‘Superbull’ males: what role do they play and what drives their appearance within the Doryteuthis gahi Patagonian Shelf population?
Jessica B. Jones
A School of Biological Sciences, University of Aberdeen, Zoology Building, Tillydrone Avenue, Aberdeen, AB24 2TZ, Scotland, UK.
B Fisheries Department, Falkland Islands Government, Stanley, PO Box 598, FIQQ 1ZZ, Falkland Islands.
C South Atlantic Environmental Research Institute, PO Box 609, Stanley Cottage, Stanley, FIQQ 1ZZ, Falkland Islands.
D Centro de Estudos do Ambiente e do Mar, Universidade de Aveiro, PT-3810-193 Aveiro, Portugal.
E Departamento de Biologia, Universidade de Aveiro, PT-3810-193 Aveiro, Portugal.
F Instituto de Investigacións Mariñas (Consejo Superior de Investigaciones Cientificas), Eduardo Cabello 6, E-36208 Vigo, Spain.
G Corresponding author. Email: jjones@fisheries.gov.fk
Marine and Freshwater Research 70(12) 1805-1817 https://doi.org/10.1071/MF18285
Submitted: 2 August 2018 Accepted: 1 February 2019 Published: 12 April 2019
Journal Compilation © CSIRO 2019 Open Access CC BY-NC-ND
Abstract
Cephalopod populations exhibit high variability in life history characteristics, such as longevity and size-at-age. The aim of this study was to understand how characteristics of a newly described ‘superbull’ male morph in Doryteuthis gahi populations (Patagonian Shelf) arise and whether there is a selective advantage. At the population level, it is speculated that superbulls provide temporal and spatial connectivity, but individual benefit is less obvious. Age structure and reproductive potential of males was investigated to determine whether superbulls could provide connectivity. Environmental variables affecting size-at-age were explored to ascertain whether morphological differences were primarily phenotypically driven. Superbulls from the autumn spawning cohort were significantly older than the residual population, with added longevity potentially leading to spawning with the following cohort. A reduction in relative testis weight was apparent in superbulls, but spermatophore production remained high. Generalised additive mixed models indicated temperature, location and hatch year had significant effects on size-at-age. Weak correlations between warm El Niño–Southern Oscillation phases and superbull abundance were found. The results suggest that superbulls provide temporal connectivity and arise through phenotypic plasticity, likely providing connectivity as a side effect of body shape and size rather than a genetically selected advantage.
Additional keywords: cephalopods, plasticity, population structure, reproduction, southwest Atlantic, statolith.
Introduction
Many fish populations have a multigenerational structure that provides a reserve of reproductive potential and genetic variability. With several year classes of reproductively viable adults available, this risk-spreading strategy reduces the potential for population collapse (O’Dor 1998). Conversely, most cephalopods are characterised by an annual life cycle. With annual recruitment depending solely on the success of a single generation, cephalopods are thought to compensate for a lack of temporal risk spreading by producing genetically and phenotypically diverse offspring that are able to survive in a heterogeneous marine environment (Pierce and Guerra 1994; O’Dor 1998). Although this is a group selectionist argument for phenotypic plasticity, it is plausible if many populations exist and population persistence depends on plasticity.
The Patagonian long-finned squid Doryteuthis gahi is abundant around the Falkland Islands, where it is targeted by a commercial trawl fishery (Arkhipkin et al. 2007). These squid undergo spatial ontogenetic migrations from their shallow inshore spawning and nursery grounds (depth ~5–50 m) to offshore feeding grounds on the Falklands shelf (depth ~200–350 m; Arkhipkin et al. 2004). D. gahi is a small-sized squid typically attaining 13–16-cm dorsal mantle length (DML; Arkhipkin et al. 2007). Squid are targeted during two commercial fishing seasons, which correspond to the appearance of the two spawning cohorts, namely the spring spawning cohort (SSC) and the autumn spawning cohort (ASC), on their shared feeding grounds (Patterson 1988; Hatfield et al. 1990; Arkhipkin and Middleton 2003; Arkhipkin et al. 2007).
Most myopsid squid populations are characterised by the presence of two size-dependent behavioural morphotypes of mature males on spawning grounds (Hall and Hanlon 2002; Hanlon et al. 2002; Wada et al. 2005; Shashar and Hanlon 2013): (1) large males, referred to as ‘bull’ males, have dominant behaviour, forming mating pairs with females; and (2) smaller subordinate individuals or ‘sneaker’ males that are of similar size or smaller than females. The small size of ‘sneaker’ males facilitates a close approach to mating pairs, where the ‘sneaker’ males then attempt to elicit extrapair copulations (EPCs; Hanlon and Messenger 1998). Some authors have pointed to evidence for the existence of more than two size groups in males, for example Collins et al. (1999) for Loligo forbesii, implying that plasticity in growth form extends beyond the existence of two reproductive strategies in males. Anomalously large males, much larger than the typical mature males, have been found to occur in populations of several loliginid squid (Mangold 1987; Pierce et al. 2013; Jones et al. 2019). A recent study investigating the intrapopulation body shape variation of D. gahi found that males exceeding 20–25 cm DML had a significantly different body shape than the rest of the population and were suggested to be a third ‘superbull’ morph (Jones et al. 2019). These superbull males had a substantially more elongated body, a heavier fin and a larger fin area than the rest of the population.
