monitoring to inform management interventions is often deemed to outweigh the predicted cost of call broadcast (e.g. Conway and Gibbs 2005; Vrezec and Bertoncelj 2018). When managing threatened species with very small populations, minimising disturbance to breeding is of high importance. However, understanding the success of current management practices, and being able to detect small changes in numbers of individuals, or territory occupancy, is also critical. In circumstances where call broadcast is a necessary strategy, experts or a small group of well-trained community volunteers should conduct surveys to meet acceptable requirements and minimise the potential impact (Viola et al. 2022).
While the citizen science-based monitoring technique was less precise than expert-led call broadcast surveys, we expect this was due to the passive nature of the approach rather than the experience of the listeners. Factors such as observer age and experience are important considerations (Eglington et al. 2010; Farmer et al. 2014); however, studies directly comparing expert and citizen science contributions do not consistently show evidence to suggest lower precision in volunteer-derived data (Lewandowski and Specht 2015). Expert researchers usually detect more calls than amateur volunteers (Zuberogoitia et al. 2020); however, providing sufficient training to volunteers can partly or fully resolve this disparity (Jiguet 2009; Lewandowski and Specht 2015; Kosmala et al. 2016). Citizen scientists often have specialised interests in particular taxa, becoming accustomed to subtle nuances in appearance, behaviour, or dynamics (Viola et al. 2022). Many citizen scientists who contribute bird-watching data to public repositories are also already familiar with the use of call broadcast (Watson et al. 2019). With careful training around ethics and research protocols, a small cohort of trained community observers may contribute as ‘experts’ to a well-structured call broadcast program.
A common criticism of structured citizen science programs is volunteer retention, which can be characterised as low, with consequent high turnover (Einolf 2018). In this context, training can be time consuming, increasing the resource demand of this approach (Beirne and Lambin 2013). Throughout the 8 weeks of this trial, volunteer retention was high, likely because of an environmentally active community with a strong interest in the project and species (see Beirne and Lambin 2013). The simplicity of the approach may have also contributed to successful volunteer retention (see Kosmala et al. 2016). Concurrently, restrictions on travel to and from the island because of the COVID-19 pandemic, as well as fewer activities available to the community, may have increased participants’ commitment. While the long-term commitment of these volunteers cannot be known, the dedication over 8 weeks supports the contention that a small cohort of citizen scientists may serve as a reliable resource for a long-term monitoring program.
The ARU approach had the highest coefficient of variation, detecting a mean of one morepork per survey, and ultimately displayed low power to detect population trends. While fewer sites were monitored using this approach relative to the broadcast and citizen science approaches, major limitations were the inability to detect more than one morepork per site and the limited distance at which calls could be detected. Each of these are common shortcomings experienced in other ARU based programs (Hill et al. 2018; Gibb et al. 2019; Sugai et al. 2019). The causes of failure for three devices used within this study are unknown. Failure may have been the result of water ingress, however, the very low build-cost of these units means that hardware failure in the absence of an external driver also occurs occasionally (Hill et al. 2018; Prince et al. 2019). Deploying more units across the landscape would likely increase the number of moreporks heard per survey which may improve the power of this approach to detect trends. Analyses that account for imperfect detection could also be used to inform sampling effort required to meet monitoring goals.
To enhance the ARU approach, improvements to microphone quality could also increase detections per site and allow differentiation between individuals based on distance or direction from the ARU (Marques et al. 2013; Pryde and Greene 2016; Shonfield and Bayne 2017). ARU-based programs could also consider incorporating automated call broadcast to improve the detectability of cryptic species or populations occurring in low densities. Such modifications have the potential to increase the power of an ARU-based program to detect trends. Other technological advances such as machine learning approaches to recognise calls of individuals within the population may also improve an ARU-based program in the future. For small, isolated populations, this approach has the potential to provide an accurate and precise measure of population size by estimating all calling individuals (Molnár et al. 2008; Mielke and Zuberbühler 2013; Clink and Klinck 2021). Nevertheless, in the short term at least, we consider that the ARU program tested here is not suitable to precisely detect changes in the Norfolk Island morepork population.
