Indicators of functional integrity in production animals
I. G. Colditz


A Agriculture and Food, CSIRO, Armidale, NSW, Australia.
B School of Environmental and Rural Science, University of New England, Armidale, NSW, Australia.
C Agriculture and Food, CSIRO, 306 Carmody Road, St. Lucia, Qld 4067, Australia.
Animal Production Science 63(9) 825-843 https://doi.org/10.1071/AN23029
Submitted: 16 January 2023 Accepted: 11 May 2023 Published: 5 June 2023
© 2023 The Author(s) (or their employer(s)). Published by CSIRO Publishing. This is an open access article distributed under the Creative Commons Attribution-NonCommercial-NoDerivatives 4.0 International License (CC BY-NC-ND)
Abstract
An ability to describe the quality of lived experience across the course of an animal’s life is of increasing interest in studies on wellbeing in farm animals. Integrity of physical functions is an important aspect of the tripartite model of wellbeing: feeling, functioning and interacting well. This review examines measures of functional integrity as potential indicators of functional (eudaimonic) wellbeing. Copious literature shows that coping with day-to-day fluctuations in the environment influences (1) periodicity of biorhythms, (2) allocation of resources to growth, defence and repair, and (3) rate of biological aging. Here we review opportunities for assessing functional integrity through analysis of (1) the dynamic status of physiological and behavioural variables, (2) the accretion of resources in production tissues (milk, eggs, body mass, offspring) and physical structures (fibre, hoof, bone, tooth, placoid scale, otolith, beak), and (3) biological age. A reduction in periodicity of biorhythms, complexity of behaviours, or uniformity of trajectories of growth and production is often associated with poorer current and future health outcomes and shorter functional longevity. These analytical approaches are grounded in statistical measures of stability in complex dynamic systems. Additionally, the cumulative effect of wear and tear on biological age can be assessed via telomere length, DNA methylation, biomarkers of allostatic load, hair cortisol, and physiological functional capacity. Timeseries of behaviour, physiology and accretion provide a richer record of functional integrity than do cumulative indicators of biological age. Indicators of integrity are needed for each phase of the animal’s life course including attainment of developmental competencies, stability of mature performance, and rate of decline with advancing age, to provide a comprehensive record across the whole of the animal’s life. The utility of measures may differ between real-time and retrospective assessments and between application to every individual versus sentinels. Further validation is required for interpretating measures of functional integrity as indicators of the functional aspect of wellbeing, together with determining their practicality and reliability before translation to commercial settings is feasible. A single type of indicator of functional wellbeing (e.g. DNA methylation) is unlikely to have utility for all life stages, species, production systems, and environments.
Keywords: affective state, allostasis, allostatic load, animal welfare, Apgar score, DNA methylation, eudaimonic wellbeing, hedonic wellbeing, homeorhesis, homeostasis, intrinsic capacity, poverty groove, quality of life, resilience, rhinotheca, sclerochronology, striae of Retzius, telomere length, trophic tone, wellbeing, Wilson bands.
Introduction
The capacity of the animal to cope with environmental fluctuations is an important aspect of its welfare (Broom 1996). Most early work on assessing the welfare status of animals relied on assessments taken on a subset of a population at infrequent intervals to provide a snapshot of the status of the group (for commentaries, see Webster 2016; Keeling et al. 2021). A more challenging goal is to describe the quality of the lived experience of each animal across the whole of its life (Yeates 2011; Mellor 2016; Bateson and Poirier 2019; Vigors et al. 2021). A strong framework has been developed for assessing the opportunities provided by environmental resources as inputs that can enable animals to have positive experiences (Edgar et al. 2013; Rowe and Mullan 2022; Stokes et al. 2022). Nonetheless, outcomes evidenced in animal-based measures are recognised as an important complement to resource-based inputs in assessment of the quality of lived experience (Main et al. 2007; Lawrence et al. 2019; Turner 2019; Keeling et al. 2021; Vigors et al. 2021; Stokes et al. 2022). Outcome-based indicators are particularly attractive if they provide an integration over extended periods to time of the quality of lived experience and can be assessed near to or at the end of the animal’s life (Grandin 2017; Bateson and Poirier 2019; Bradshaw 2019). Hence, the development of outcome-based indicators of quality of life is an important challenge for research on animal welfare and wellbeing (see Table 1 for a glossary of terms written in italic font).
Term | Meaning |
Affective state | The psychological state arising from a balance between positives and negatives within each of valence, motivation to act and arousal. |
Allostasis | ‘Stability through change’. In the context of short-term environmental conditions that persist from moments to hours, allostasis describes the anticipatory regulation of behavioural and physiological activities that prepare the animal for imminent threats and impending demands that may perturb the homeostatic state from its normal range. Over longer time frames extending from days to weeks, a sustained environmental load can lead to reactive adaptive changes in physiological and behavioural activities (plasticity) that is also termed allostasis. Allostasis, as defined in the literature, can lead to maladaptive behavioural and physiological states such as stereotypies and Type 2 diabetes. |
Allostatic load | The burden placed on regulatory processes when physiological and behavioural variables are persistently driven outside their normal operating range due to unfavourable environmental conditions. |
Biological age | The location of an animal along its life course. Animals in a population can age at different rates, hence the biological age of an individual can differ from its chronological age. |
Chronological age | The average rate of progression of animals in a population along their life course, measured in chronological units of time. |
Functional integrity | The degree to which physiological, behavioural, and immunological activities enable the animal to flourish. In a broader sense, functional integrity includes the instrumental roles of affective state, cognition and memory in regulatory performance. This functional role contrasts with the qualitative role of affect in subjective hedonic experience. |
Homeorhesis | The process whereby the sensitivity and metabolic activities of tissues to anabolic and catabolic signals is changed in anticipation of emerging physiological demands such as ontogenetic development, pregnancy and lactation. Homeorhetic changes are driven by genetic programs for physiological and behavioural functioning. Homeorhesis prescribes the trajectories of performance that an animal springs back towards when environmental conditions become more favourable such as compensatory growth. |
Homeostasis | The continuously dynamic outcome state within the internal environment that arises from anticipatory allostatic signalling and reactive homeostatic reflexes. Anticipatory and reactive signalling modulates activities in physiological, immunological, behavioural, cognitive and affect systems in order to maintain homeostasis. Balance within the homeostatic state arises within the context of somatic tissue sensitivity and metabolic activity in response to signals. These somatic tissue dynamics are pre-conditioned by homeorhetic and allostatic processes. |
Indicators of functional integrity | Statistical measures of dynamic stability in physiological, behavioural, and immunological activities, especially those evident in the face of day-to-day (quotidian) fluctuations in the environment. Theoretically, it is considered that affective state is an aspect of regulatory performance that helps guide the animal towards homeostatic integrity and hence is an aspect of functional integrity. From this functional perspective, the importance to the animal of the quality of the felt experience of affect may differ from its functional role. Hence, suffering matters beyond its functional consequence. Similarly, functioning has consequences beyond its affective experience. |
Intrinsic capacity | A concept developed by WHO to describe functional status in humans, intrinsic capacity is a composite of all the physical and mental (including psychosocial) capacities that an individual can draw on at any point in time. Intrinsic capacity interacts with characteristics of the individual’s environment to determine their functional ability. Healthy aging, in this view is the process of developing and maintaining the functional ability that confers resilience and enables wellbeing in older age. |
Positive welfare | Most descriptions of positive welfare describe it as a positive state that arises when positive hedonic experiences are more prevalent than negative experiences. In more detail, positive welfare is described in terms of positive emotions, positive affective engagement, quality of life, and happiness. Some descriptions recognise positive physical functioning as an aspect of positive welfare, and hence align very closely with the concept of wellbeing. |
Regulatory performance | How well the animal accomplishes the task of maintaining a state conducive to thriving. |
Resilience | The capacity to maintain or regain functional integrity in the face of environmental disturbances. |
Trophic tone | The balance in the systemic metabolic environment between anabolic and catabolic signals that influences the allocation of resources to trophism (growth) or defence and repair. Principal sources of trophic signals are the autonomic nervous system, the somatotropic axis and the immune system. The microbiome and pathogens are also sources of signals with direct and indirect effects on trophic tone. |
Welfare | A tripartite concept describing the state of the animal as it attempts to cope with its environment in terms of feeling well, functioning well and living a natural life. Welfare extends from poor to positive. |
Wellbeing | A tripartite concept describing the positive aspects of physical functioning, hedonic experience, and social interactions. |
The functional capacity of the animal to cope with day-to-day variations and events in its environment may provide a window on the quality of its lived experience (Bateson and Poirier 2019; Bradshaw 2019; Turner 2019; Colditz 2023). Several approaches can be taken when assessing the functional integrity of the animal on a day-by-day basis. The first is to analyse the dynamic status of physiological and behavioural variables from high frequency or continuous records (Sun et al. 2021; Wagner et al. 2021). The second approach is to analyse the accretion of resources in production tissues such as muscle, milk, eggs and wool, and in physical structures such as bone and teeth (Neville 1967; Smolyar and Bromage 2004). A third approach is to estimate the biological age of the animal (Bateson and Poirier 2019). Proposed indicators of biological age include DNA methylation and telomere length, changes in functional capacity of physiological and behavioural systems, and biomarkers of ‘wear and tear’. Utility of these approaches may differ among life stages and be influenced by the purpose for which assessment is undertaken. This narrative review begins with a brief description of the physiological context in which daily variation in functional integrity occurs. It then examines the approaches described above for assessing functional integrity. The potential insights into lifetime wellbeing provided by these measurements are examined. Finally, some further steps needed to refine and validate measurements of lifetime wellbeing are suggested.
Physiological context for variation in functional integrity
Resources and threats present in an animal’s internal and external environments vary in both predictable and unpredictable ways on timescales that fluctuate from moment-to-moment to the whole of the animal’s lifespan. Whenever possible, the animal seeks to minimise disturbances to its internal environment through prediction and control of perturbations (Moore-Ede 1986; Jensen and Toates 1997; Sterling 2012; Boissy and Erhard 2014; Colditz 2018; Kristiansen and Fernö 2020). So as to maintain the internal environment in a homeostatic state, the supply of metabolic substrates that are needed to support maintenance and growth is continually balanced against the need to provide resources to support defence and to repair damage caused by accidents and environmental threats (Ye and Medzhitov 2019). The trophic tone of growth factors and nutrients in the animal’s internal environment continuously modulates allocation of resources among growth, defence and repair (Fig. 1; Schulkin and Sterling 2019). The roles of prediction and control are illustrated by the daily light–dark cycle which synchronises physiological and behavioural activities of the animal with the availability of resources and the risk of threats (Moore-Ede 1986; Plaut and Casey 2012; Spiga and Lightman 2020; Casey and Plaut 2022). Additional patterns of resource availability and physiological demand are predicted by seasons and by the impending development of physiological states such as pregnancy and lactation (Fig. 1; Bell and Bauman 1997; Casey and Plaut 2022). In addition to inherited methods of prediction such as circadian and seasonal clocks, learning enables the animal to associate certain internal and external cues with the imminent appearance of resources, threats and physiological demands (Deans 2021). This enables the animal to undertake anticipatory activities to minimise the disturbances these events might otherwise bring (Sterling 2012; Kristiansen and Fernö 2020). Predictable patterns in the availability and utilisation of resources can be disrupted by threats and damage from adventitious events such as infections, injury and predation. These unanticipated disruptions can induce a facultative catabolic state in which resources are drawn from body reserves in support of defence and repair (Fig. 1; Elsasser et al. 1997; Colditz 2002; Ye and Medzhitov 2019). The capacity of the animal to predict, control and accommodate environmental variations influences the dynamic balance between anabolism and catabolism that constitutes the trophic state of the animal. Sensitivity of tissues to anabolic and catabolic signals and activity of metabolic pathways are also modulated as an aspect of the trophic tone of the animal (Bell and Bauman 1997; Casey and Plaut 2012).
The flow of metabolic resources from inside and outside the animal into biological functions is modulated by systemic trophic tone. Metabolic resources (inputs, left column) are drawn from the diet, body stores and the microbiome. A systemic trophic balance between anabolism and catabolism is tuned by three principal axes: autonomic, somatotropic and immunologic that generate systemic trophic tone (centre box). The balance between anabolism and catabolism exhibits temporal dynamics that synchronise predictable availability of resources with predictable somatic demands for those resources (right of box). Prominent cycles in supply and demand exhibit circadian, seasonal and life-history patterns. Unpredicted disturbances to supply and demand associated with environmental stressors, injury, disease, social isolation, etc. recruit facultative adaptations that disrupt predicted patterns. Sinks utilising resources are shown in the right column. The balance of anabolic and catabolic activities is also influenced by tissue sensitivity to trophic signals and the activation status of metabolic pathways. Figure style adapted from Schiller et al. (2022).