Owing to the streamlined shape and large fins characteristic of this morph, it is likely that these superbull males have enhanced gliding abilities and are able to undertake longer migrations than the rest of the population (Arkhipkin et al. 2015a). It was therefore hypothesised that these superbull males extend their migratory routes, providing geographic connectivity by migrating between remote spawning areas (Jones et al. 2019). In other species, anomalously large individuals have delayed maturation and an extended life cycle. For example, in the eastern Pacific, temperature determines whether jumbo squid Dosidicus gigas have a 1-year life cycle (early maturation and a small size) or a 1.5- to 2-year life cycle (delayed maturation and a large size; Arkhipkin et al. 2015b). If this is the case for D. gahi, these individuals may also potentially maintain temporal connectivity by reproducing with the following spawning cohort.
The intrinsic or extrinsic mechanisms driving the existence of these superbull males are presently unknown and the hypothesis that they provide geographical or temporal connectivity is yet to be confirmed. Given that (spatial or temporal) connectivity is a population-level phenomenon, the existence of superbull males might be explained by either a selective advantage at an individual level (e.g. enhanced reproductive output relative to smaller males, either through increased gamete holding capacity or through increased success in conflicts with other males) or selective pressure favouring phenotypic variability, the expression of which may be environmentally driven (O’Dor 1998). It is plausible that populations with higher individual variability or plasticity are more persistent. Several sources of evidence are relevant to the existence of superbulls and the selective pressures at work:
-
evidence of enhanced swimming ability or longer life would indicate the potential for providing spatial or temporal connectivity
-
evidence of superbulls mating with squid in distant locations or with squid from the next generation would confirm that connectivity is achieved
-
evidence of enhanced reproductive output would confirm the possibility of a direct selective advantage for superbulls, if this characteristic is selected
-
evidence of consistent genetic differences at one or more locus, between superbulls and other males, would suggest the superbull characteristics are heritable
-
evidence that the occurrence of superbulls is environmentally driven would provide circumstantial evidence against heritability.
Considering that the superbulls exhibit a high degree of interannual variation in abundance, it is likely that their occurrence is driven by extrinsic phenotypic variation rather than any genetic influence. Small changes in environmental conditions (most often temperature) and food availability are known to have significant effects on life history characteristics such as longevity and size-at-age in squid (Pecl et al. 2004). Variability of growth in D. gahi has been largely attributed to variation in temperature during early life stages, with squid hatched in summer (at higher temperatures) being significantly larger than squid of the same age hatched during the winter months (Hatfield 2000). Clearly environmental influences have a marked effect on the population characteristics of this species. Indeed, large-scale events such as the propagation of the El Niño–Southern Oscillation (ENSO) from the Pacific Ocean have been correlated with population abundance in D. gahi (Waluda et al. 2004). The ENSO is recognised as an important diver of sea surface temperature (SST) variability in south-west Atlantic and Antarctic waters (Trathan and Murphy 2003; Meredith et al. 2008; Baylis et al. 2012). However, the effects of large-scale events on the size-at-age relationship have not yet been investigated.
The aim of this study was to quantify the reproductive output and compare squid age between spawning cohorts and between superbulls and smaller males to investigate the potential that superbulls provide temporal or geographic connectivity. In addition, the effect of extrinsic variables, predominantly temperature and food availability, on the size-at-age relationship was investigated to determine whether the appearance of superbull males is phenotypically driven, the hypothesis being that these males have experienced similar environmental conditions that have resulted in their large size. We examined this in two ways: (1) comparing size-at-age relationships across years to see whether differences are environmentally driven; and (2) relating the annual abundance of superbulls to large-scale temperature anomalies.