Conclusion
Determining the most effective survey method is an important element of designing appropriate monitoring programs, especially when the subject is a threatened species with a very small population and high precision is required. Monitoring is a significant investment in any conservation program, and resource limitations can often lead to monitoring being carried out insufficiently frequently or with suboptimal methods (Lindenmayer et al. 2012, 2018; Clink and Klinck 2021). It is therefore essential for programs to have a clear understanding of the power of different methods (in combination with other factors such as site number and survey frequency) to detect change, and to balance what is achievable for implementation against the target level of monitoring precision. Despite the growing availability of technology for wildlife monitoring purposes, our results highlight that, in some cases, traditional monitoring approaches may still best meet program requirements and achieve the most reliable outcomes for population monitoring. To inform conservation of the Norfolk Island morepork, we consider that the high precision of call broadcast outweighs the advantages of reduced resource requirements from passive strategies. This conclusion may be valid for other territorial and vocal species with extremely small populations.
Declaration of funding
This research was funded by the Department of Climate Change, Energy, the Environment and Water through the National Environmental Science Program and Director of National Parks. Victoria Florence Sperring was supported by an Australian Government Research Training Program Scholarship.
Acknowledgements
We acknowledge the contribution of Parks Australia, and Norfolk Island Regional Council. Thanks to Lilli King, Paula Campos, and Will Webster for their assistance conducting the call broadcast surveys. We appreciate the dedication and commitment of all citizen science volunteers. Thanks also to Darren Southwell, Finella Dawlings and Erik Doerr for their feedback on the manuscript. All research was approved by Parks Australia and conducted under Monash University animal ethics approval 2021-25691 and human ethics approval 2020-24574. We recognise and value the customs and traditions of Norfolk Islanders, particularly the descendants of the first Pitcairn settlers, and their ongoing cultural connections to the island.
References
Adler FR, Green AM, Şekercioğlu ÇH (2020) Citizen science in ecology: a place for humans in nature. Annals of the New York Academy of Sciences 1469(1), 52-64.
| Crossref | Google Scholar | PubMed |
Allen T, Finkbeiner SL, Johnson DH (2004) Comparison of detection rates of breeding marsh birds in passive and playback surveys at Lacreek National Wildlife Refuge, South Dakota. Waterbirds 27(3), 277-281.
| Crossref | Google Scholar |
Appleby BM, Yamaguchi N, Johnson PJ, Macdonald DW (1999) Sex-specific territorial responses in Tawny Owls Strix aluco. Ibis 141(1), 91-99.
| Crossref | Google Scholar |
Au WW, Cajas-Salazar N, Salama S (1998) Factors contributing to discrepancies in population monitoring studies. Mutation Research/Fundamental and Molecular Mechanisms of Mutagenesis 400(1-2), 467-478.
| Crossref | Google Scholar | PubMed |
Beirne C, Lambin X (2013) Understanding the determinants of volunteer retention through capture–recapture analysis: answering social science questions using a wildlife ecology toolkit. Conservation Letters 6(6), 391-401.
| Crossref | Google Scholar |
Benshemesh J, Southwell D, Barker R, McCarthy M (2020) Citizen scientists reveal nationwide trends and drivers in the breeding activity of a threatened bird, the malleefowl (Leipoa ocellata). Biological Conservation 246, 108573.
| Crossref | Google Scholar |
Blanco G, Sergio F, Sanchéz-Zapata JA, Pérez-García JM, Botella F, Martínez F, Zuberogoitia I, Frías O, Roviralta F, Martínez JE, Hiraldo F (2012) Safety in numbers? Supplanting data quality with fanciful models in wildlife monitoring and conservation. Biodiversity and Conservation 21, 3269-3276.
| Crossref | Google Scholar |
Bonney R, Phillips TB, Ballard HL, Enck JW (2016) Can citizen science enhance public understanding of science? Public Understanding of Science 25(1), 2-16.
| Crossref | Google Scholar | PubMed |
Chandler M, See L, Copas K, Bonde AMZ, López BC, Danielsen F, Legind JK, Masinde S, Miller-Rushing AJ, Newman G, Rosemartin A, Turak E (2017) Contribution of citizen science towards international biodiversity monitoring. Biological Conservation 213, 280-294.