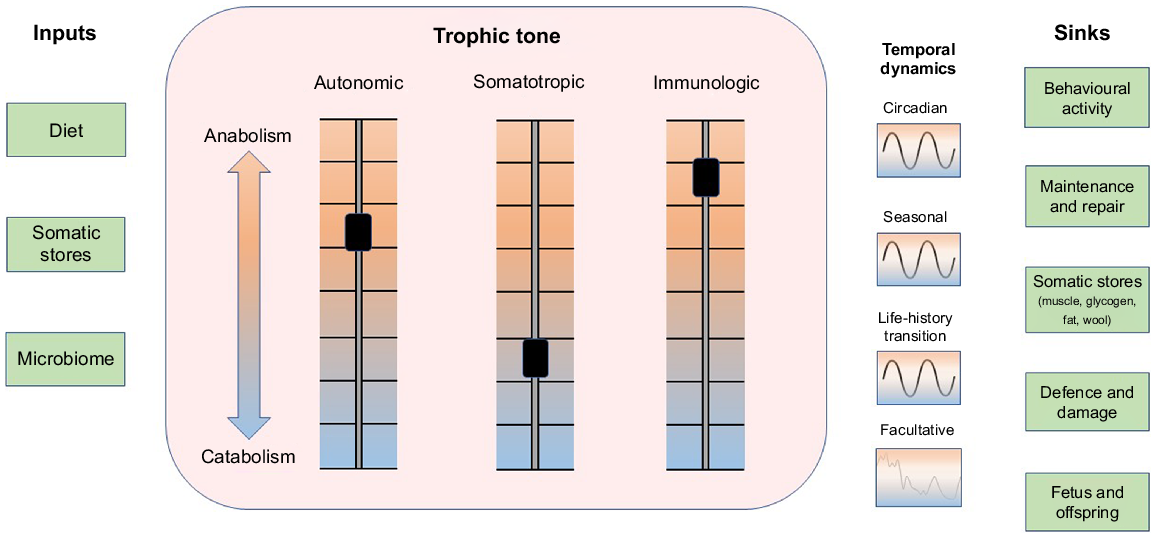
The mediators of anabolism and catabolism can act with a high degree of local tissue specificity when they are released within target organs as autocrine or paracrine signals or from autonomic nerve endings. In addition, endocrine and cytokine mediators of anabolism and catabolism can be distributed systemically via blood to induce global changes in the animal’s metabolic economy. An important influence on trophic tone is associated with the affective state of the animal. Three aspects of affective state are described as valence, motivation to act, and arousal (Fraser and Duncan 1998; Mendl et al. 2010; Schiller et al. 2022). The state that arises from these internal conditions modulates behavioural activity, cognition, and the release of trophic signals from the autonomic nervous system (including the adrenal medulla), somatotropic axis (including adrenal cortex) and the immune system (Fig. 2; Schiller et al. 2022). Affective state exhibits temporal patterns that include short-term changes from moment-to-moment described as emotions, longer trends that persist for hours to days described as moods, and a more persistent balancing point of the three aspects of affect that is associated with the temperament or personality of the animal (Mendl et al. 2010; Boissy and Erhard 2014; Schiller et al. 2022). The long-term trend and cumulative balance of affect is considered to be an important indicator of wellbeing (Fig. 2; Colditz 2022).
Processing of information from inside and outside the animal generates a systemic affective state. Information flows from internal and external environments via neural, hormonal, cytokine and small molecular messengers (e.g. microbiome and pathogen signalling molecules) via sensory pathways of exteroception, interoception, proprioception, and humoral transport (Inputs, left column). Three aspects of affective state are valence, motivation to act and arousal, which can independently vary from positive to negative (Schiller et al. 2022) and together generate a dynamic systemic affective state (central box). Affective state exhibits both facultative and predictable dynamics, which change on time scales from moment to moment (emotions), over several hours (moods), or show circadian, and longer-term trajectories associated with wellbeing and temperament (Colditz 2022). Seasonal dynamics in affective state seen in humans (e.g. seasonal affective disorder) may also occur in animals such as happy cows in spring time (Lindahl et al. 2012; not shown). Affective state modulates behavioural activity, autonomic tone, somatotropic axis, immune function, cognition and microbiome (right column) and, hence, affective state plays an overarching role as a synopsis of the ‘information context’ within which supply and demand cycles and trophic tone operate, as depicted in Fig. 1. Figure style has been adapted from Schiller et al. (2022).
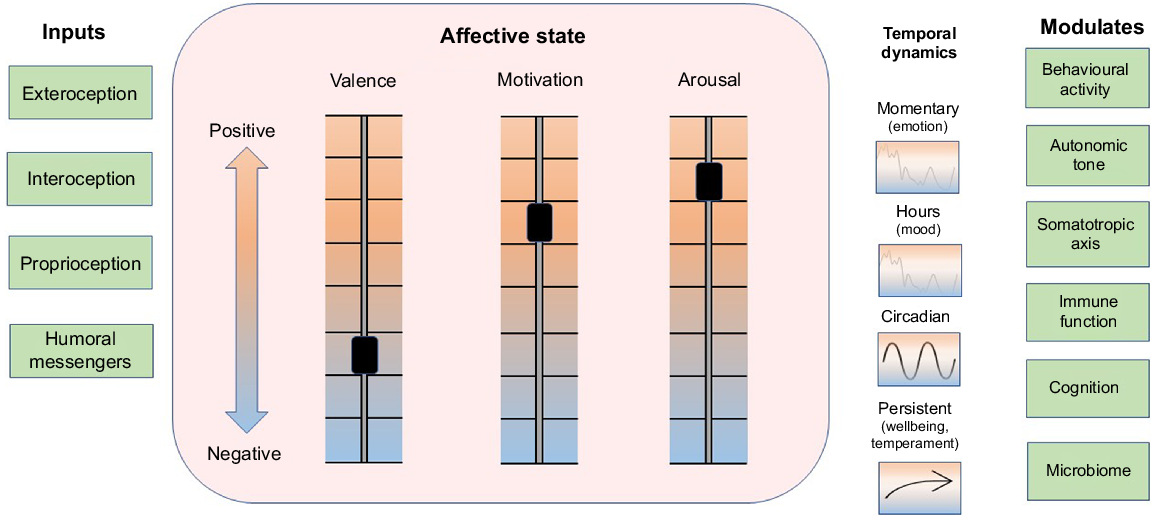
The capacity of the animal to minimise interruptions to its cyclical patterns of physiological and behavioural activity and to quickly re-establish these patterns in the face of disturbances is described as resilience (Colditz and Hine 2016; Berghof et al. 2019a; Basile et al. 2021). A consequence of the animal’s ability to maintain integrity in physiological and behavioural activities is continuity in supply of metabolic resources to support anabolic activities associated with growth, production and reproduction (Neville 1967). Daily fluctuations in homeostatic balance are written down in incremental patterns of growth, production, and accretion of resources in hard tissues of the body. This dynamic character of homeostatic balance underwrites the potential for measures of functional integrity to inform assessment of the wellbeing of the animal as it engages with day-to-day variations in its environment.
Analysis of physiological and behavioural variables
Individuals within a group express persistent differences in the homeostatic balance of their physiological and behavioural activities (Careau et al. 2008). These differences can be observed as among-animal variation in measures such as concentrations of metabolites and behavioural activities such as duration of feeding bouts (Herd and Arthur 2009). For many years, selective breeding for traits such as temperament, production and residual feed intake (feed efficiency) has drawn on these differences in homeostatic balance to modify the characteristics of offspring. More recently, high-frequency monitoring of behavioural and physiological variables has shown that a capacity to maintain homeostasis in the face of short-term environmental fluctuations also differs among individuals (Scheibe et al. 1999; Nunes Marsiglio Sarout et al. 2018; Rao and Androulakis 2019). Indicators of homeostatic integrity include the periodicity of biorhythms and the dynamic complexity of system functions such as behaviours and affective state. The degree of synchronisation of behaviours and physiological variables within the 24 h circadian cycle is decreased when current and future health status and some production outcomes are poorer in free-grazing sheep (Nunes Marsiglio Sarout et al. 2018), calves (Veissier et al. 1989; Sun et al. 2021; Rhodes et al. 2022) and cows (van Dixhoorn et al. 2018; Wagner et al. 2021). Many methods have been developed for analysing the strength of periodicity of biorhythms (e.g. Scheibe et al. 1999; Wagner et al. 2021; Casey and Plaut 2022; Rhodes et al. 2022). In addition to changes in periodicity of biorhythms, the temporal complexity of behaviours (Asher et al. 2009) is decreased during periods of stress and disease in livestock (Burgunder et al. 2018; Herborn et al. 2020), zoo animals (Miller et al. 2020) and wild species (MacIntosh et al. 2011). For reviews of biorhythms in ruminants, see Casey et al. (2022) and Goh et al. (2019).
From an evolutionary perspective, a functional or instrumental role of affective state in guiding the animal towards synchrony with its environment has been frequently proposed (e.g. Fraser and Duncan 1998; Mendl et al. 2010; Trimmer et al. 2013). One aspect of the functional role of affect is illustrated by periodicity in affective states. Humans exhibit a circadian pattern of positive mood (Porto et al. 2006) that is closely coupled to the circadian pattern of glucocorticoid secretion (Kalafatakis et al. 2021). Environmental and pharmacological manipulations of the circadian glucocorticoid cycle lead to disruption of the pattern of positive mood in humans (Kalafatakis et al. 2021) that, in a rat model, is associated with a decrease in resilience to environmental stressors (Spiga and Lightman 2020). A second feature of affect dynamics is the daily diversity (complexity) in both positive and negative affective episodes (Quoidbach et al. 2014). Drawing inspiration from the contribution of complexity to resilience within ecosystems, studies in humans have found that greater daily diversity and abundance in both positive and negative affective episodes is favourably associated with better wellbeing (Quoidbach et al. 2014; Urban-Wojcik et al. 2022). In addition to the focus in this paper on physical functions, the circadian patterning of affective states raises the possibility that analysis of the periodicity and complexity of biophysical indicators of affect (Keeling et al. 2021) may provide indicators of the instrumental role of functional integrity of the animal’s affective state in achieving beneficial wellbeing outcomes.
Accretion of resources in anatomical structures and animal products
A downstream consequence of fluctuations in trophic balance is short-term variations in the rate of accretion of resources in production tissues such as muscle, wool and milk, and in the anatomical hard structures that support these tissues such as bone and teeth (Neville 1967). These physical products of the animal can therefore provide an archive of success in maintaining a positive trophic balance within the internal environment. Three of the approaches described in the literature for assessing variations in the rate of accumulation of resources in tissues are isotope analysis, tissue morphology and yield of animal products. These approaches are part of a widely used strategy in biology of studying variability in incremental growth patterns as an indicator of daily variation in internal and external environments and life-history transitions of the organism (Neville 1967; Smolyar and Bromage 2004; Grønkjær 2016). More recently, this approach has been applied to analysis of variability in short-term output of production variables such as body mass, milk yield, egg production, and fibre diameter.
Isotope analysis
The abundance of stable isotopes in living and dead tissues that are metabolically inert once they are laid down reflects the environmental and physiological conditions experienced at the time of tissue growth (Schwertl et al. 2003; Zazzo et al. 2005; Zazzo et al. 2007). Mineralised tooth and bone, and keratinised tissues including hoof, hair, wool, claw and beak are especially sensitive to these effects.
The analysis of stable isotope concentrations in tissues supports authentication of production systems (e.g. organic versus conventional), geographic and species provenance (e.g. is this meat from France or Australia and is it lamb or beef?), dietary composition, efficiency of use of available resources such as nitrogen, and the previous occurrence of physiological stressors. In cattle, tail hair and hoof can contain information extending up to 15 months prior to sample collection and both tissues can be analysed by stable isotope assays to a resolution of about 5-day intervals (Zazzo et al. 2007). The growth rate of hair and hoof is influenced by genetics as well as nutrition, season, temperature, housing, physiological status, and the occurrence of internal and external stressors that modify metabolic status (Hahn et al. 1986; Ruegg 2000; Greenough 2001; Schwertl et al. 2003). A focus of stable isotope analyses in ruminants has been on reconstructing the nutritional status and dietary composition of free-grazing animals (Schwertl et al. 2003; Li et al. 2012; Rysava et al. 2016), and on detecting the prior occurrence of major stressful events such as seasonal starvation (Rysava et al. 2016). More recently, stable isotope analyses of tail fibres have been used to assesses nitrogen-use efficiency in cattle (Silva et al. 2022). In this study, persistent differences were observed among individuals in their metabolic efficiency in use of nitrogen. These persistent individual differences highlight the need for changes in isotope concentrations to be assessed against each individual animal’s own baseline.
Stable isotope analysis of bovine and ovine teeth can be undertaken with a spatial resolution of 10 μm; however, the spatio-temporal process of mineralisation is complex and, hence, inferring the temporal sequence from spatial data can be challenging (Zazzo et al. 2005). As for keratinised tissues, most isotope studies on teeth have used the record of mineralisation to reconstruct diets, husbandry practices and the seasonal timing of major life-history events such as birth and weaning in archaeological samples (e.g. Balasse et al. 2012; Towers et al. 2014).
Morphological analysis
Growth patterns in hard tissues leave morphological evidence of fluctuations in trophic tone (Neville 1967). Sequential lines termed striae of Retzius are laid down in teeth during mineralisation. Physiological stressors such as parasitism in sheep accentuate these marks which, when more visibly prominent, are termed Wilson bands (Suckling 1989; Goodman and Rose 1990). Again, most studies of striae and hypomineralisation defects such as Wilson bands have been undertaken on archaeological samples of wild and domesticated animals (e.g. Mainland et al. 2016).
The quality of hoof epidermis is very sensitive to the physiological status of the animal during keratinisation (Tomlinson et al. 2004). Stressors, especially nutritional disturbances, can lead to the development of horizontal grooves termed poverty lines as well as deeper fissures in the hoof surface (Greenough 2001). Similar horizontal stress lines in finger nails of humans are termed Beau’s lines (Zaiac and Walker 2013). In a research dairy, Ruegg (2000) found that greater than 90% of cows without horizontal grooves on both hind feet had no history of either clinical disease or feed disturbances associated with other studies undertaken in the facility. Animals in which horizontal grooves were present were five to six times more likely to have a record of clinical disease or feed disturbance. Foot health is a prominent welfare indicator and has been proposed as an indicator for retrospective assessment of the welfare history of animals at the time of slaughter (Llonch et al. 2015; Grandin 2017). In an abattoir survey in northern Mexico, Bautista-Fernández et al. (2021) observed abnormal claw shape in more than 55% and fissures in more than 25% of cattle (n = 1040) from rangeland, feedlot and intensive dairy production systems. The presence of severe claw disorders was a significant predictor of final muscle pH of >6.0.