Materials and methods
Biological sampling
An existing dataset representative of the squid population within the Falkland Islands interim conservation and management zone (FICZ) and international waters north of the zone was extended during this analysis to incorporate additional superbull males (defined as those exceeding 25 cm DML; Fig. 1), which are a rare component of the fishery catch. Data were sourced from three different datasets from the Falkland Islands Fisheries Department, as detailed below:
-
Data source 1: random samples of aged male specimens (n = 7505) collected and frozen by scientific observers on board commercial fishing vessels during the two commercial fishing seasons (Season 1: March–May; Season 2: July–October) and from two research cruises (the RV Dorada and RV Castelo) between fishing seasons (between 1998 and 2007); measurements and statoliths were taken from fortnightly samples of ‘mixed’ squid (unsorted by size; Table 1)
-
Data source 2: non-random aged samples including rare superbull males exceeding 25 cm DML (n = 162), collected and frozen during 2014 and 2015, to ensure squid of a large size were represented within the sample (Table 1)
-
Data source 3: random length–frequency data (n = 921 742) from males collected daily by scientific observers during the two commercial fishing seasons within the designated fishing zone (the ‘Loligo box’; Fig. 1) between 1998 and 2017 for construction of length–frequency graphs and distributional maps to aid in data interpretation.
![]() |
![]() |
Frozen samples from Data sources 1 and 2 were thawed overnight and processed in the Falkland Islands Fisheries Department laboratory. Squid were measured (DML; ±1 mm), weighed (total bodyweight (BW); ±1 g) and visually assessed for sex and maturity stage according to a modified version of Lipinski (1979), using a maturity scale ranging from 1 (immature) to 6 (spent). Statoliths were dissected from the cephalic cartilage and stored in 96% ethanol. A subsample of aged males (n = 386) collected between 1999 and 2007 had additional data collected including mantle weight (MW), digestive gland weight (DGW) and reproductive organ weights (i.e. testis weight (TW), Needham’s complex weight (NCW) and the reproductive system weight, RW = TW + NCW). To determine how the proportion of somatic weight allocated to different organs changes relative to size, four indices were computed, defined as:
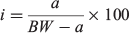
where I is the gonadosomatic index (GSI), testis index (TWI), Needham’s complex index (NCI) or digestive gland index (DGI) and a is the corresponding organ weight (RW, TW, NCW or DGW). Samples from Data source 3 were processed at sea and were measured for DML (±5 mm) and visually assessed for maturity stage.
Age estimation
One statolith per specimen was mounted concave side up for age analysis, then ground and polished on both sides using wet waterproof grinding paper to expose the nucleus. The thin transverse section produced was embedded in mounting medium (Canada balsam, Merck KGaA, Darmstadt, Germany) and covered with a cover glass for observation. Statoliths were read under the transmitted light of an Olympus BX51 compound microscope (Olympus Corporation, Tokyo, Japan) at 400× magnification, with a phase-contrast Nomarski effect used to improve readability (Arkhipkin and Shcherbich 2012).
Statoliths were first read in the lateral dome from the natal ring to the first prominent check. This check was followed into the rostrum and read in the rostrum to the tip. Because the ‘one ring = one day’ hypothesis has been validated in several loliginid species, including Sepioteuthis lessoniana (Jackson et al. 1993), Lolliguncula brevis (Jackson et al. 1997), Loligo reynaudii (Lipinski et al. 1998), Loligo vulgaris (Villanueva 2000) and Loligo plei (Jackson and Forsythe 2002), total increment number was assumed to represent postembryonic age in days (from here onwards referred to as age). To minimise counting errors, the total number of growth increments for each specimen was taken as the mean of at least two counts. If the difference between these counts exceeded 10%, a third count was made. If the difference between the second and third readings still exceeded 10%, the statolith was rejected from further age analysis. For Data source 1, statolith ages were intermittently verified by a second reader. For Data source 2, 6% of age counts were verified by a second reader.
Environmental variables
SST data were extracted from the Integrated Global Ocean Services System database (IGOSS nmc Reyn_SmithOIv2 monthly; http://iridl.ldeo.columbia.edu/SOURCES/.IGOSS/.nmc/.Reyn_SmithOIv2/.monthly/, accessed 20 February 2018) to determine how average monthly SST affected the size-at-age relationship (Reynolds et al. 2002). SST data were collected between 53.5 and 45.5°S and 63.5 and 56.5°W to obtain average monthly SST values for the whole sampling area. Mean monthly chlorophyll-a concentration (mg m–3) was extracted for the same area from the European Union Copernicus marine service information system and was used as a proxy for productivity at month of hatching and the month following month of hatching (to account for a lag between primary production and zooplankton production). Data were derived from global ocean colour satellite observations (L4_NRT_OBSERVATIONS_009_033 and L4_REP_OBSERVATIONS_009_082) at a resolution of 4 × 4 km using SeaWiFS, MODIS-Aqua, MERIS, VIIRSN and OLCI-S3A sensors (http://marine.copernicus.eu, accessed 20 February 2018).