| Crossref | Google Scholar |
Chrenková M, Dobrý M, Šálek M (2017) Further evidence of large-scale population decline and range contraction of the little owl Athene noctua in Central Europe. Folia Zoologica 66(2), 106-116.
| Crossref | Google Scholar |
Clarke RH, Oliver DL, Boulton RL, Cassey P, Clarke MF (2003) Assessing programs for monitoring threatened species – a tale of three honeyeaters (Meliphagidae). Wildlife Research 30, 427-435.
| Crossref | Google Scholar |
Clink DJ, Klinck H (2021) Unsupervised acoustic classification of individual gibbon females and the implications for passive acoustic monitoring. Methods in Ecology and Evolution 12(2), 328-341.
| Crossref | Google Scholar |
Conrad CC, Hilchey KG (2011) A review of citizen science and community-based environmental monitoring: issues and opportunities. Environmental Monitoring and Assessment 176(1-4), 273-291.
| Crossref | Google Scholar | PubMed |
Conway CJ, Gibbs JP (2005) Effectiveness of call-broadcast surveys for monitoring marsh birds. The Auk 122(1), 26-35.
| Crossref | Google Scholar |
Cooke R, Grant H, Ebsworth I, Rendall AR, Isaac B, White JG (2017) Can owls be used to monitor the impacts of urbanisation? A cautionary tale of variable detection. Wildlife Research 44(7), 573-581.
| Crossref | Google Scholar |
Duchac LS, Lesmeister DB, Dugger KM, Ruff ZJ, Davis RJ (2020) Passive acoustic monitoring effectively detects Northern Spotted Owls and Barred Owls over a range of forest conditions. The Condor 122(3), duaa017.
| Crossref | Google Scholar |
Eglington SM, Davis SE, Joys AC, Chamberlain DE, Noble DG (2010) The effect of observer experience on English Breeding Bird Survey population trends. Bird Study 57(2), 129-141.
| Crossref | Google Scholar |
Einolf C (2018) Evidence-based volunteer management: a review of the literature. Voluntary Sector Review 9(2), 153-176.
| Crossref | Google Scholar |
Elliot NB, Bett A, Chege M, Sankan K, de Souza N, Kariuki L, Broekhuis F, Omondi P, Ngene S, Gopalaswamy AM (2020) The importance of reliable monitoring methods for the management of small, isolated populations. Conservation Science and Practice 2(7), e217.
| Crossref | Google Scholar |
Farhadinia MS, Moll RJ, Montgomery RA, Ashrafi S, Johnson PJ, Hunter LTB, Macdonald DW (2018) Citizen science data facilitate monitoring of rare large carnivores in remote montane landscapes. Ecological Indicators 94, 283-291.
| Crossref | Google Scholar |
Farmer RG, Leonard ML, Mills Flemming JE, Anderson SC (2014) Observer aging and long-term avian survey data quality. Ecology and Evolution 4(12), 2563-2576.
| Crossref | Google Scholar | PubMed |
Gibb R, Browning E, Glover-Kapfer P, Jones KE (2019) Emerging opportunities and challenges for passive acoustics in ecological assessment and monitoring. Methods in Ecology and Evolution 10(2), 169-185.
| Crossref | Google Scholar |
Gibbs JP (2001) MONITOR Vol. 2023. Available at https://www.mbr-pwrc.usgs.gov/software/monitor/help_overview.html
Gibbs JP, Droege S, Eagle P (1998) Monitoring populations of plants and animals. BioScience 48(11), 935-940.
| Crossref | Google Scholar |
Gregory RD, Gibbons DW, Donald PF (2004) Bird census and survey techniques. In ‘Bird ecology and conservation: a handbook of techniques’. (Eds WJ Sutherland, I Newton, R Green) pp. 17–56. (Oxford University Press: Oxford, England) 10.1093/acprof:oso/9780198520863.003.0002
Hambler C, Newing J, Hambler K (1993) Population monitoring for the flightless rail Dryolimnas cuvieri aldabranus. Bird Conservation International 3(4), 307-318.