During the anagen phase of fibre growth, conditions prevailing in the internal and external environments influence both the rate of wool-fibre elongation and the diameter of the fibre produced (Masters et al. 1998; Rogers 2006). In sheep breeds such as Merinos that are locked into a persistent anagen phase of fibre growth, wool can provide a continuous record of trophic tone. In most sheep production systems, wool is harvested annually and therefore contains a record of physiological status over the preceding 12 months. Instrumentation has been developed to measure the variation of fibre diameter along the wool staple (bundle of wool fibres) at 5 mm increments with an average diameter at each increment estimated for the sample of fibres (Baxter et al. 1992). While finer measurement resolutions (1–2 mm) can be achieved on clean (grease-free) wool staples, providing a temporal resolution of 2–6 days (depending on the wool growth rate), measurements are more readily performed on greasy wool staples at 5 mm increments and are adequate for ranking animals for differences in longitudinal fibre-diameter variation (Gloag and Behrendt 2002). Thus far, studies have examined the influence of breed, nutrition, husbandry practices and prevailing seasonal conditions on longitudinal fibre-diameter variation (Adams and Briegel 1998; Brown et al. 2002; Gonzalez et al. 2020). Fibre-diameter profiles differ among animals within a flock (Brown et al. 2002) and may therefore provide an individualised record of the animal’s experience over the preceding year, as seen for other production variables described below. Analysis of longitudinal fibre-diameter profiles in cattle hair does not appear to have been reported in the literature.
The incremental structure of fish scales and otoliths provide a record of daily growth and have been used for many years to assess growth rates and age in fish (Smolyar and Bromage 2004; Grønkjær 2016). Analysis of daily variability in growth recorded in these structures and in the rhinotheca of the chicken beak (Urano et al. 2019) may have utility for assessing functional integrity.
Production variables
An outcome of homeostatic regulation is the availability of resources for accretion in body mass, eggs, milk, wool and offspring. Differences occur among individuals within a management group in the day-to-day variability observed in growth rate (Berghof et al. 2019b; Mengistu et al. 2022), milk yield (Elgersma et al. 2018; Adriaens et al. 2020; Poppe et al. 2020; Poppe et al. 2021) and egg production (Bedere et al. 2022). The magnitude of day-to-day deviations from the individual animal’s performance trajectory is associated with health outcomes (Berghof et al. 2019a; Putz et al. 2019) and longevity (Adriaens et al. 2020; Poppe et al. 2022), and has been interpreted as an indicator of resilience to day-to-day fluctuations in the production environment (Colditz and Hine 2016; Friggens et al. 2017; Berghof et al. 2019a; Garcia-Baccino et al. 2021). Support for this conclusion that short-term variability in production is associated with ability to cope with fluctuations in environmental conditions comes from several sources. Piglets raised in an enriched environment display less variability in growth rate during exposure to experimental stressors than do conventionally raised piglets (Parois et al. 2022). Cows with less variability in daily milk yield across a lactation exhibit a smaller drop in milk yield when exposed to a disturbance associated with animal management practices that are implemented at the herd level than do cows with greater daily variability in milk production (Poppe et al. 2021). Daily variations in performance are often poorly synchronised within the group, which suggests that individual animals are often responding to their individual experience of their personal local environment rather than responding to the environmental conditions shared by the group (Ben Abdelkrim et al. 2021; Poppe et al. 2021). Behavioural studies have indicated that animals housed in a shared environment experience a personal ‘non-shared’ environment within the communal space (Freund et al. 2013). These observations support the conclusion that cumulative measures of daily variability around a performance trajectory across the whole of an animal’s life may provide a cumulative indicator of the quality of the individual animal’s day-to-day experience of their environment. Importantly, in these analyses it is within-animal variation that provides an indicator of functional integrity rather than the absolute level of production (Colditz 2022). Thus, these observations do not imply that higher production is itself an indicator of welfare.
Biological age (wear and tear)
The life course of an animal follows a trajectory encompassing phases of development, maturity and senescence (Horvath and Raj 2018; Pyrkov et al. 2021). The pathway taken across the life course is influenced by inherited factors and is also conditioned by developmental experiences that can enhance (e.g. through environmental enrichment and play) or diminish (e.g. through trauma, deficiency and disease) the course of the life trajectory as it unfolds (Khan et al. 2017). Many functions and processes of the animal exhibit repeated cycles on varying timescales during development, maturity and decline, including the molecular machinery of the circadian clock, the central seasonal switch, seasonal adaptations within peripheral tissues, oestrus cycle, pregnancy, lactation cycle, hair cycle, immune responses, associative learning, and so on. Together, these cycles of varying period are integrated into a whole-of-animal life trajectory (Kirkwood and Melov 2011; Promislow et al. 2022). The location of an animal along its life course is described as its biological age, which can deviate from the mean position of the group along their life courses at each calendar age, which is described as chronological age (Khan et al. 2017; Horvath and Raj 2018). The rate of life course progression (i.e. the rate of biological aging) differs among individuals (Horvath and Raj 2018; Caulton et al. 2022; Nery da Silva et al. 2022). The cumulative exposure to fluctuations in the internal and external environments, termed the exposome, causes wear and tear on structures and processes in the body (McEwen 2003). The declining phase of the life trajectory is thought to be influenced by inherent aging processes (Kirkwood and Melov 2011; Horvath and Raj 2018; Bateson and Poirier 2019; Promislow et al. 2022). Many theories of aging have been developed to account for progression along the life course. Biological age has been proposed as a common currency that integrates the quality of the whole-of-life experience of the animal, and hence has attracted attention as a cumulative indicator of whole-of-life wellbeing (Bateson and Poirier 2019; Bradshaw 2019; Keeling et al. 2021; Nery da Silva et al. 2022). Signatures of biological age and of the cumulative burden of environmental experiences are written down in DNA as telomere length and epigenetic methylation marks, in the functional capacity of physiological, behavioural and cognitive processes, and in biomarkers of aging (Jylhävä et al. 2017). Research on biological age in humans has focused on prediction of the future life course, including predicted longevity and future functional capacities. A strong motivation for this research in humans lies in prediction of future quality of life and care needs. Thus, from this viewpoint, the utility of biomarkers of aging has been defined in terms of their (1) predictive power, (2) relation to aging processes rather than disease, (3) ease of repeated use, and (4) correlation with animal models of aging (Baker and Sprott 1988; Johnson 2006). Here, the benchmark for utility of a biomarker of aging is its ability to exceed the predictive power of chronological age. This application in humans contrasts with studies on animal wellbeing where a major focus in estimation of biological age is on the retrospective indication it provides of the quality of past experiences such as the cumulative burden of stressors as the cause of faster than expected progression along the life course.
DNA methylation or epigenetic clocks
In a review of biomarkers of aging in humans it was concluded that DNA methylation had the highest level of validation and predictive ability in comparison with telomer length and composite biomarkers, including transcriptomic, proteomic, or metabolomic-based estimators (Jylhävä et al. 2017). Levels of DNA methylation reflect chronological age as well as stress-associated decline in function and are described as an ‘epigenetic clock’ (Hannum et al. 2013). These clocks can be very precise, and have the potential to provide an integrated measure of functional age at the level of the whole animal, as well as providing a measure of organ-specific and disease-specific functional age (Bell et al. 2019). An epigenetic clock calibrated against chronological age has been developed in ruminants for retrospective estimatation of the date when calves were born in extensive grazing systems (Hayes et al. 2021). Methylation changes associated with chronological age are modified by the cumulative burden of environmental stimuli termed the exposome. Calibration of methylation patterns associated with specific stressors has commenced in pigs (Hao et al. 2016; Nery da Silva et al. 2022), chickens (Pértille et al. 2020) and cattle (Del Corvo et al. 2020, 2021). In accord with other indicators of stress, both physical and psychological trauma influence DNA methylation in humans and animal models (Vinkers et al. 2015; Sales et al. 2021). Variation among stressors in their effects on methylation patterns (Vetter et al. 2022) highlights a need for further refinement of these tools for their use in the retrosepctive assessment of the quality of lived experience in livestock. Genetic variation in DNA methylation patterns in cattle has been estimated to have a heritability of 0.45 (Ribeiro et al. 2022). The heritable variation seen here could lie in the molecular machinery of methylation, as well as in susceptibility of animals to environmental conditions that modulate methylation. For commercial application as an indicator of health and longevity, an automated and cost-effective technology is required. Utility of the technology might be improved by inclusion of a genomic tool for use, in parallel with assessment of prior wellbeing for genetic selection purposes (Clarke et al. 2021). Other molecular biomarkers of aging such as non-coding RNA (ncRNA), and most predominantly microRNA (miRNA), are being explored as indicators of the cumulative burden of environmental stressors, but require further validation (Jylhävä et al. 2017; Miretti et al. 2020; Marsh et al. 2021).
Telomere length
Telomeres are protective nucleoprotein structures located at both ends of chromosomes (Blackburn 1991). Telomeric shortening occurs during various events including normal cell division and incomplete end-to-end replication and have been proposed as a biological clock and predictor of lifespan (Chatelain et al. 2020). Telomere length is not only a mitotic clock indicating chronological age but is also affected through oxidative stress and damage due to exposure to stressors (Lin and Epel 2022). Studies in various livestock species have indicated that telomere length is affected by physical and psychological stressors in a species-specific manner (Bateson 2016; Laubenthal et al. 2016; Seibt et al. 2022). It has been questioned whether telomer length per se or rate of telomere attrition is the more appropriate indicator of the cumulative burden of exposure to stressors. As well as retrospective interpretation of life experiences (Bateson 2016; Bateson and Poirier 2019; Bradshaw 2019), telomere length measured in neonatal cattle has been used as a predictor of survival to 12 months, length of productive life and future health outcomes (Brown et al. 2012; Ilska-Warner et al. 2019). Although the predictive ability of characteristics of telomere length requires further validation, the reliability of prediction and the scale of usability will depend on the development of a standard testing protocol that is easy and cost-effective to implement.
Functional capacity
Functional capacity describes performance of body systems against a benchmark of performance expected for animals of the same chronological age. The development of functional competence in sensory, motor, physiological, behavioural, immunological and cognitive systems commences in utero and continues during postnatal life until attaining a plateau during maturity and then declining with advancing age (Mellor 2019; Colditz 2022). Functional performance during these three phases of progress across the life course can vary among biological systems, which may require independent assessment to determine their individual contributions to the whole-of-life wellbeing of the animal. For the first phase, despite copious literature on developmental biology of production animals (e.g. Dwyer 2003; Sangild et al. 2013; Andersen et al. 2016; Greenwood and Bell 2019; Špinka 2019), milestones for functional development do not appear to have been consolidated into indexes such as Apgar scores (Baxter et al. 2008; Probo and Veronesi 2022) that extend beyond the first few hours of life. Rates of acquisition of periodicity (Rhodes et al. 2022) and complexity (Wu et al. 2020) in behavioural activity in the young may have roles as indicators of functional development in addition to more commonly used task-specific performances such as time to first ingestion of colostrum (Quesnel et al. 2023).
Most assessments of physiological functions and uniformity of production variables described above have focused on mature animals, although some studies have addressed uniformity of behavioural and physiological trajectories during periods of developmental transition associated with weaning (Monk et al. 2018; Revilla et al. 2019) and puberty (Neave et al. 2020). Studies on aging in humans and animal models have shown that following maturity, the rate of functional decline is not uniform but differs within and among physiological, motor, behavioural, immunological and cognitive domains (Khan et al. 2017). This limits the capacity for a single measure of functional capacity to describe the rate of aging. To address the varying rates of senescence among functions and organs, many batteries of tests and clinical scores has been developed in humans and mice (Whitehead et al. 2014). Similar functional tests such as an ability to walk 100 m without rest have been used to assess welfare in production animals (Colditz and Le Jambre 2008). In 2015, WHO issued its first World report on aging and health in humans that drew the multiple dimensions of functional capacity together in a proposed integrated indicator of healthy aging termed intrinsic capacity. This indicator encapsulates all the physical and mental reserves an individual can draw on to help deal with life’s daily challenges (Beard et al. 2016). The conceptual advance provided by the report was to move assessments of aging away from a negative focus on clinical scores of the severity of disease to a positive focus of functional capacity and the ability of the individual to access environments that enable resilience (Beard et al. 2016). Intrinsic capacity in humans has been operationalised through functional tests in four domains, namely, cognition, sensory, locomotion, psychosocial, and a fifth overarching domain termed vitality that enables expression of capacity in the first four domains (Beard et al. 2019).
For production animals, measures of functional competence based on longevity (stayability) may contribute to assessments of the rate of biological aging (e.g. Hu et al. 2021; Friggens et al. 2022; Vargovic et al. 2022). For example, a novel indicator of functional capacity during aging may be encoded in the persistence of production over successive years or parities. Units of production suitable for analysis could include 305-day milk yield, birth weight, litter size, weaning weight of offspring, weaning percentage, and annual wool yield. Additional measures of reproductive performance that vary with parity in pigs include live births, still births, and the number of runts in a litter (Vargovic et al. 2022). The premise here is the same as for telomere length and DNA methylation, namely, that accelerated decline in performance with age or parity is indicative of a greater than average burden of wear and tear and hence indicates accelerated biological aging. Genetic variation between individuals in trait trajectories across parities would need consideration in the estimation of deviations from expected age-related trajectories (Williams et al. 2022).