To assess whether the number of superbull males appearing in the fishery was affected by broad-scale climate variability in temperature, the southern oscillation index (SOI) was used. The SOI is the standardised difference in surface pressure anomalies between Tahiti in the Central Pacific (17°33′S, 149°37′W) and Darwin in Australia (12°28′S, 130°51′E), with negative SOI values indicating a warm phase of the ENSO. Yearly SOI values were accessed on the Australian government Bureau of Meteorology website (http://www.bom.gov.au/climate/current/soihtm1.shtml, accessed 15 February 2018).
Statistical analysis
Reproductive indices were plotted against squid size (DML) and fitted with local regression smoothers (locally estimated scatterplot smoothing (LOESS)) to visually assess reproductive output, with DGI also plotted with LOESS to investigate whether energy for gonad development was derived from stored sources (most often sourced from the lipid-rich digestive gland) or diet. Hatch date was determined by back-calculation of the total number of increments from the date of capture. Based on hatch date, each male was assigned to a spawning cohort. Squid hatched between April and November were considered to belong to the ASC and those hatching between December and March were assigned to the SSC (Arkhipkin et al. 2013). Data exploration was undertaken for aged samples following procedures described by Zuur et al. (2010). Extreme outliers identified in bivariate scatterplots and Cleveland dotplots were removed. Nine recorded values were considered implausible (0.001% of the dataset) and were discarded before analysis. To explore the potential that superbulls provide temporal connectivity between cohorts, age was modelled by means of a generalised linear model (GLM) with a Gaussian distribution and an identity link:

where ϵi ~ N(0,σ2), Ai is the post-hatching age, in days, of individual squid i, cohort is fitted as a categorical variable with two levels (ASC and SSC) and type is fitted as a categorical variable with two levels (superbull (≥25 cm DML) and normal (<25 cm DML)). The significance of an interaction term between cohort and type was evaluated by comparing GLM models with and without an interaction, using a Chi-squared test. Only mature male squid (Maturity stages 5 and 6) were used in this analysis (n = 3883). Effects from the GLM model were exhibited using the package ‘effects’ (Fox 2003) in the R environment (ver. 3.3.0, R Foundation for Statistical Computing, Vienna, Austria, see https://www.R-project.org/, accessed 25 July 2018).
Generalised additive models (GAMs) and generalised additive mixed models (GAMMs) with Gaussian distributions and identity links were used to describe variation in squid size (DML) in relation to environmental variables, using data from all aged males that had complete sets of environmental data (n = 7308). Several models were fitted, with Akaike information criterion (AIC; Akaike 1973) and Akaike weights used in conjunction with a forwards model selection procedure (based on biological knowledge of the species and the objectives of the study) to identify the optimal model (Wagenmakers and Farrell 2004). Examination of the residuals of the simplest model, using DML as the response variable and age as the smoothed explanatory variable, suggested a transformation was necessary to avoid violating the assumptions of normality and to reduce heterogeneity. A Box–Cox transformation (Box and Cox 1964) was applied to DML in all models, which improved residual fit, defined as:
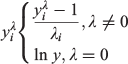
where yi is the DML of an individual squid i and λ is an exponent between –5 and 5 that transforms the data to the best approximation of a normal distribution curve. To incorporate dependency among observations found in the same level of maturity, the addition of a random intercept for each maturity stage (categorical; six levels) was investigated (i.e. aj, if squid i is from maturity level j, where aj are assumed to be independent and identically distributed (i.i.d.) random effects) (Wood 2008). Putative explanatory variables were as follows: hatch_yr (year of hatch, categorical, 13 levels), col_year (year of sample collection, categorical, 11 levels) age (days, continuous), lon,lat (of collection, continuous), area (of collection, spatial categorical data with four levels, see Fig. 1), SST (SST during month of hatching (°C), continuous), CHL (chlorophyll-a during month of hatching (mg g–1), continuous) and CHL+1 (chlorophyll-a during month after hatching (mg g–1), continuous). Either hatch_yr or col_year was incorporated into the model to account for interannual variation in size. Multipanel scatter plots and variance inflation factors (VIF) indicated strong collinearity between SST and CHL (Zuur et al. 2010). Therefore, the covariates SST and CHL were included in separate models. Smoothers for fixed variables were fitted using thin plate regression splines (Wood 2003). To avoid oversmoothing and to make the relationship between the response and explanatory variable clearer and more interpretable, the number of degrees of freedom for the environmental variables SST, CHL and CHL+1 was restricted to 6. Model assumptions were verified by plotting residuals against fitted values and against all potential covariates (Zuur and Ieno 2016). The additions of certain fixed effects were verified by applying F-tests. All statistical modelling was performed in the package ‘mgcv’ (Wood 2011) in R (R Foundation for Statistical Computing).