| Crossref | Google Scholar |
Harris JBC, Haskell DG (2013) Simulated birdwatchers’ playback affects the behavior of two tropical birds. PLoS ONE 8(10), e77902.
| Crossref | Google Scholar |
Hauser CE, Pople AR, Possingham HP (2006) Should managed populations be monitored every year? Ecological Applications 16(2), 807-819.
| Crossref | Google Scholar | PubMed |
Hill AP, Prince P, Piña Covarrubias E, Doncaster CP, Snaddon JL, Rogers A (2018) AudioMoth: evaluation of a smart open acoustic device for monitoring biodiversity and the environment. Methods in Ecology and Evolution 9(5), 1199-1211.
| Crossref | Google Scholar |
Jaramillo-Legorreta A, Cardenas-Hinojosa G, Nieto-Garcia E, Rojas-Bracho L, Ver Hoef J, Moore J, Tregenza N, Barlow J, Gerrodette T, Thomas L, Taylor B (2017) Passive acoustic monitoring of the decline of Mexico’s Critically Endangered vaquita. Conservation Biology 31(1), 183-191.
| Crossref | Google Scholar | PubMed |
Jiguet F (2009) Method learning caused a first-time observer effect in a newly started breeding bird survey. Bird Study 56(2), 253-258.
| Crossref | Google Scholar |
Kamp J, Oppel S, Heldbjerg H, Nyegaard T, Donald PF (2016) Unstructured citizen science data fail to detect long-term population declines of common birds in Denmark. Diversity and Distributions 22(10), 1024-1035.
| Crossref | Google Scholar |
Kosmala M, Wiggins A, Swanson A, Simmons B (2016) Assessing data quality in citizen science. Frontiers in Ecology and the Environment 14(10), 551-560.
| Crossref | Google Scholar |
Kotila M, Suominen KM, Vasko VV, Blomberg AS, Lehikoinen A, Andersson T, Aspi J, Cederberg T, Hänninen J, Inkinen J (2023) Large-scale long-term passive-acoustic monitoring reveals spatio-temporal activity patterns of boreal bats. Ecography 2023, e06617.
| Crossref | Google Scholar |
Legge S, Robinson N, Lindenmayer D, Scheele B, Southwell D, Wintle B (2018) Introduction: making it count. In ‘Monitoring threatened species and ecological communities’. (Eds S Legge, D Lindenmayer, N Robinson, B Scheele, D Southwell, B Wintle, JC Woinarski, E Bayraktarov) pp. 1–10. (CSIRO Publishing: Melbourne, Australia) 10.1071/9781486307722
Lewandowski E, Specht H (2015) Influence of volunteer and project characteristics on data quality of biological surveys. Conservation Biology 29(3), 713-723.
| Crossref | Google Scholar | PubMed |
Lindenmayer D, Likens G (2018) ‘Effective ecological monitoring.’ (CSIRO publishing: Melbourne, Australia) 10.1071/9781486308934
Lindenmayer DB, Gibbons P, Bourke M, Burgman M, Dickman CR, Ferrier S, Fitzsimons J, Freudenberger D, Garnett ST, Groves C, Hobbs RJ, Kingsford RT, Krebs C, Legge S, Lowe AJ, Mclean R, Montambault J, Possingham H, Radford J, Robinson D, Smallbone L, Thomas D, Varcoe T, Vardon M, Wardle G, Woinarski J, Zerger A (2012) Improving biodiversity monitoring. Austral Ecology 37(3), 285-294.
| Crossref | Google Scholar |
Lindenmayer D, Michael D, Crane M, Florance D (2018) Ten lessons in 20 years: insights from monitoring fauna and temperate woodland revegetation. Ecological Management & Restoration 19, 36-43.
| Crossref | Google Scholar |
Lor S, Malecki RA (2002) Call-response surveys to monitor marsh bird population trends. Wildlife Society Bulletin 30, 1195-1201.
| Crossref | Google Scholar |
Marion WR, O’Meara TE, Maehr DS (1981) Use of playback recordings in sampling elusive or secretive birds. Studies in Avian Biology 6, 81-85.