Biomarkers of allostatic load
The effort (ponos) of coping was recognised by the ancient Greek physician Hippocrates to accompany the suffering (pathos) caused by disease (cited by Moberg 1985). The burden of coping can lead to facultative adaptations in physiological, behavioural and immune functions through the process termed allostasis (Sterling 2012). It has been suggested that, over time, the cumulative burden of attempting to cope places an allostatic load on the animal that can contribute to a decline in functional capacity that is described as ‘wear and tear’ or ‘weathering’ (McEwen and Stellar 1993; Geronimus et al. 2006). Molecular and physiological signatures of allostatic load have therefore attracted attention as indicators of functional decline in humans (Ryff and Singer 1998). To guide clinical assessments of allostatic load in humans, several indexes have been developed that measure biomarkers in categories including cardiovascular, body form, inflammatory, energy metabolism, lipids and steroids. While the concepts of allostasis and allostatic load have been incorporated into studies of animal welfare (Korte et al. 2007; Seeley et al. 2022), and assessment of physiological and behavioural biomarkers is the cornerstone of studies on chronic stress (Ralph and Tilbrook 2016), development and validation of standardised indexes of biomarkers for assessing allostatic load in production animals remains in its infancy (Korte et al. 2007; Seeley et al. 2022). Biomarkers of allostatic load used most frequently in animals have been analytes in the hypothalamic pituitary adrenal (HPA) axis, glucose, lactate, inflammatory cytokines, acute-phase proteins, albumin and heart function variables (Seeley et al. 2022). A decline in circadian periodicity of biomarkers is also recognised as an indicator of allostatic load (Rao and Androulakis 2019). Some biomarkers of allostatic load are included in the assessment of intrinsic capacity in humans (Beard et al. 2019).
Beyond the conceptual framework of allostatic load, a wide range of molecular and behavioural biomarkers has been examined in the search for signatures of normal and pathological functioning in production animals (Moberg 1985). Molecular candidates include biomarkers of stress-response pathways, adrenal androgens, neurotransmitters from the central and peripheral nervous systems, pheromones, immune and inflammatory mediators, micro RNAs and energy metabolites (Ralph and Tilbrook 2016; de Almeida et al. 2019; Krogh et al. 2020; Miretti et al. 2020; Zachut et al. 2020). As illustrated by biomarkers of allostatic load, interpretation of biomarkers depends on the biological model underpinning the concepts of welfare and wellbeing that is used to appraise the quality of the animal’s life.
Corticosteroids have historically held a special place among biomarkers of wellbeing due to their prominence during stress (Moberg 1985; Ralph and Tilbrook 2016). The analysis of concentrations of corticosteroids in hair, feathers and eggs as an indicator of cumulative systemic stress has been comprehensively reviewed (Burnard et al. 2017; Heimbürge et al. 2019; Heimbürge et al. 2020; Ghassemi Nejad et al. 2022). In addition to the influence of systemic concentrations of corticosteroids, concentrations in hair are influenced by animal age, body site, hair colour, sex, adiposity, pregnancy, environmental contamination with urine and faeces, and UV exposure (Burnard et al. 2017; Heimbürge et al. 2020; Tamminen et al. 2021; Ghassemi Nejad et al. 2022; Otten et al. 2022). Heimbürge et al. (2020) reported on a serial analysis of cortisol in hair of cattle and pigs with a resolution of 4 weeks. An important challenge to the interpretation of hair corticosteroids is the complexity of HPA axis function in chronic stress (Mormède et al. 2007). Tamminen et al. (2021) noted both elevated and depressed concentration of hair cortisol associated with animal-based indicators of poor welfare in calves and concluded that associations between coping style and HPA axis function during stress could be a confounding factor in interpretation of hair cortisol. High-frequency serial sampling has the potential to identify response patterns that are unique to the individual animal, termed idiographic analysis (Colditz 2023), that may help with interpretation of incremental changes in hair cortisol. Most authors conclude that substantial further work is needed to establish the technical feasibility and construct validity of hair cortisol as an indicator of the cumulative lived experience of the animal.
Interpreting these indicators: relationships between functional integrity and wellbeing
The integrity of physical functioning is recognised as an important dimension of an animal’s welfare (Fraser et al. 1997; Fraser 1999; Verhoog 2000; Dawkins 2021) and wellbeing (Moberg 1985; Turner 2019; Williams 2021; Colditz 2023). Wellbeing is a tripartite construct used to describe the positive aspects of physical eudaimonic functioning, hedonic experience and social interactions that has substantial overlap with concepts in positive welfare (Sandöe et al. 2004; Williams 2021; Colditz 2023). The quality of an animal’s life is determined not only by the absence of harms but also through realisation of opportunities for positive experiences (Boissy et al. 2007; Yeates and Main 2008; FAWC 2009; Yeates 2011; Edgar et al. 2013; Mellor 2016; Vigors et al. 2021; Rowe and Mullan 2022). A strong focus in theoretical and empirical studies on positive welfare and wellbeing has been on developing metrics and methods for assessing the prevalence of pleasant hedonic experiences in the animal’s life (Lawrence et al. 2019; Webb et al. 2019; Keeling et al. 2021). Nonetheless, the potential for positive physical functions to contribute to the quality of the animal’s life is also recognised (Turner 2019; Rault et al. 2020; Vigors et al. 2021; Williams 2021; Colditz 2023). Measures of the integrity of biological functioning across the whole life of the animal may therefore be able to contribute to assessment of the quality of an animal’s life and its lifetime wellbeing.
Functional integrity has some overlap with the concept of phenotypic fitness or adaptedness (Barker 2009). From the perspective of animal wellbeing, functional integrity draws attention to the success of day-to-day regulatory processes in accommodating short-term fluctuations in the environment. The degree of success in maintaining homeostasis has also been termed regulatory performance (Husak et al. 2009) and is theorised to improve fidelity of internal communication and the efficiency of metabolic performance (Woods and Wilson 2013). The internal environment is maintained in a dynamic state through reactive homeostatic reflexes and through anticipatory allostatic signals from neural and somatic tissues that combine to continually amend and prepare the animal for imminent environmental changes (Korte et al. 2007; Schulkin and Sterling 2019; Deans 2021). The dynamic homeostatic outcome that arises through these processes occurs within the context of tissue settings that are tuned by homeorhetic signalling in accord with genetic drives (Bauman 2000; Friggens and Newbold 2007; Baumgard et al. 2017). Tissue settings can also be tuned in the face of persistent environmental loads to enable adaptive plasticity (Romero et al. 2009; Koolhaas et al. 2011). The longer-term changes in the level of performance (e.g. milk yield) due to homeorhesis and adaptive plasticity are indicators of phenotypic robustness and also contribute to phenotypic fitness and adaptedness (Friggens and Newbold 2007). The close coupling of behaviours and physiological regulation with circadian dynamics in the external environment lend support to the focus on homeostatic performance as an indicator of functional integrity. From this viewpoint, serial outcomes of short-term homeostatic performance laid down in production tissues, structural tissues, epigenetic marks, and telomere length have the potential to provide cumulative indicators of wellbeing, as previously concluded by others (Bateson 2016; Bateson and Poirier 2019; Bradshaw 2019; Caulton et al. 2022).
As noted in the introductory section on the physiological context of functional integrity, the physiological activities of cells and organs contribute to the animal’s affective state. Thus, a continuous dialogue occurs between conditions in the body and affective state. Metabolites and messengers generated by metabolic and immune functions and the gastrointestinal tract pass to the central nervous system via sensory nerves and blood. These signals constitute the sensory system called interoception ((Bud) Craig 2003). Central processing integrates these interoceptive signals with sensations and perceptions from the external environment to generate affective state. In a reciprocal fashion, affective state continuously modulates metabolic, immune and organ functions via autonomic and neuroendocrine signals. Thus, homeostatic activities occurring at the levels of cells and organs in peripheral tissues contribute to affective state as a whole-of-body condition (Fig. 2). Despite these interdependencies between function and affect, further validation is required to establish the extent to which functional integrity provides an indicator of the quality of the lived experience.
Two applications of indicators of functional integrity are retrospective assessment and real-time monitoring. Retrospective analyses of sensor data and records archived in tissues can contribute to accreditation of the functional dimension of the quality of lived experience, whereas real-time analyses of sensor data can support animal management and continuous assessment of welfare and wellbeing, as well as be accumulated over time to give a whole-of-life summary. As noted above, monitoring behavioural complexity and affect dynamics may also contribute to assessment of social and hedonic dimensions of wellbeing. An important issue for application of real-time monitoring to animal management is the need for thresholds as triggers for intervention (Sun et al. 2021; Wagner et al. 2021; Fuchs et al. 2022). Continuous monitoring of individuals creates the possibility of establishing individualised thresholds that align with persistent differences among individuals in physiological, behavioural and affective characteristics associated with personality. While individualised thresholds may be feasible, it would, nonetheless, be highly desirable to have universal thresholds that do not require alignment with persistent individual differences. Studies by Nunes Marsiglio Sarout et al. (2018), Wagner et al. (2021) and Fuchs et al. (2022) showed some promise for developing general (universal) thresholds for describing deviations in circadian functions.
Profile analysis
Analysis of integrity via the strength of biorhythms, complexity of networks and uniformity of trajectories draws on concepts of stability in complex dynamic systems in resilience theory (Scheffer et al. 2009; Scheffer et al. 2018; van Dixhoorn et al. 2018). Deviations from trajectories (hereafter profile analysis) is particularly relevant to assessment of incremental patterns of tissue growth and production. The shape of trajectories expressed during development, during productive maturity and as performance declines with age is influenced by inherited and developmentally acquired factors and by contemporary environmental conditions such as feed availability. Profile analysis requires a reference trajectory as the basis for estimation of deviations (Doekes et al. 2022). Reference trajectories have been modelled on both individual animal data and group means and is a topic requiring further refinement. In the absence of high-frequency (e.g. daily) records, lower sampling intervals may have utility for establishing the reference trajectory (Mattalia et al. 2022). The trajectory of performance over successive years or parities may also be suitable for profile analyses in studies on longevity as an indicator of functional integrity. In the absence of high-frequency animal-based measurements, there may be a role for estimation of reference curves by genomic prediction (Alexandre et al. 2021). A variety of descriptors of deviation from the reference trajectory have been explored as indicators of functional integrity and resilience (e.g. Berghof et al. 2019a; Revilla et al. 2019; Adriaens et al. 2020; Poppe et al. 2020; Doekes et al. 2022). Informative measures of variability are likely to differ among the species, production systems, data sources and the biological functions being analysed.
Given the fine resolution of incremental change in isotope accumulation and growth morphology in tooth, hoof, and fibre, these materials may contain a historical time series that is suitable for profile analysis as indicators of the uniformity of growth trajectories (Smolyar and Bromage 2004). Important information about environmental conditions experienced by the contemporary group that is contained in the absolute level of growth trajectories may complement assessments of deviations from profiles. Incremental patterning of growth in otoliths and placoid scales in fish and in the rhinotheca in birds may also be suitable for profile analysis.
Absolute versus relative indicators of functional integrity and functional wellbeing
The environment can compromise functional integrity in many ways, such as by imposing stressors that overburden homeostatic and allostatic responses. Conversely, an animal should be able to attain functional integrity and wellbeing in a diversity of environments that may be suboptimal for maximum production. Indeed, despite a trend towards reducing variation among environments experienced by production animals through intensification and standardisation of management practices, such as by housing animals indoors and feeding total mixed rations, variation remains among the environmental conditions experienced by management groups. For example, dairy cows are sensitive to differences among milking-parlour workers in their attitudes towards animals (Maller et al. 2005). In evaluations of the genetic merit for performance traits such as growth rate and daily milk yield, the influence of local environmental effects on expressed phenotype is addressed through genetic linkages among individuals in various management groups. Hence, genetic merit describes the relative value of the individual via its ranking against other animals through analyses that accommodate differences in absolute performance among environments.
If functional integrity and wellbeing of an individual can be optimised in a diversity of environments, then animal-based metrics need to provide absolute values that are directly comparable among management groups without a need for correction for local environmental effects. For example, growth rate performance is assessed by comparison to other animals within a management group. In a genetic evaluation, progeny from a top-ranked sire may have average daily growth of 1.1 kg/day in one environment and 1.6 kg/day in a second environment. In contrast, periodicity has a maximum score of 1, indicating complete synchronicity, and uniformity assessed via deviations can have a minimum score of 0, indicating complete uniformity. For an evaluation of functional integrity, the animal topping one contemporary group with a score of circadian periodicity of say 0.85 would not be equivalent in terms of wellbeing to the animal topping a second contemporary group with a score of 0.95. Thus, integrity and wellbeing as descriptions of an absolute state of the animal, need, at least ideally, to be assessed by measurements scored on an absolute rather than a relative scale.
Periodicity, complexity and uniformity have promise here as absolute measures, as their metrics can be based on invariant scales that translate among environments. Nonetheless, a portion of the variation between individuals in functional integrity is likely to be due to inherited factors, as is seen in studies on resilience (reviewed by Berghof et al. 2019a). This suggests that a capacity to attain absolute functional integrity may differ among individuals. Similarly, a propensity for individuals to experience positive hedonic states is influenced by temperament (Boissy and Erhard 2014). This raises the dilemma whether individuals with an inherited predisposition to poorer functional integrity, poorer functional wellbeing and less frequent experience of positive hedonic states, irrespective of the environment they experience, should be penalised in welfare assessments and breeding programs.