Cross-correlation analysis was used to determine the correlation between SOI anomalies and absolute abundance of superbull males. Absolute abundance was calculated by multiplying the number of superbulls found in Data source 3 in each year by a catch factor. The catch factor divided the total annual catch (obtained from fishery statistics reports; Falkland Islands Government 2017) by the minimum total catch found for the period 1998–2015 to improve the accuracy of interannual comparisons.
Results
Reproductive v. somatic growth
BW, MW and DGW showed a consistent increase with increasing DML (Fig. 2a–c). DGW showed more variability than the other two weight variables. However, RW, which is the sum of TW and NCW, reached an asymptote at 25–30 cm DML and showed a high degree of variation (Fig. 2d). When the constituents of RW were considered separately, TW started to decline after 25–30 cm DML (Fig. 2e), whereas NCW consistently increased with body size (Fig. 2f).
Reproductive and digestive gland indices were calculated for each individual (Fig. 3). Above 25 cm DML, the GSI dropped markedly along with the TWI. There was a negative linear relationship between DGI and squid size. The NCI increased from ~15 cm DML, reaching an asymptote at 23–25 cm DML. This suggests that although the RW is increasing (Fig. 2d), less energy is being directed into reproductive growth for individuals exceeding 25 cm DML.
![]() |
Age structure
The modal size of mature males was 14 cm DML, with a mean size of 17.4 cm DML and a maximum size of 44.5 cm DML. Of the 3883 mature-aged specimens, 427 individuals were superbull males exceeding 25 cm DML. Of these, there were 234 superbull males assigned to the ASC and 193 assigned to the SSC, showing a similar distribution of month of hatching (Fig. 4a) to the rest of the male population (Fig. 4b). Peak hatching for both superbulls and the main population was found during December–January, with a second smaller hatching peak during July–September in the main population and August–October in the superbulls (despite peaks in hatch date, superbulls were still a small proportion of the entire population during these months). Hatching occurred almost all year round. Superbulls of the SSC were only collected in 4 months, peaking from September–October and those of the ASC were found in 8 months, with two main peaks in May and August. Maximum post-hatching age in male D. gahi was estimated at 368 days (a 41 cm DML superbull individual), the only individual exceeding 360 days. The GLM applied to the mature aged males indicated that the SSC was significantly younger than the ASC (t = –7.65, P < 0.001) and that the superbull males were significantly older than normal-sized males in both cohorts (t = 22.87, P < 0.001; Fig. 5). A likelihood ratio test indicated that the inclusion of an interaction term significantly improved model fit (χ2 = 10 541, P < 0.001). Superbull males found within the ASC were the oldest with a predicted mean age of 286 days. Superbull males had a larger variation in age than the normal male morph (Fig. 5).
![]() |
![]() |
Environmental effects
The candidate models that explored the effects of environmental variables on the size-at-age relationship are given in Table 2. The inclusion of year of hatch (Model 2) rather than year of collection (Model 1) lowered the AIC and significantly improved model fit (d.f. = 2.43, F = 126.2, P < 0.001). To remove the effect of maturity stage, a random intercept was applied for each level of maturity, which also had a significant positive effect on model fit (d.f. = 3.82, F = 65.1, P < 0.001 using the restricted maximum likelihood (REML) estimation; see Fig. S1 of the Supplementary material). Although the inclusion of all three environmental variables (SST in month-of-hatch, chlorophyll-a in month of hatch and chlorophyll-a in month after hatch) lowered the AIC, SST improved model fit the most, followed by chlorophyll-a in month of hatch (see Fig. S2 of the Supplementary material). We assessed the residuals of Model 4 for spatial dependency and could not find any obvious patterns in the residuals (see Fig. S3 of the Supplementary material). However, we included spatial components at different spatial scales, with AIC favouring the simpler spatial model, separating the population into four broad regions (‘Loligo box’, n = 5521; west, n = 844; north, n = 578; international, n = 365; Fig. 1). Partial effects of the smooth from the more complex Model 7, using longitude and latitude, suggested the largest individuals were located in the north-west coastal waters of west Falklands and, more generally, all inshore coastal regions around the Falkland Islands (Fig. 6). Large individuals were also located in western international waters in the partial effects, but this could be an artefact resulting from the small sample size in this region. Based on raw distributional plots of superbull males collected during the first and second fishing seasons, first-season superbulls were often caught to the west of the Falkland Islands (as suggested by the partial effects of Model 7), contrary to normal-sized males of the first and second seasons, which were caught within the ‘Loligo box’ region. Second-season superbulls occurred more often to the north of the ‘Loligo box’ (for distributional plots, see Fig. S4–S7 of the Supplementary material).