| Google Scholar |
Marques TA, Thomas L, Martin SW, Mellinger DK, Ward JA, Moretti DJ, Harris D, Tyack PL (2013) Estimating animal population density using passive acoustics. Biological Reviews 88(2), 287-309.
| Crossref | Google Scholar | PubMed |
Marsland S, Priyadarshani N, Juodakis J, Castro I (2019) AVIANZ: a future-proofed program for annotation and recognition of animal sounds in long-time field recordings. Methods in Ecology and Evolution 10(8), 1189-1195.
| Crossref | Google Scholar |
Mielke A, Zuberbühler K (2013) A method for automated individual, species and call type recognition in free-ranging animals. Animal Behaviour 86(2), 475-482.
| Crossref | Google Scholar |
Molnár C, Kaplan F, Roy P, Pachet F, Pongrácz P, Dóka A, Miklósi Á (2008) Classification of dog barks: a machine learning approach. Animal Cognition 11, 389-400.
| Crossref | Google Scholar | PubMed |
Nisbet E (2007) Cinderella science. Nature 450(7171), 789-790.
| Crossref | Google Scholar | PubMed |
Olsen J, Hayes G (2002) Vocalisations used by Southern Boobooks (Ninox novaeseelandiae) in the Australian Capital Territory. In ‘Ecology and conservation of owls’. (Eds I Newton, R Kavanagh, J Olsen, I Taylor) p. 305. (CSIRO Publishing: Melbourne, Australia) 10.1071/9780643069886
Pérez-Granados C, Schuchmann K-L (2021) Passive acoustic monitoring of Chaco Chachalaca (Ortalis canicollis) over a year: vocal activity pattern and monitoring recommendations. Tropical Conservation Science 14, 19400829211058295.
| Crossref | Google Scholar |
Prince P, Hill A, Piña Covarrubias E, Doncaster P, Snaddon JL, Rogers A (2019) Deploying acoustic detection algorithms on low-cost, open-source acoustic sensors for environmental monitoring. Sensors 19(3), 553.
| Crossref | Google Scholar |
Pryde MA, Greene TC (2016) Determining the spacing of acoustic call count stations for monitoring a widespread forest owl. New Zealand Journal of Ecology 40(1), 100-107.
| Crossref | Google Scholar |
Pryde MA, Mortimer JAJ, Greene TC, Thygesen HH (2020) Optimising monitoring times for surveys of rūrū (Ninox novaeseelandiae novaeseelandiae). New Zealand Journal of Ecology 44(1), 3401.
| Crossref | Google Scholar |
R Core Team (2023) R: a language and environment for statistical computing (4.2.2). R Foundation for Statistical Computing. Available at https://www.r-project.org/
Ribeiro JW, Jr., Sugai LSM, Campos-Cerqueira M (2017) Passive acoustic monitoring as a complementary strategy to assess biodiversity in the Brazilian Amazonia. Biodiversity and Conservation 26(12), 2999-3002.
| Crossref | Google Scholar |
Rohner C (1997) Non-territorial ‘floaters’ in Great Horned Owls: space use during a cyclic peak of Snowshoe Hares. Animal Behaviour 53(5), 901-912.
| Crossref | Google Scholar |
Severinghaus LL (2000) Territoriality and the significance of calling in the Lanyu Scops Owl Otus elegans botelensis. Ibis 142(2), 297-304.
| Crossref | Google Scholar |
Shonfield J, Bayne EM (2017) Autonomous recording units in avian ecological research: current use and future applications. Avian Conservation and Ecology 12(1), 14.
| Crossref | Google Scholar |
Sorrell KJ, Clarke RH, Holmberg R, McIntosh RR (2019) Remotely piloted aircraft improve precision of capture–mark–resight population estimates of Australian fur seals. Ecosphere 10(8), e02812.
| Crossref | Google Scholar |
Southwell DM, Smart A, Merson SD, Selwood KE, Macgregor NA (2024) Using power analysis and spatial prioritization to evaluate the design of a forest bird monitoring programme. Oryx 58, 522-531.