As a step towards resolving this dilemma, further understanding is needed of the role that a diversity among individuals in functional integrity and hedonic disposition plays in the social ecology of the group (Moreno García et al. 2020; Moreno García et al. 2022). Is it advantageous for the group for some individuals to ‘take one for the team’? For example, more fearful individuals may serve a benefit to the group through heightened awareness of the presence of predators at the personal cost of lower reproductive performance, slower growth, more variable daily performance and more frequent experience of negative affective states (Colditz 2021). To cast this in more positive terms, each individual carves out a dynamic affective niche in the social landscape (Colombetti and Krueger 2015). Borrowing from Cannon (1939) who described homeostasis as the ‘wisdom of the body’, we can say that the ‘wisdom of the group’ assembles an affective social landscape (Colditz 2020; Nagatsu and Salmela 2022) echoing the ‘wisdom of the cell’ that enables self-assembly of complex structures during embryogenesis and tissue repair (Shreesha and Levin 2023). And as social dominance shows, not all affective niches are equal (Krahn et al. 2023). As a consequence, social ecological factors may induce variations among individuals in functional wellbeing irrespective of genetic factors, as seen for behavioural diversity among genetically identical mice housed in a shared environment (Freund et al. 2013). Such social limits to individual functioning and wellbeing do not diminish our responsibility to provide animals with the opportunity to realise their potential for positive affective experiences (Mellor 2015).
Subjectivity of environmental sensibility
When animals are housed in a shared environment such as a cow barn, grower pen or mouse cage, many of the daily variations in individual performance trajectories are not synchronised within the group. This variation among animals draws attention to the subjectivity of the individual’s experience (Webster 2013) and has led to the concept that animals sharing a common space also inhabit a private non-shared environment (Freund et al. 2013). Day-to-day fluctuations in the animal’s non-shared environment have been termed micro-stressors (DiCorcia and Tronick 2011) and are not necessarily captured by objective descriptions of environmental variables such as climate, feed practices, and other management practices implemented at the group level (Garcia-Baccino et al. 2021; Poppe et al. 2021). Both types of environmental conditions can influence homeostatic integrity. The subjectivity of environmental perception can be captured in the aphorism ‘Stress, like beauty, is in the eye of the beholder’ and lends support to the proposition that functional integrity is linked with the quality of subjective experience. Nonetheless, substantial further investigation is required to establish the relationships between subjective experience and functional integrity as indicators of wellbeing (Vigors et al. 2021; Williams 2021; Colditz 2023).
Other potential measures of functional integrity
Biological records archived in structures and functions are unlikely to provide a complete account of the lived experience of the animal and could be complemented by clerical records of husbandry practices, animal medications, disease events and environmental conditions in accord with commonly used protocols for welfare assessment. Clinical assessments of the animal at slaughter (Llonch et al. 2015; Grandin 2017; Bautista-Fernández et al. 2021) may be an important adjunct to biological and clerical records for assessing cumulative wellbeing.
Combining indicators of functional integrity
The changes in biological functions that occur across the life-course suggest that a single indicator is unlikely to be informative of functional status at each stage; hence, a combination of indicators may be needed to assess functional wellbeing. In particular, different indictors may be needed to assess functional competences during development, homeostatic integrity during maturity, and functional decline due to inherent aging processes and wear and tear. A substantial amount of work will be needed to refine measurement technologies and combine data streams so as to provide an integrated description of lifetime functional integrity and wellbeing. The finest resolution of functional integrity is likely to be provided by high-frequency sensor data on physiological and behavioural functions, whereas records archived in physical structures may be able to provide a coarser-grained summary of wellbeing. The temporal sequence of deviations embedded in profiles of behaviour, physiology, and accretion of resources in products and stable structures may be able to be collated with clerical records and meteorological information to enrich the interpretation of prior experiences. In contrast, the cumulative summary encoded in telomere length and DNA methylation lacks this temporal nuance. Processing cost for lab analyses may limit feasibility of performing some types of analyses on every animal. Combining indicators of functional integrity with indictors of hedonic and social wellbeing remains an important goal for describing the quality of lived experience across the whole of the animal’s life.
This review has addressed approaches to assessing the totality of wellbeing across the whole of an animal’s life. The observation that changes in methylation patterns can vary with different types of stressors points to a second application, namely, the development of diagnostic signatures for quantifying the impact of key events in the animal’s life that are recognised as prominent causes of compromised welfare. These events include husbandry practices such as a marking, weaning, social mixing, transport, and changes in management environments (e.g. feedlot entry), as well as life-history transitions such as parturition and the cessation of lactation at drying off.
Conclusions
The influence of physiological regulatory performance on the integrity of biological functions provides a window on the success of the animal in coping with day-to-day fluctuations in its environment. Changes in the circadian harmony, complexity of behaviours and the day-to-day uniformity of production outcomes and in incremental growth in structural components of the body may provide indicators of functional wellbeing. Real-time monitoring of these outcomes by sensor technologies can be complemented by records archived in stable structures of the body and as epigenetic marks and telomere length. Homeostatic performance as an indicator of wellbeing draws attention to the success of the animal in navigating the day-to-day course of its life trajectory. Further work is required to validate measures such of incremental changes in fibre diameter, and in growth patterns of hoof, scale, otolith and beak as indicators of homeostatic integrity, and to establish the reliability and feasibility for deploying these measures in commercial environments. Complexities of sample preparation and wet laboratory analysis may limit some indicators from application in production animals beyond research studies. Informative indicators of wellbeing are likely to differ among the species, production systems, environments, biological functions, and life stages being assessed. There is an opportunity for indexes of developmental milestones to be developed as benchmarks for assessment of developmental wellbeing. Important information about environmental conditions that is contained in the level of performance trajectories may complement assessment of deviations from profiles as indicators of functional integrity. Time-series records have greater potential for collation with the temporal sequence of events in the external environment than do cumulative indicators of wear and tear such as telomere length and DNA methylation. Further construct validation is required to establish the relationships among functional, hedonic and social indicators of wellbeing.
Data availability
Data sharing is not applicable as no new data were generated or analysed during this study.
Declaration of funding
The project was supported by funding from Meat & Livestock Australia grant B.AWW.0009. Australian Commonwealth Government contributions are acknowledged through the support of the Research Training Program stipend and Destination Australia Program scholarships, which E. G. Smith has received.
Acknowledgements
We thank Jessica Monk, Hannah Salvin and an anonymous reviewer for valuable comments on a draft of this paper. HS drew to our attention the possibility of using some of these indicators of functional integrity to develop diagnostic signatures for assessing the impact of key husbandry practices on welfare.
References
Adams NR, Briegel JR (1998) Liveweight and wool growth responses to a Mediterranean environment in three strains of Merino sheep. Australian Journal of Agricultural Research 49, 1187-1194.
| Crossref | Google Scholar |
Adriaens I, Friggens NC, Ouweltjes W, Scott H, Aernouts B, Statham J (2020) Productive life span and resilience rank can be predicted from on-farm first-parity sensor time series but not using a common equation across farms. Journal of Dairy Science 103, 7155-7171.
| Crossref | Google Scholar |
Alexandre PA, Li Y, Hine BC, Duff CJ, Ingham AB, Porto-Neto LR, Reverter A (2021) Correction to: Bias, dispersion, and accuracy of genomic predictions for feedlot and carcase traits in Australian Angus steers. Genetics Selection Evolution 53, 88.
| Crossref | Google Scholar |
Andersen AD, Sangild PT, Munch SL, van der Beek EM, Renes IB, Ginneken Cv, Greisen GO, Thymann T (2016) Delayed growth, motor function and learning in preterm pigs during early postnatal life. American Journal of Physiology-Regulatory, Integrative and Comparative Physiology 310, R481-R492.
| Crossref | Google Scholar |
Asher L, Collins LM, Ortiz-Pelaez A, Drewe JA, Nicol CJ, Pfeiffer DU (2009) Recent advances in the analysis of behavioural organization and interpretation as indicators of animal welfare. Journal of The Royal Society Interface 6, 1103-1119.
| Crossref | Google Scholar |
Baker GT, III, Sprott RL (1988) Biomarkers of aging. Experimental Gerontology 23, 223-239.
| Crossref | Google Scholar |
Balasse M, Obein G, Ughetto-Monfrin J, Mainland I (2012) Investigating seasonality and season of birth in past herds: a reference set of sheep enamel stable oxygen isotope ratios. Archaeometry 54, 349-368.
| Crossref | Google Scholar |
Basile F, Capaccia C, Zampini D, Biagetti T, Diverio S, Guelfi G (2021) Omics insights into animal resilience and stress factors. Animals 11, 47.
| Crossref | Google Scholar |
Bateson M (2016) Cumulative stress in research animals: telomere attrition as a biomarker in a welfare context? BioEssays 38, 201-212.
| Crossref | Google Scholar |
Bateson M, Poirier C (2019) Can biomarkers of biological age be used to assess cumulative lifetime experience? Animal Welfare 28, 41-56.
| Crossref | Google Scholar |
Baumgard LH, Collier RJ, Bauman DE (2017) A 100-year review: regulation of nutrient partitioning to support lactation. Journal of Dairy Science 100, 10353-10366.
| Crossref | Google Scholar |
Bautista-Fernández M, Estévez-Moreno LX, Losada-Espinosa N, Villarroel M, María GA, De Blas I, Miranda-de La Lama GC (2021) Claw disorders as iceberg indicators of cattle welfare: evidence-based on production system, severity, and associations with final muscle pH. Meat Science 177, 108496.
| Crossref | Google Scholar |
Baxter BP, Brims MA, Taylor TB (1992) Description and performance of the optical fibre diameter analyser (OFDA). Journal of the Textile Institute 83, 507-526.
| Crossref | Google Scholar |
Baxter EM, Jarvis S, D’Eath RB, Ross DW, Robson SK, Farish M, Nevison IM, Lawrence AB, Edwards SA (2008) Investigating the behavioural and physiological indicators of neonatal survival in pigs. Theriogenology 69, 773-783.
| Crossref | Google Scholar |
Beard JR, Officer A, de Carvalho IA, Sadana R, Pot AM, Michel J-P, Lloyd-Sherlock P, Epping-Jordan JE, Peeters GMEE, Mahanani WR, Thiyagarajan JA, Chatterji S (2016) The World report on ageing and health: a policy framework for healthy ageing. The Lancet 387, 2145-2154.
| Crossref | Google Scholar |
Beard JR, Jotheeswaran AT, Cesari M, Araujo de Carvalho I (2019) The structure and predictive value of intrinsic capacity in a longitudinal study of ageing. BMJ Open 9, e026119.
| Crossref | Google Scholar |
Bedere N, Berghof TVL, Peeters K, Pinard-van der Laan M-H, Visscher J, David I, Mulder HA (2022) Using egg production longitudinal recording to study the genetic background of resilience in purebred and crossbred laying hens. Genetics Selection Evolution 54, 26.
| Crossref | Google Scholar |
Bell AW, Bauman DE (1997) Adaptations of glucose metabolism during pregnancy and lactation. Journal of Mammary Gland Biology and Neoplasia 2, 265-278.
| Crossref | Google Scholar |
Bell CG, Lowe R, Adams PD, Baccarelli AA, Beck S, Bell JT, Christensen BC, Gladyshev VN, Heijmans BT, Horvath S, Ideker T, Issa J-PJ, Kelsey KT, Marioni RE, Reik W, Relton CL, Schalkwyk LC, Teschendorff AE, Wagner W, Zhang K, Rakyan VK (2019) DNA methylation aging clocks: challenges and recommendations. Genome Biology 20, 249.
| Crossref | Google Scholar |
Ben Abdelkrim A, Tribout T, Martin O, Boichard D, Ducrocq V, Friggens NC (2021) Exploring simultaneous perturbation profiles in milk yield and body weight reveals a diversity of animal responses and new opportunities to identify resilience proxies. Journal of Dairy Science 104, 459-470.
| Crossref | Google Scholar |
Berghof TVL, Poppe M, Mulder HA (2019a) Opportunities to improve resilience in animal breeding programs. Frontiers in Genetics 9, 692.
| Crossref | Google Scholar |
Berghof TVL, Bovenhuis H, Mulder HA (2019b) Body weight deviations as indicator for resilience in layer chickens. Frontiers in Genetics 10, 1216.
| Crossref | Google Scholar |
Blackburn EH (1991) Structure and function of telomeres. Nature 350, 569-573.
| Crossref | Google Scholar |
Boissy A, Manteuffel G, Jensen MB, Moe RO, Spruijt B, Keeling LJ, Winckler C, Forkman B, Dimitrov I, Langbein J, Bakken M, Veissier I, Aubert A (2007) Assessment of positive emotions in animals to improve their welfare. Physiology & Behavior 92, 375-397.
| Crossref | Google Scholar |
Bradshaw WJ (2019) Assessing biomarkers of ageing as measures of cumulative animal welfare. Available at https://ecoevorxiv.org/repository/view/4407/
Broom DM (1996) Animal welfare defined in terms of attempts to cope with the environment. Acta Agriculturae Scandinavica, Section A. Animal Science Supplement 27, 22-28.
| Google Scholar |
Brown DJ, Crook BJ, Purvis IW (2002) Differences in fibre diameter profile characteristics in wool staples from Merino sheep and their relationship with staple strength between years, environments, and bloodlines. Australian Journal of Agricultural Research 53, 481-491.
| Crossref | Google Scholar |
Brown DE, Dechow CD, Liu WS, Harvatine KJ, Ott TL (2012) Hot topic: association of telomere length with age, herd, and culling in lactating Holsteins. Journal of Dairy Science 95, 6384-6387.
| Crossref | Google Scholar |
(Bud) Craig AD (2003) Interoception: the sense of the physiological condition of the body. Current Opinion in Neurobiology 13, 500-505.
| Crossref | Google Scholar |
Burgunder J, Petrželková KJ, Modrý D, Kato A, MacIntosh AJJ (2018) Fractal measures in activity patterns: do gastrointestinal parasites affect the complexity of sheep behaviour? Applied Animal Behaviour Science 205, 44-53.
| Crossref | Google Scholar |
Burnard C, Ralph C, Hynd P, Hocking Edwards J, Tilbrook A (2017) Hair cortisol and its potential value as a physiological measure of stress response in human and non-human animals. Animal Production Science 57, 401-414.