![]() |
The most parsimonious model in Table 2 was Model 9, in which the age effect had a smooth term that differed by area (Fig. 7). Comparison of models with and without separate smooths for the effect of age indicated that the inclusion of separate smooths significantly improved the model (d.f. = 7.87, F = 13.5, P < 0.001). The shape of all the smoothers for the effect of age demonstrated that squid of a larger size were older. There appears to be a discontinuity in the age–length relationship in the ‘Loligo box’ area at ~200 and 300 days (Fig. 7b). To the north of the zone, the shape of the smoother for the effect of age was almost linear (Fig. 7c). As expected, error increased at model extremes. Partial effects of area suggest that the ‘Loligo box’ area has the largest individuals, whereas areas in the rest of the conservation zone (north and west) have the smallest individuals (Fig. 8a). The optimal fitted GAMM demonstrated that SST (F = 145.18, P < 0.001) had a significant negative linear relationship with squid size, with error increasing with SST (Fig. 8b). Within this model, the effect of SST became a negative linear effect. Model validation indicated that no strong residual patterns were evident (see Fig. S8 of the Supplementary material).
![]() |
![]() |
Between-year differences
Annual SOI (Fig. 9a) was compared to the absolute abundance of large males exceeding 25 cm DML to determine how broad-scale environmental variation affects their presence in the fishery, given that there is a high degree of interannual variability in population size distribution (Fig. 9b). It seems that the appearance of superbull males coincides with negative SOI anomalies (i.e. warm ENSO phases), contrary to the results of the GAMM analysis, which found that squid achieve a larger size-at-age at higher temperatures during month of hatching. However, cross-correlation analysis indicates that the correlation between SOI and superbull absolute abundance is weak (–0.37 with a lag of 1 year) and non-significant at the 5% level (critical level = –0.47).
![]() |
Discussion
To fully understand the ecology of a species, many factors must be taken into consideration. Population connectivity is usually determined by methods that can track an individual’s life history throughout ontogeny, such as tagging (Gilly et al. 2006). However, these techniques are difficult to implement on loliginid squid, which are often too fragile for an external tag, have a short post-capture survival time and are lacking a suitable attachment site that does not inhibit their behaviour (Arkhipkin 2005). In this case, in order to obtain a complete picture of ontogenetic movements, a toolbox approach is required, where a range of independent techniques provides information at different temporal scales that can be used to understand connectivity (Sturrock et al. 2012). This can be achieved using methods such as elemental analysis of statoliths (Jones et al. 2018) or genetic methods (Shaw et al. 2004), but can also be achieved by analysing more basic information, such as size-at-age data. This study has made several steps in advancing our understanding of why superbull males exist within the D. gahi population using a toolbox approach.
The streamlined shape and large fins characteristic of the superbull morph are hypothesised to result in an extension of their migratory routes, providing a population-level benefit by connecting geographically separate subpopulations within Falkland Islands waters (Arkhipkin et al. 2015a; Jones et al. 2019). In addition to this connectivity between remote spawning areas, it is also possible that these squid provide temporal connectivity between spawning cohorts. At present, the mechanisms connecting the two cohorts are unresolved (Arkhipkin et al. 2013). Genetic studies using allozyme markers found no evidence of genetic differentiation among temporally distinct samples, suggesting that squid from both cohorts belong to a single interbreeding population (Carvalho and Pitcher 1989; Shaw et al. 2004). Several mechanisms have been proposed to allow for interbreeding between different seasonal cohorts, including early maturation and mating of males with mature females from the previous cohort, a wide variation in spawning time resulting in overlap or an extended life cycle of more than 1 year for some individuals (Mesnil 1977; Boyle et al. 1995; Arkhipkin et al. 2013). Therefore, superbulls were hypothesised to have a life cycle exceeding 1 year (including the period of embryogenesis), providing the population level benefit of temporal connectivity (Jones et al. 2019). In order for a population-level benefit to occur, these squid would have to be reproductively viable. On an individual level, an enhanced reproductive output compared with normal-sized individuals may be a selective advantage. Alternatively, the existence of superbulls may be a by-product of phenotypic plasticity, with no specific individual selective advantage. The selective advantage of plasticity would, of course, also occur at the population level, but selection is also arguably plausible if populations with (heritable) higher plasticity are more persistent over evolutionary time. This latter scenario is consistent with the fact that the occurrence of superbulls is rare (Jones et al. 2019).