| Crossref | Google Scholar |
Sperring V, Brown S, Macgregor N, Olsen P, Clarke R, Wilson M, Greenup N, Weeks A, Ward R, Greenwood D, Christian M, Garnett S (2021) Norfolk Island Morepork Ninox novaeseelandiae undulata. In ‘The action plan for Australian birds 2020’. (Eds S Garnett, G Baker) pp. 360–363. (CSIRO Publishing: Melbourne) 10.1071/9781486311910
Sperring VF, Bradsworth N, Isaac B, Brown S, Wilson M, Macgregor NA, Clarke RH (2024a) Habitat requirements of a Critically Endangered Ninox hawk-owl: implications for island-wide restoration. Restoration Ecology 32, e14284.
| Crossref | Google Scholar |
Sperring VF, Weeks AR, Webster W, Macgregor NA, Wilson M, Isaac B, Clarke RH (2024b) Diet breadth of a critically endangered owl presents challenges for invasive rodent management: a conservation conundrum. Emu - Austral Ornithology 124, 187-198.
| Crossref | Google Scholar |
Sugai LSM, Silva TSF, Ribeiro JW, Jr, Llusia D (2019) Terrestrial passive acoustic monitoring: review and perspectives. BioScience 69, 15-25.
| Crossref | Google Scholar |
Viola BM, Sorrell KJ, Clarke RH, Corney SP, Vaughan PM (2022) Amateurs can be experts: a new perspective on collaborations with citizen scientists. Biological Conservation 274, 109739.
| Crossref | Google Scholar |
Vrezec A, Bertoncelj I (2018) Territory monitoring of Tawny Owls Strix aluco using playback calls is a reliable population monitoring method. Bird Study 65(sup1), S52-S62.
| Crossref | Google Scholar |
Watson DM, Znidersic E, Craig MD (2019) Ethical birding call playback and conservation. Conservation Biology 33(2), 469-471.
| Crossref | Google Scholar | PubMed |
Westgate MJ, Scheele BC, Ikin K, Hoefer AM, Beaty RM, Evans M, Osborne W, Hunter D, Rayner L, Driscoll DA (2015) Citizen science program shows urban areas have lower occurrence of frog species, but not accelerated declines. PLoS ONE 10(11), e0140973.
| Crossref | Google Scholar |
Wickham H, François R, Henry L, Müller K, Vaughan D (2023) dplyr: a grammar of data manipulation, R package version 1.1.4. Available at https://github.com/tidyverse/dplyr
Wolfgang A, Haines A (2016) Testing automated call-recognition software for winter bird vocalizations. Northeastern Naturalist 23(2), 249-258.
| Crossref | Google Scholar |
Wood CM, Gutiérrez RJ, Keane JJ, Peery MZ (2020) Early detection of rapid Barred Owl population growth within the range of the California Spotted Owl advises the Precautionary Principle. The Condor 122(1), duz058.
| Crossref | Google Scholar |
Yoccoz NG, Nichols JD, Boulinier T (2001) Monitoring of biological diversity in space and time. Trends in Ecology & Evolution 16(8), 446-453.
| Crossref | Google Scholar |
Zuberogoitia I, Zabala J, Martínez JE (2011) Bias in little owl population estimates using playback techniques during surveys. Animal Biodiversity and Conservation 34(2), 395-400.
| Crossref | Google Scholar |
Zuberogoitia I, Martínez JE, González-Oreja JA, de Buitrago CG, Belamendia G, Zabala J, Laso M, Pagaldai N, Jiménez-Franco MV (2020) Maximizing detection probability for effective large-scale nocturnal bird monitoring. Diversity and Distribution 26, 1034-1050.
| Crossref | Google Scholar |
Zwerts JA, Stephenson PJ, Maisels F, Rowcliffe M, Astaras C, Jansen PA, van der Waarde J, Sterck LEHM, Verweij PA, Bruce T, Brittain S, van Kuijk M (2021) Methods for wildlife monitoring in tropical forests: comparing human observations, camera traps, and passive acoustic sensors. Conservation Science and Practice 3(12), e568.
| Crossref | Google Scholar |