| Crossref | Google Scholar |
Careau V, Thomas D, Humphries MM, Réale D (2008) Energy metabolism and animal personality. Oikos 117, 641-653.
| Crossref | Google Scholar |
Casey TM, Plaut K (2012) Lactation biology symposium: circadian clocks as mediators of the homeorhetic response to lactation. Journal of Animal Science 90, 744-754.
| Crossref | Google Scholar |
Casey TM, Plaut K (2022) Circadian clocks and their integration with metabolic and reproductive systems: our current understanding and its application to the management of dairy cows. Journal of Animal Science 100, skac233.
| Crossref | Google Scholar |
Casey TM, Plaut K, Boerman J (2022) Circadian clocks and their role in lactation competence. Domestic Animal Endocrinology 78, 106680.
| Crossref | Google Scholar |
Caulton A, Dodds KG, McRae KM, Couldrey C, Horvath S, Clarke SM (2022) Development of epigenetic clocks for key ruminant species. Genes 13, 96.
| Crossref | Google Scholar |
Chatelain M, Drobniak SM, Szulkin M (2020) The association between stressors and telomeres in non-human vertebrates: a meta-analysis. Ecology Letters 23, 381-398.
| Crossref | Google Scholar |
Clarke S, Caulton A, McRae K, Brauning R, Couldrey C, Dodds K (2021) Beyond the genome: a perspective on the use of DNA methylation profiles as a tool for the livestock industry. Animal Frontiers 11, 90-94.
| Crossref | Google Scholar |
Colditz IG (2002) Effects of the immune system on metabolism: implications for production and disease resistance in livestock. Livestock Production Science 75, 257-268.
| Crossref | Google Scholar |
Colditz IG (2018) Objecthood, agency and mutualism in valenced farm animal environments. Animals 8, 50.
| Crossref | Google Scholar |
Colditz IG (2020) A consideration of physiological regulation from the perspective of Bayesian enactivism. Physiology & Behavior 214, 112758.
| Crossref | Google Scholar |
Colditz IG (2021) Adrenergic tone as an intermediary in the temperament syndrome associated with flight speed in beef cattle. Frontiers in Animal Science 2, 652306.
| Crossref | Google Scholar |
Colditz IG (2022) Competence to thrive: resilience as an indicator of positive health and positive welfare in animals. Animal Production Science 62, 1439-1458.
| Crossref | Google Scholar |
Colditz IG (2023) A biological integrity framework for describing animal welfare and wellbeing. Animal Production Science 63, 423-440.
| Crossref | Google Scholar |
Colditz IG, Hine BC (2016) Resilience in farm animals: biology, management, breeding and implications for animal welfare. Animal Production Science 56, 1961-1983.
| Crossref | Google Scholar |
Colditz IG, Le Jambre LF (2008) Development of a faecal occult blood test to determine the severity of Haemonchus contortus infections in sheep. Veterinary Parasitology 153, 93-99.
| Crossref | Google Scholar |
Colombetti G, Krueger J (2015) Scaffoldings of the affective mind. Philosophical Psychology 28, 1157-1176.
| Crossref | Google Scholar |
de Almeida AM, Zachut M, Hernández-Castellano LE, Šperanda M, Gabai G, Mobasheri A (2019) Biomarkers of fitness and welfare in dairy animals: healthy living. Journal of Dairy Research 86, 379-387.
| Crossref | Google Scholar |
Deans C (2021) Biological prescience: the role of anticipation in organismal processes. Frontiers in Physiology 12, 672457.
| Crossref | Google Scholar |
Del Corvo M, Bongiorni S, Stefanon B, Sgorlon S, Valentini A, Ajmone Marsan P, Chillemi G (2020) Genome-wide DNA methylation and gene expression profiles in cows subjected to different stress level as assessed by cortisol in milk. Genes 11, 850.
| Crossref | Google Scholar |
Del Corvo M, Lazzari B, Capra E, Zavarez L, Milanesi M, Utsunomiya YT, Utsunomiya ATH, Stella A, de Paula Nogueira G, Garcia JF, Ajmone-Marsan P (2021) Methylome patterns of cattle adaptation to heat stress. Frontiers in Genetics 12, 633132.
| Crossref | Google Scholar |
DiCorcia JA, Tronick E (2011) Quotidian resilience: exploring mechanisms that drive resilience from a perspective of everyday stress and coping. Neuroscience & Biobehavioral Reviews 35, 1593-1602.
| Crossref | Google Scholar |
Doekes HP, Bovenhuis H, Berghof TVL, Peeters K, Visscher J, Mulder HA (2022) Research note: genome-wide association study for natural antibodies and resilience in a purebred layer chicken line. Poultry Science 102, 102312.
| Crossref | Google Scholar |
Dwyer CM (2003) Behavioural development in the neonatal lamb: effect of maternal and birth-related factors. Theriogenology 59, 1027-1050.
| Crossref | Google Scholar |
Edgar JL, Mullan SM, Pritchard JC, McFarlane UJC, Main DCJ (2013) Towards a ‘good life’ for farm animals: development of a resource tier framework to achieve positive welfare for laying hens. Animals 3, 584-605.
| Crossref | Google Scholar |
Elgersma GG, De Jong G, Van der Linde R, Mulder HA (2018) Fluctuations in milk yield are heritable and can be used as a resilience indicator to breed healthy cows. Journal of Dairy Science 101, 1240-1250.
| Crossref | Google Scholar |
Elsasser TH, Kahl S, Steele NC, Rumsey TS (1997) Nutritional modulation of somatotropic axis-cytokine relationships in cattle: a brief review. Comparative Biochemistry and Physiology Part A: Physiology 116, 209-221.
| Crossref | Google Scholar |
FAWC (2009) Farm animal welfare in Great Britain: past, present and future. Farm Animal Welfare Council. Available at https://www.gov.uk/government/publications/fawc-report-on-farm-animal-welfare-in-great-britain-past-present-and-future [Accessed 20 May 2022]
Fraser D (1999) Animal ethics and animal welfare science: bridging the two cultures. Applied Animal Behaviour Science 65, 171-189.
| Crossref | Google Scholar |
Fraser D, Duncan IJH (1998) ‘Pleasures’, ‘pains’ and animal welfare: toward a natural history of affect. Animal Welfare 7, 383-396.
| Crossref | Google Scholar |
Fraser D, Weary DM, Pajor EA, Milligan BN (1997) A scientific conception of animal welfare that reflects ethical concerns. Animal Welfare 6, 187-205.
| Crossref | Google Scholar |
Freund J, Brandmaier AM, Lewejohann L, Kirste I, Kritzler M, Krüger A, Sachser N, Lindenberger U, Kempermann G (2013) Emergence of individuality in genetically identical mice. Science 340, 756-759.
| Crossref | Google Scholar |
Friggens NC, Newbold JR (2007) Towards a biological basis for predicting nutrient partitioning: the dairy cow as an example. Animal 1, 87-97.
| Crossref | Google Scholar |
Friggens NC, Blanc F, Berry DP, Puillet L (2017) Review: deciphering animal robustness. A synthesis to facilitate its use in livestock breeding and management. Animal 11, 2237-2251.
| Crossref | Google Scholar |
Friggens NC, Adriaens I, Boré R, Cozzi G, Jurquet J, Kamphuis C, Leiber F, Lora I, Sakowski T, Statham J, De Haas Y (2022) Resilience: reference measures based on longer-term consequences are needed to unlock the potential of precision livestock farming technologies for quantifying this trait. Peer Community Journal 2, e38.
| Crossref | Google Scholar |
Fuchs P, Adrion F, Shafiullah AZM, Bruckmaier RM, Umstätter C (2022) Detecting ultra- and circadian activity rhythms of dairy cows in automatic milking systems using the degree of functional coupling – a pilot study. Frontiers in Animal Science 3, 839906.
| Crossref | Google Scholar |
Garcia-Baccino CA, Marie-Etancelin C, Tortereau F, Marcon D, Weisbecker J-L, Legarra A (2021) Detection of unrecorded environmental challenges in high-frequency recorded traits, and genetic determinism of resilience to challenge, with an application on feed intake in lambs. Genetics Selection Evolution 53, 4.
| Crossref | Google Scholar |
Geronimus AT, Hicken M, Keene D, Bound J (2006) ‘Weathering’ and age patterns of allostatic load scores among blacks and whites in the United States. American Journal of Public Health 96, 826-833.
| Crossref | Google Scholar |
Ghassemi Nejad J, Ghaffari MH, Ataallahi M, Jo J-H, Lee H-G (2022) Stress concepts and applications in various matrices with a focus on hair cortisol and analytical methods. Animals 12, 3096.
| Crossref | Google Scholar |
Gloag C, Behrendt R (2002) The influence of measurement interval and grease on OFDA2000 profile characteristics. Wool Technology and Sheep Breeding 50, 805-811.
| Google Scholar |
Goh GH, Maloney SK, Mark PJ, Blache D (2019) Episodic ultradian events – ultradian rhythms. Biology 8, 15.
| Crossref | Google Scholar |
Gonzalez EB, Sacchero DM, Easdale MH (2020) Environmental influence on Merino sheep wool quality through the lens of seasonal variations in fibre diameter. Journal of Arid Environments 181, 104248.
| Crossref | Google Scholar |
Goodman AH, Rose JC (1990) Assessment of systemic physiological perturbations from dental enamel hypoplasias and associated histological structures. American Journal of Physical Anthropology 33, 59-110.
| Crossref | Google Scholar |
Grandin T (2017) On-farm conditions that compromise animal welfare that can be monitored at the slaughter plant. Meat Science 132, 52-58.
| Crossref | Google Scholar |
Greenough PR (2001) Sand cracks, horizontal fissures, and other conditions affecting the wall of the bovine claw. Veterinary Clinics of North America: Food Animal Practice 17, 93-110.
| Google Scholar |
Greenwood PL, Bell AW (2019) Developmental programming and growth of livestock tissues for meat production. Veterinary Clinics of North America: Food Animal Practice 35, 303-319.
| Crossref | Google Scholar |
Grønkjær P (2016) Otoliths as individual indicators: a reappraisal of the link between fish physiology and otolith characteristics. Marine and Freshwater Research 67, 881-888.
| Crossref | Google Scholar |
Hahn MV, McDaniel BT, Wilk JC (1986) Rates of hoof growth and wear in Holstein cattle. Journal of Dairy Science 69, 2148-2156.
| Crossref | Google Scholar |
Hannum G, Guinney J, Zhao L, Zhang L, Hughes G, Sadda SV, Klotzle B, Bibikova M, Fan J-B, Gao Y, Deconde R, Chen M, Rajapakse I, Friend S, Ideker T, Zhang K (2013) Genome-wide methylation profiles reveal quantitative views of human aging rates. Molecular Cell 49, 359-367.
| Crossref | Google Scholar |
Hao Y, Cui Y, Gu X (2016) Genome-wide DNA methylation profiles changes associated with constant heat stress in pigs as measured by bisulfite sequencing. Scientific Reports 6, 27507.
| Crossref | Google Scholar |
Hayes BJ, Nguyen LT, Forutan M, Engle BN, Lamb HJ, Copley JP, Randhawa IAS, Ross EM (2021) An epigenetic aging clock for cattle using portable sequencing technology. Frontiers in Genetics 12, 760450.
| Crossref | Google Scholar |
Heimbürge S, Kanitz E, Otten W (2019) The use of hair cortisol for the assessment of stress in animals. General and Comparative Endocrinology 270, 10-17.
| Crossref | Google Scholar |
Heimbürge S, Kanitz E, Tuchscherer A, Otten W (2020) Is it getting in the hair? Cortisol concentrations in native, regrown and segmented hairs of cattle and pigs after repeated ACTH administrations. General and Comparative Endocrinology 295, 113534.
| Crossref | Google Scholar |
Herborn KA, McElligott AG, Mitchell MA, Sandilands V, Bradshaw B, Asher L (2020) Spectral entropy of early-life distress calls as an iceberg indicator of chicken welfare. Journal of The Royal Society Interface 17, 20200086.
| Crossref | Google Scholar |
Herd RM, Arthur PF (2009) Physiological basis for residual feed intake. Journal of Animal Science 87, E64-E71.
| Crossref | Google Scholar |
Horvath S, Raj K (2018) DNA methylation-based biomarkers and the epigenetic clock theory of ageing. Nature Reviews Genetics 19, 371-384.
| Crossref | Google Scholar |
Hu H, Mu T, Ma Y, Wang XP, Ma Y (2021) Analysis of longevity traits in Holstein cattle: a review. Frontiers in Genetics 12, 695543.
| Crossref | Google Scholar |
Husak JF, Irschick DJ, McCormick SD, Moore IT (2009) Hormonal regulation of whole-animal performance: implications for selection. Integrative and Comparative Biology 49, 349-353.
| Crossref | Google Scholar |
Ilska-Warner JJ, Psifidi A, Seeker LA, Wilbourn RV, Underwood SL, Fairlie J, Whitelaw B, Nussey DH, Coffey MP, Banos G (2019) The genetic architecture of bovine telomere length in early life and association with animal fitness. Frontiers in Genetics 10, 1048.
| Crossref | Google Scholar |
Jensen P, Toates FM (1997) Stress as a state of motivational systems. Applied Animal Behaviour Science 53, 145-156.
| Crossref | Google Scholar |
Johnson TE (2006) Recent results: biomarkers of aging. Experimental Gerontology 41, 1243-1246.
| Crossref | Google Scholar |
Jylhävä J, Pedersen NL, Hägg S (2017) Biological age predictors. EBioMedicine 21, 29-36.