In the present study there was no evidence of enhanced reproductive output in superbulls, but the data do suggest that superbull males are reproductively able to maintain connectivity, whether it is geographical or temporal. Results indicate some trade-off between somatic and reproductive growth in those exceeding 25 cm DML, with a reduction in TW relative to BW (TWI) and with the NCW increasing synonymously with BW. Although there is a trade-off, this should not inhibit the ability of these superbull males to reproduce with females on spawning grounds because NCW (responsible for spermatophore production and accumulation) is maintained. Most likely, the relative reduction in TW is due to increased weight of muscles in the mantle and fins to maintain a streamlined shape. Energy for production of reproductive organs appears to be derived from diet rather than a stored energy source, given the gradual decline of the DGI with size. An obvious drop would suggest that lipid deposits within the digestive gland are being used as an energy source at the onset of reproductive maturation (Guerra and Castro 1994; Moltschaniwskyj and Semmens 2000).
As shown in previous growth papers (Arkhipkin and Roa-Ureta 2005) and from the significant relationship between age and DML in the GAMM results in this study, there is a positive relationship between age and DML. Therefore, if a proportion of the population had an extended lifespan, it would be expected to be the largest animals, including superbull males, which are at the extreme positive end of the size distribution. In the present study, even after the inclusion of non-random samples of the largest individuals, maximum post-hatching age was estimated at 368 days, comparable to previous ageing studies (Hatfield 1991; Arkhipkin 1993). Results from the GLM suggest that the oldest individuals within the population are superbull males from the ASC, with a mean post-hatching age of 286 days. There is a large contrast in mean age between superbull males from the SSC and ASC and a larger variation in age for superbulls compared with the rest of the mature male population. Before hatching, embryo development is estimated at 51–65 days in austral summer (SSC) and 125 days in the winter (ASC; Arkhipkin and Middleton 2003). Inclusion of the long embryo development period suggests that superbulls from this particular cohort may have a life cycle exceeding 1 year. Mesnil (1977) proposed that squid hatched from eggs laid early in the breeding season are capable of reproduction and completion of their life cycle within 1 year. Those arising from the latter part of one season may not be reproductively competent in the next year, but live to breed at the start of the following breeding season (i.e. delayed maturation and an extended life cycle), and thus contribute towards connectivity between spawning groups. This hypothesis is consistent with the higher mean post-hatching ages of the superbull males from the ASC, which are likely providing temporal connectivity between spawning cohorts. Peak spawning for the ASC occurs from May to June, whereas for the SSC peak spawning occurs from October to November (Arkhipkin et al. 2007). The ASC extending their life cycle to breed with individuals from the SSC is far more likely because the difference in spawning time is smaller than if the SSC extended their life cycle to breed with individuals from the ASC (a 2- to 4-month gap rather than a 4- to 6-month gap). Sample collection dates of superbulls from each cohort further confirm this hypothesis. Peak collection for the SSC superbull samples was September–October. The SSC remain on their offshore feeding grounds until the end of October, when they rapidly migrate inshore to spawn. The ASC move inshore to spawn during March–May, with peak spawning during May–June (Arkhipkin et al. 2013). Collection of superbulls from the ASC peaked during both May and August, with superbull males from this cohort present in samples for several months of the year. The peak in collection during May coincides with the migration of the ASC to their spawning grounds, whereas the peak during August, well beyond the migration and spawning period for this cohort, provides further evidence that some individuals may have an extended life cycle. The wide variation in ages, wide distribution in hatching dates (also found in Shaw et al. 2004) and the availability of superbulls in samples during different times of the year suggest that there are several pathways to achieving this superbull morph, either through slow continuous growth and an extended life cycle or fast growth and a normal life-span, which provides evidence against heritability and instead favours the hypothesis that this morph arises through phenotypic variation.
Variability in size at maturity and adult size in cephalopods has largely been attributed to two main factors: temperature and food availability (Pecl et al. 2004). The response to extrinsic factors can be sex specific; a 5-year study on Sepioteuthis australis found that interannual differences in squid size, condition, reproductive investment and possibly growth rate were sex specific (Pecl et al. 2004). This is evident in the D. gahi population, which shows the most pronounced size and shape variability in the male population (Jones et al. 2019). The partial effects of temperature in Model 9 suggested that there was a significant linear negative relationship between SST during month of hatching and DML. This is in contrast with the findings of Hatfield (2000), who found that D. gahi hatched in months with high mean monthly SST were significantly larger than those hatched in winter months. A larger size-at-age for individuals hatched in warmer conditions is evident in field studies of other loliginid species (Moreno et al. 2007) and is consistent with the Forsythe hypothesis, which suggests that increased temperature during a squid’s early growth phase may result in accelerated growth and potentially a larger maximum size (Forsythe 2004). However, a negative relationship was found in the jumbo squid D. gigas (Arkhipkin et al. 2015b). Low temperatures during early ontogeny are associated with slow growth, delayed maturation and an extended life cycle, resulting in a large size. This pattern was also found when comparing broad spatial regions (equatorial, tropical and subtropical) for the loliginid squid S. lessoniana (Jackson and Moltschaniwskyj 2002). For D. gigas in Peruvian waters, low temperatures resulted in a large size and a life cycle that extended from 1 to 2 years (Arkhipkin et al. 2015b). In the present study, large-scale temperature variability found weak non-significant correlations between warm ENSO phases and the abundance of superbulls when lagged by 1 year. Although lower temperatures during month of hatching resulted in larger individuals, higher temperatures during other periods of ontogeny (i.e. juvenile and adult stages) could result in accelerated growth and perhaps a larger size.