| Crossref | Google Scholar |
Kalafatakis K, Russell GM, Ferguson SG, Grabski M, Harmer CJ, Munafò MR, Marchant N, Wilson A, Brooks JC, Thakrar J, Murphy P, Thai NJ, Lightman SL (2021) Glucocorticoid ultradian rhythmicity differentially regulates mood and resting state networks in the human brain: a randomised controlled clinical trial. Psychoneuroendocrinology 124, 105096.
| Crossref | Google Scholar |
Keeling LJ, Winckler C, Hintze S, Forkman B (2021) Towards a positive welfare protocol for cattle: a critical review of indicators and suggestion of how we might proceed. Frontiers in Animal Science 2, 753080.
| Crossref | Google Scholar |
Khan SS, Singer BD, Vaughan DE (2017) Molecular and physiological manifestations and measurement of aging in humans. Aging Cell 16, 624-633.
| Crossref | Google Scholar |
Kirkwood TBL, Melov S (2011) On the programmed/non-programmed nature of ageing within the life history. Current Biology 21, R701-R707.
| Crossref | Google Scholar |
Koolhaas JM, Bartolomucci A, Buwalda B, de Boer SF, Fluegge G, Korte SM, Meerlo P, Murison R, Olivier B, Palanza P, Richter-Levin G, Sgoifo A, Steimer T, Stiedl O, van Dijk G, Wöhr M, Fuchs E (2011) Stress revisited: a critical evaluation of the stress concept. Neuroscience & Biobehavioral Reviews 35, 1291-1301.
| Crossref | Google Scholar |
Korte SM, Olivier B, Koolhaas JM (2007) A new animal welfare concept based on allostasis. Physiology & Behavior 92, 422-428.
| Crossref | Google Scholar |
Krahn J, Foris B, Weary DM, von Keyserlingk MAG (2023) Invited review: social dominance in dairy cattle: a critical review with guidelines for future research. Journal of Dairy Science 106, 1489-1501.
| Crossref | Google Scholar |
Krogh MA, Hostens M, Salavati M, Grelet C, Sorensen MT, Wathes DC, Ferris CP, Marchitelli C, Signorelli F, Napolitano F, Becker F, Larsen T, Matthews E, Carter F, Vanlierde A, Opsomer G, Gengler N, Dehareng F, Crowe MA, Ingvartsen KL, Foldager L (2020) Between-and within-herd variation in blood and milk biomarkers in Holstein cows in early lactation. Animal 14, 1067-1075.
| Crossref | Google Scholar |
Laubenthal L, Hoelker M, Frahm J, Dänicke S, Gerlach K, Südekum K-H, Sauerwein H, Häussler S (2016) Short communication: Telomere lengths in different tissues of dairy cows during early and late lactation. Journal of Dairy Science 99, 4881-4885.
| Crossref | Google Scholar |
Lawrence AB, Vigors B, Sandöe P (2019) What is so positive about positive animal welfare? A critical review of the literature. Animals 9, 783.
| Crossref | Google Scholar |
Li C, Hao X, Willms WD, Mcallister TA (2012) Responses of herbage and cattle tail switch hair δ15N value to long-term stocking rates on a rough fescue grassland. Soil Science and Plant Nutrition 58, 326-333.
| Crossref | Google Scholar |
Lin J, Epel E (2022) Stress and telomere shortening: insights from cellular mechanisms. Ageing Research Reviews 73, 101507.
| Crossref | Google Scholar |
Lindahl C, Lundqvist P, Norberg AL (2012) Swedish dairy farmers’ perceptions of animal-related injuries. Journal of Agromedicine 17, 364-376.
| Crossref | Google Scholar |
Llonch P, King EM, Clarke KA, Downes JM, Green LE (2015) A systematic review of animal based indicators of sheep welfare on farm, at market and during transport, and qualitative appraisal of their validity and feasibility for use in UK abattoirs. The Veterinary Journal 206, 289-297.
| Crossref | Google Scholar |
MacIntosh AJJ, Alados CL, Huffman MA (2011) Fractal analysis of behaviour in a wild primate: behavioural complexity in health and disease. Journal of The Royal Society Interface 8, 1497-1509.
| Crossref | Google Scholar |
Main DCJ, Whay HR, Leeb C, Webster AJF (2007) Formal animal-based welfare assessment in UK certification schemes. Animal Welfare 16, 233-236.
| Crossref | Google Scholar |
Mainland I, Towers J, Ewens V, Davis G, Montgomery J, Batey C, Card N, Downes J (2016) Toiling with teeth: an integrated dental analysis of sheep and cattle dentition in Iron Age and Viking–Late Norse Orkney. Journal of Archaeological Science: Reports 6, 837-855.
| Crossref | Google Scholar |
Maller CJ, Hemsworth PH, Ng KT, Jongman EJ, Coleman GJ, Arnold NA (2005) The relationships between characteristics of milking sheds and the attitudes to dairy cows, working conditions, and quality of life of dairy farmers. Australian Journal of Agricultural Research 56, 363-372.
| Crossref | Google Scholar |
Marsh L, Hutchinson MR, McLaughlan C, Musolino ST, Hebart ML, Terry R, Verma PJ, Hiendleder S, Whittaker AL (2021) Evaluation of miRNA as biomarkers of emotional valence in pigs. Animals 11, 2054.
| Crossref | Google Scholar |
Masters DG, Peterson AD, Mata G, Liu SM (1998) Influence of liveweight, liveweight change, and diet on wool growth, staple strength, and fibre diameter in young sheep. Australian Journal of Agricultural Research 49, 269-278.
| Crossref | Google Scholar |
Mattalia S, Rocland M, Aguerre S, Poppe M (2022) Test-day genetic evaluations: a tool to measure herd resilience through monthly milk records. In ‘Proceedings of 12th World Congress on Genetics Applied to Livestock Production (WCGALP): technical and species orientated innovations in animal breeding, and contribution of genetics to solving societal challenges, Rotterdam’. (Eds RF Veerkamp, Y de Haas) pp. 651–654. (Wageningen Academic Publishers: Netherlands)
McEwen BS (2003) Interacting mediators of allostasis and allostatic load: towards an understanding of resilience in aging. Metabolism 52, 10-16.
| Crossref | Google Scholar |
McEwen BS, Stellar E (1993) Stress and the individual: mechanisms leading to disease. Archives of Internal Medicine 153, 2093-2101.
| Crossref | Google Scholar |
Mellor DJ (2015) Enhancing animal welfare by creating opportunities for positive affective engagement. New Zealand Veterinary Journal 63, 3-8.
| Crossref | Google Scholar |
Mellor DJ (2016) Updating animal welfare thinking: moving beyond the ‘Five Freedoms’ towards ‘a Life Worth Living’. Animals 6, 21.
| Crossref | Google Scholar |
Mellor DJ (2019) Preparing for life after birth: introducing the concepts of intrauterine and extrauterine sensory entrainment in mammalian young. Animals 9, 826.
| Crossref | Google Scholar |
Mendl M, Burman OHP, Paul ES (2010) An integrative and functional framework for the study of animal emotion and mood. Proceedings of the Royal Society B: Biological Sciences 277, 2895-2904.
| Crossref | Google Scholar |
Mengistu SB, Mulder HA, Bastiaansen JWM, Benzie JAH, Khaw HL, Trinh TQ, Komen H (2022) Fluctuations in growth are heritable and a potential indicator of resilience in Nile tilapia (Oreochromis niloticus). Aquaculture 560, 738481.
| Crossref | Google Scholar |
Miller LJ, Vicino GA, Sheftel J, Lauderdale LK (2020) Behavioral diversity as a potential indicator of positive animal welfare. Animals 10, 1211.
| Crossref | Google Scholar |
Miretti S, Lecchi C, Ceciliani F, Baratta M (2020) MicroRNAs as biomarkers for animal health and welfare in livestock. Frontiers in Veterinary Science 7, 578193.
| Crossref | Google Scholar |
Monk JE, Hine BC, Colditz IG, Lee C (2018) A novel protocol to assess acclimation rate in Bos taurus heifers during yard weaning. Animals 8, 51.
| Crossref | Google Scholar |
Moore-Ede MC (1986) Physiology of the circadian timing system: predictive versus reactive homeostasis. American Journal of Physiology: Regulatory, Integrative and Comparative Physiology 250, R737-R752.
| Crossref | Google Scholar |
Moreno García CA, Maxwell TMR, Hickford J, Gregorini P (2020) On the search for grazing personalities: from individual to collective behaviors. Frontiers in Veterinary Science 7, 74.
| Crossref | Google Scholar |
Moreno García CA, Zhou H, Altimira D, Dynes R, Gregorini P, Jayathunga S, Maxwell TMR, Hickford J (2022) The glutamate metabotropic receptor 5 (GRM5) gene is associated with beef cattle home range and movement tortuosity. Journal of Animal Science and Biotechnology 13, 111.
| Crossref | Google Scholar |
Mormède P, Andanson S, Aupérin B, Beerda B, Guémené D, Malmkvist J, Manteca X, Manteuffel G, Prunet P, van Reenen CG, Richard S, Veissier I (2007) Exploration of the hypothalamic–pituitary–adrenal function as a tool to evaluate animal welfare. Physiology & Behavior 92, 317-339.
| Crossref | Google Scholar |
Nagatsu M, Salmela M (2022) Interpersonal and collective affective niche construction: empirical and normative perspectives on social media. Review of Philosophy and Psychology
| Crossref | Google Scholar |
Neave HW, Costa JHC, Weary DM, von Keyserlingk MAG (2020) Long-term consistency of personality traits of cattle. Royal Society Open Science 7, 191849.
| Crossref | Google Scholar |
Nery da Silva A, Silva Araujo M, Pértille F, Zanella AJ (2022) How epigenetics can enhance pig welfare? Animals 12, 32.
| Crossref | Google Scholar |
Neville AC (1967) Daily growth layers in animals and plants. Biological Reviews 42, 421-439.
| Crossref | Google Scholar |
Nunes Marsiglio Sarout B, Waterhouse A, Duthie C-A, Candal Poli CHE, Haskell MJ, Berger A, Umstatter C (2018) Assessment of circadian rhythm of activity combined with random regression model as a novel approach to monitoring sheep in an extensive system. Applied Animal Behaviour Science 207, 26-38.
| Crossref | Google Scholar |
Otten W, Heimbürge S, Tuchscherer A, Kanitz E (2022) The age of hair matters: the incorporation of cortisol by external contamination is enhanced in distal hair segments of pigs and cattle. Animal 16, 100495.
| Crossref | Google Scholar |
Parois SP, Van Der Zande LE, Knol EF, Kemp B, Rodenburg TB, Bolhuis JE (2022) A multi-suckling system combined with an enriched housing environment during the growing period promotes resilience to various challenges in pigs. Scientific Reports 12, 6804.
| Crossref | Google Scholar |
Pértille F, Ibelli AMG, Sharif ME, Poleti MD, Fröhlich AS, Rezaei S, Ledur MC, Jensen P, Guerrero-Bosagna C, Coutinho LL (2020) Putative epigenetic biomarkers of stress in red blood cells of chickens reared across different biomes. Frontiers in Genetics 11, 508809.
| Crossref | Google Scholar |
Plaut K, Casey T (2012) Does the circadian system regulate lactation? Animal 6, 394-402.
| Crossref | Google Scholar |
Poppe M, Veerkamp RF, Van Pelt ML, Mulder HA (2020) Exploration of variance, autocorrelation, and skewness of deviations from lactation curves as resilience indicators for breeding. Journal of Dairy Science 103, 1667-1684.
| Crossref | Google Scholar |
Poppe M, Mulder HA, Veerkamp RF (2021) Validation of resilience indicators by estimating genetic correlations among daughter groups and with yield responses to a heat wave and disturbances at herd level. Journal of Dairy Science 104, 8094-8106.
| Crossref | Google Scholar |
Poppe M, Veerkamp RF, Mulder HA, Hogeveen H (2022) Observational study on associations between resilience indicators based on daily milk yield in first lactation and lifetime profitability. Journal of Dairy Science 105, 8158-8176.
| Crossref | Google Scholar |
Porto R, Duarte L, Menna-Barreto L (2006) Circadian variation of mood: comparison between different chronotypes. Biological Rhythm Research 37, 425-431.
| Crossref | Google Scholar |
Probo M, Veronesi MC (2022) Clinical scoring systems in the newborn calf: an overview. Animals 12, 3013.
| Crossref | Google Scholar |
Promislow D, Anderson RM, Scheffer M, Crespi B, DeGregori J, Harris K, Horowitz BN, Levine ME, Riolo MA, Schneider DS, Spencer SL, Valenzano DR, Hochberg ME (2022) Resilience integrates concepts in aging research. iScience 25, 104199.
| Crossref | Google Scholar |
Putz AM, Harding JCS, Dyck MK, Fortin F, Plastow GS, Dekkers JCM, Canada P (2019) Novel resilience phenotypes using feed intake data from a natural disease challenge model in wean-to-finish pigs. Frontiers in Genetics 9, 660.
| Crossref | Google Scholar |
Pyrkov TV, Avchaciov K, Tarkhov AE, Menshikov LI, Gudkov AV, Fedichev PO (2021) Longitudinal analysis of blood markers reveals progressive loss of resilience and predicts human lifespan limit. Nature Communications 12, 2765.
| Crossref | Google Scholar |
Quesnel H, Resmond R, Merlot E, Père M-C, Gondret F, Louveau I (2023) Physiological traits of newborn piglets associated with colostrum intake, neonatal survival and preweaning growth. animal 100843.
| Crossref | Google Scholar |
Quoidbach J, Gruber J, Mikolajczak M, Kogan A, Kotsou I, Norton MI (2014) Emodiversity and the emotional ecosystem. Journal of Experimental Psychology: General 143, 2057-2066.
| Crossref | Google Scholar |
Ralph CR, Tilbrook AJ (2016) Invited review: the usefulness of measuring glucocorticoids for assessing animal welfare. Journal of Animal Science 94, 457-470.
| Crossref | Google Scholar |
Rao R, Androulakis IP (2019) The physiological significance of the circadian dynamics of the HPA axis: interplay between circadian rhythms, allostasis and stress resilience. Hormones and Behavior 110, 77-89.
| Crossref | Google Scholar |
Rault J-L, Hintze S, Camerlink I, Yee JR (2020) Positive welfare and the like: distinct views and a proposed framework. Frontiers in Veterinary Science 7, 370.
| Crossref | Google Scholar |
Revilla M, Friggens NC, Broudiscou LP, Lemonnier G, Blanc F, Ravon L, Mercat MJ, Billon Y, Rogel-Gaillard C, Le Floch N, Estellé J, Muñoz-Tamayo R (2019) Towards the quantitative characterisation of piglets’ robustness to weaning: a modelling approach. Animal 13, 2536-2546.
| Crossref | Google Scholar |
Rhodes V, Maguire M, Shetty M, McAloon C, Smeaton AF (2022) Periodicity intensity of the 24 h circadian rhythm in newborn calves show indicators of herd welfare. Sensors 22, 5843.
| Crossref | Google Scholar |
Ribeiro AMF, Sanglard LP, Wijesena HR, Ciobanu DC, Horvath S, Spangler ML (2022) DNA methylation profile in beef cattle is influenced by additive genetics and age. Scientific Reports 12, 12016.
| Crossref | Google Scholar |
Rogers GE (2006) Biology of the wool follicle: an excursion into a unique tissue interaction system waiting to be re-discovered. Experimental Dermatology 15, 931-949.
| Crossref | Google Scholar |
Romero LM, Dickens MJ, Cyr NE (2009) The reactive scope model – a new model integrating homeostasis, allostasis, and stress. Hormones and Behavior 55, 375-389.
| Crossref | Google Scholar |
Rowe E, Mullan S (2022) Advancing a ‘good life’ for farm animals: development of resource tier frameworks for on-farm assessment of positive welfare for beef cattle, broiler chicken and pigs. Animals 12, 565.
| Crossref | Google Scholar |
Ryff CD, Singer B (1998) The contours of positive human health. Psychological Inquiry 9, 1-28.
| Crossref | Google Scholar |
Rysava K, McGill RAR, Matthiopoulos J, Hopcraft JGC (2016) Re-constructing nutritional history of Serengeti wildebeest from stable isotopes in tail hair: seasonal starvation patterns in an obligate grazer. Rapid Communications in Mass Spectrometry 30, 1461-1468.
| Crossref | Google Scholar |
Sales AJ, Guimarães FS, Joca SRL (2021) DNA methylation in stress and depression: from biomarker to therapeutics. Acta Neuropsychiatrica 33, 217-241.
| Crossref | Google Scholar |
Sandöe P, Forkman B, Christiansen SB (2004) Scientific uncertainty: how should it be handled in relation to scientific advice regarding animal welfare issues? Animal Welfare 13, S121-S126.
| Crossref | Google Scholar |
Sangild PT, Thymann T, Schmidt M, Stoll B, Burrin DG, Buddington RK (2013) Invited review: the preterm pig as a model in pediatric gastroenterology. Journal of Animal Science 91, 4713-4729.
| Crossref | Google Scholar |
Scheffer M, Bascompte J, Brock WA, Brovkin V, Carpenter SR, Dakos V, Held H, van Nes EH, Rietkerk M, Sugihara G (2009) Early-warning signals for critical transitions. Nature 461, 53-59.
| Crossref | Google Scholar |
Scheffer M, Bolhuis JE, Borsboom D, Buchman TG, Gijzel SMW, Goulson D, Kammenga JE, Kemp B, van de Leemput IA, Levin S, Martin CM, Melis RJF, van Nes EH, Romero LM, Olde Rikkert MGM (2018) Quantifying resilience of humans and other animals. Proceedings of the National Academy of Sciences 115, 11883-11890.
| Crossref | Google Scholar |
Scheibe KM, Berger A, Langbein J, Streich WJ, Eichhorn K (1999) Comparative analysis of ultradian and circadian behavioural rhythms for diagnosis of biorhythmic state of animals. Biological Rhythm Research 30, 216-233.
| Crossref | Google Scholar |
Schiller D, Yu ANC, Alia-Klein N, Becker S, Cromwell HC, Dolcos F, Eslinger PJ, Frewen P, Kemp AH, Pace-Schott EF, et al. (2022) The human affectome. Available at https://hal.science/hal-03728066/
Schulkin J, Sterling P (2019) Allostasis: a brain-centered, predictive mode of physiological regulation. Trends in Neurosciences 42, 740-752.
| Crossref | Google Scholar |
Schwertl M, Auerswald K, Schnyder H (2003) Reconstruction of the isotopic history of animal diets by hair segmental analysis. Rapid Communications in Mass Spectrometry 17, 1312-1318.
| Crossref | Google Scholar |
Seeley KE, Proudfoot KL, Edes AN (2022) The application of allostasis and allostatic load in animal species: a scoping review. PLoS ONE 17, e0273838.
| Crossref | Google Scholar |
Seibt KD, Ghaffari MH, Scheu T, Koch C, Sauerwein H (2022) Effects of different feeding levels during a 14-week preweaning phase in dairy heifer calves on telomere length and mitochondrial DNA copy number in blood. Journal of Dairy Science 105, 8509-8522.
| Crossref | Google Scholar |
Shreesha L, Levin M (2023) Cellular competency during development alters evolutionary dynamics in an artificial embryogeny model. Entropy 25, 131.
| Crossref | Google Scholar |
Silva LFP, Hegarty RS, Meale SJ, Costa DAF, Fletcher MT (2022) Using the natural abundance of nitrogen isotopes to identify cattle with greater efficiency in protein-limiting diets. animal 16, 100551.
| Crossref | Google Scholar |
Smolyar IV, Bromage TG (2004) Discrete model of fish scale incremental pattern: a formalization of the 2D anisotropic structure. ICES Journal of Marine Science 61, 992-1003.
| Crossref | Google Scholar |
Špinka M (2019) Animal agency, animal awareness and animal welfare. Animal Welfare 28, 11-20.
| Crossref | Google Scholar |
Sterling P (2012) Allostasis: a model of predictive regulation. Physiology & Behavior 106, 5-15.
| Crossref | Google Scholar |
Stokes JE, Rowe E, Mullan S, Pritchard JC, Horler R, Haskell MJ, Dwyer CM, Main DCJ (2022) A ‘good life’ for dairy cattle: developing and piloting a framework for assessing positive welfare opportunities based on scientific evidence and farmer expertise. Animals 12, 2540.
| Crossref | Google Scholar |
Suckling GW (1989) Developmental defects of enamel-historical and present-day perspectives of their pathogenesis. Advances in Dental Research 3, 87-94.
| Crossref | Google Scholar |
Sun D, Webb L, van der Tol PPJ, van Reenen K (2021) A systematic review of automatic health monitoring in calves: glimpsing the future from current practice. Frontiers in Veterinary Science 8, 761468.
| Crossref | Google Scholar |
Tamminen L-M, Keeling LJ, Svensson A, Briot L, Emanuelson U (2021) Unraveling the complexity to observe associations between welfare indicators and hair cortisol concentration in dairy calves. Frontiers in Animal Science 2, 793558.
| Crossref | Google Scholar |
Tomlinson DJ, Mülling CH, Fakler TM (2004) Invited review: formation of keratins in the bovine claw: roles of hormones, minerals, and vitamins in functional claw integrity. Journal of Dairy Science 87, 797-809.
| Crossref | Google Scholar |
Towers J, Gledhill A, Bond J, Montgomery J (2014) An investigation of cattle birth seasonality using δ13C and δ18O profiles within first molar enamel. Archaeometry 56, 208-236.
| Crossref | Google Scholar |
Trimmer PC, Paul ES, Mendl MT, McNamara JM, Houston AI (2013) On the evolution and optimality of mood states. Behavioral Sciences 3, 501-521.
| Crossref | Google Scholar |
Turner PV (2019) Moving beyond the absence of pain and distress: focusing on positive animal welfare. ILAR Journal 60, 366-372.
| Crossref | Google Scholar |
Urano Y, Sugimoto Y, Tanoue K, Matsumoto R, Kawabe S, Ohashi T, Fujiwara SI (2019) The sandwich structure of keratinous layers controls the form and growth orientation of chicken rhinotheca. Journal of Anatomy 235, 299-312.
| Crossref | Google Scholar |
Urban-Wojcik EJ, Mumford JA, Almeida DM, Lachman ME, Ryff CD, Davidson RJ, Schaefer SM (2022) Emodiversity, health, and well-being in the Midlife in the United States (MIDUS) daily diary study. Emotion 22, 603-615.
| Crossref | Google Scholar |
van Dixhoorn IDE, de Mol RM, van der Werf JTN, van Mourik S, van Reenen CG (2018) Indicators of resilience during the transition period in dairy cows: a case study. Journal of Dairy Science 101, 10271-10282.
| Crossref | Google Scholar |
Vargovic L, Harper J-A, Bunter KL (2022) Traits defining sow lifetime maternal performance. Animals 12, 2451.
| Crossref | Google Scholar |
Veissier I, Le Neindre P, Trillat G (1989) The use of circadian behaviour to measure adaptation of calves to changes in their environment. Applied Animal Behaviour Science 22, 1-12.
| Crossref | Google Scholar |
Vetter VM, Kalies CH, Sommerer Y, Spira D, Drewelies J, Regitz-Zagrosek V, Bertram L, Gerstorf D, Demuth I (2022) Relationship between 5 epigenetic clocks, telomere length, and functional capacity assessed in older adults: cross-sectional and longitudinal analyses. The Journals of Gerontology: Series A 77, 1724-1733.
| Crossref | Google Scholar |
Vigors B, Sandøe P, Lawrence AB (2021) Positive welfare in science and society: differences, similarities and synergies. Frontiers in Animal Science 2, 738193.
| Crossref | Google Scholar |
Vinkers CH, Kalafateli AL, Rutten BP, Kas MJ, Kaminsky Z, Turner JD, Boks MP (2015) Traumatic stress and human DNA methylation: a critical review. Epigenomics 7, 593-608.
| Crossref | Google Scholar |
Wagner N, Mialon M-M, Sloth KH, Lardy R, Ledoux D, Silberberg M, de Boyer des Roches A, Veissier I (2021) Detection of changes in the circadian rhythm of cattle in relation to disease, stress, and reproductive events. Methods 186, 14-21.
| Crossref | Google Scholar |
Webb LE, Veenhoven RR, Harfeld JL, Jensen MB (2019) What is animal happiness? Annals of the New York Academy of Sciences 1438, 62-76.
| Crossref | Google Scholar |
Webster J (2013) International standards for farm animal welfare: science and values. The Veterinary Journal 198, 3-4.
| Crossref | Google Scholar |
Webster J (2016) Animal welfare: freedoms, dominions and ‘a life worth living’. Animals 6, 35.
| Crossref | Google Scholar |
Whitehead JC, Hildebrand BA, Sun M, Rockwood MR, Rose RA, Rockwood K, Howlett SE (2014) A clinical frailty index in aging mice: comparisons with frailty index data in humans. Journals of Gerontology Series A: Biomedical Sciences and Medical Sciences 69, 621-632.
| Crossref | Google Scholar |
Williams LA (2021) From human wellbeing to animal welfare. Neuroscience & Biobehavioral Reviews 131, 941-952.
| Crossref | Google Scholar |
Williams M, Sleator RD, Murphy CP, McCarthy J, Berry DP (2022) Exploiting genetic variability in the trajectory of lactation yield and somatic cell score with each progressing parity. Journal of Dairy Science 105, 3341-3354.
| Crossref | Google Scholar |
Woods HA, Wilson JK (2013) An information hypothesis for the evolution of homeostasis. Trends in Ecology & Evolution 28, 283-289.
| Crossref | Google Scholar |
Wu Y-C, Straathof EJ, Heineman KR, Hadders-Algra M (2020) Typical general movements at 2 to 4 months: movement complexity, fidgety movements, and their associations with risk factors and SINDA scores. Early Human Development 149, 105135.
| Crossref | Google Scholar |
Ye J, Medzhitov R (2019) Control strategies in systemic metabolism. Nature Metabolism 1, 947-957.
| Crossref | Google Scholar |
Yeates JW (2011) Is ‘a life worth living’ a concept worth having? Animal Welfare 20, 397-406.
| Crossref | Google Scholar |
Yeates JW, Main DCJ (2008) Assessment of positive welfare: a review. The Veterinary Journal 175, 293-300.
| Crossref | Google Scholar |
Zachut M, Šperanda M, de Almeida AM, Gabai G, Mobasheri A, Hernández-Castellano LE (2020) Biomarkers of fitness and welfare in dairy cattle: healthy productivity. Journal of Dairy Research 87, 4-13.
| Crossref | Google Scholar |
Zaiac MN, Walker A (2013) Nail abnormalities associated with systemic pathologies. Clinics in dermatology 31, 627-649.
| Crossref | Google Scholar |
Zazzo A, Balasse M, Patterson WP (2005) High-resolution δ13C intratooth profiles in bovine enamel: implications for mineralization pattern and isotopic attenuation. Geochimica et Cosmochimica Acta 69, 3631-3642.
| Crossref | Google Scholar |
Zazzo A, Harrison SM, Bahar B, Moloney AP, Monahan FJ, Scrimgeour CM, Schmidt O (2007) Experimental determination of dietary carbon turnover in bovine hair and hoof. Canadian Journal of Zoology 85, 1239-1248.
| Crossref | Google Scholar |