The inclusion of CHL as a smoother improved model fit during the GAMM analysis, yet the effect of CHL was not as significant as the inclusion of SST, especially with a 1-month lag. The ‘Loligo box’, the fishing zone for the D. gahi trawl fishery, is occupied primarily by waters associated with the northward-flowing Falkland Current, consisting of sub-Antarctic superficial water in the upper layer and Antarctic intermediate water in the lower layer (Arkhipkin et al. 2004). Mixing of these waters with inshore waters results in high productivity in the summer and winter (Agnew 2002). The persistent macrozooplankton densities mean that D. gahi, which predominantly feeds on Euphausia spp. and the pelagic amphipod Themisto gaudichaudii (excluding self-cannibalism, which is most likely net feeding), is rarely food limited (Guerra et al. 1991; Brickle et al. 2001).
There appeared to be a significant spatial effect, with the largest individuals found in the ‘Loligo box’ for the optimal model, which included broad-scale spatial variability. However, for the model including a smoother of longitude and latitude, partial effects indicated that large individuals were located to the west of the Falkland Islands, which is more aligned with the raw data. Uneven sample distribution is a limitation in this study, with the majority of samples originating from the commercial fishing area (‘Loligo box’). Despite this discrepancy, a spatial effect is evident within both models, which are likely capturing residual unexplained environmental variation (such as upwelling effects or differences in temperature based on depth). The age–length relationship also differed significantly between areas. The ‘Loligo box’ in particular had discontinuity in the age–length relationship, which means either that growth accelerates after a certain size or that two different growth trajectories are present within the ‘Loligo box’ (potentially superbulls and normal individuals). Different growth trajectories for different morphs would be logical if observed differences were phenotypically driven.
In conclusion, the ecological role of superbulls within the D. gahi population is still not fully understood; however, superbulls from the ASC are likely to provide temporal connectivity between cohorts. Without evidence of enhanced swimming ability there is no direct way to confirm that these individuals provide geographic connectivity. There was no evidence of enhanced reproductive output in superbull males (which would confer a selective advantage), but they are still reproductively viable. Recently, spermatozoa from spermatophores of the loliginid squid L. reynaudii (Iwata et al. 2018) and Heterololigo bleekeri (Iwata et al. 2015) have been found to be dimorphic, associated with the two alternative ‘sneaker’ and ‘bull’ mating tactics. A useful future step to aid in understanding whether and how the reproductive status of superbull males differs relative to the rest of the male population would be to analyse the morphology of spermatozoa from mature males of different sizes. Although there may not be a reproductive advantage in terms of the relative weight of reproductive organs, sperm shape may be advantageous. Because temperature, area and, to a lesser extent, food availability have significant effects on the age–length relationship, it appears that the existence of these large superbulls can be attributed to phenotypic variability. Understanding why these superbull males exist may help us to discern how this commercially important species is connected on the Patagonian Shelf.
Conflicts of interest
The authors declare that they have no conflicts of interest.
Declaration of funding
This work was funded by the Falkland Islands Government. Thanks are due to FCT/MCTES for financial support to CESAM (UID/AMB/50017/2019) through national funds and ERDF co-financing, under the Partnership Agreement for the PT2020 and Compete 2020 programs.
Acknowledgements
This study was conducted using European Union Copernicus Marine Service information. The authors are grateful to the scientific observers from the Falkland Islands fisheries department for sample collection and to the Director of Fisheries, John Barton, for supporting this work.
References
Agnew, D. J. (2002). Critical aspects of the Falkland Islands pelagic ecosystem: distribution, spawning and migration of pelagic animals in relation to oil exploration. Aquatic Conservation 12, 39–50.| Critical aspects of the Falkland Islands pelagic ecosystem: distribution, spawning and migration of pelagic animals in relation to oil exploration.Crossref | GoogleScholarGoogle Scholar |
Akaike, H. (1973). Maximum likelihood identification of Gaussian autoregressive moving average models. Biometrika 60, 255–265.
| Maximum likelihood identification of Gaussian autoregressive moving average models.Crossref | GoogleScholarGoogle Scholar |
Arkhipkin, A. (1993). Statolith microstructure and maximum age of Loligo gahi (Myopsida: Loliginidae) on the Patagonian Shelf. Journal of the Marine Biological Association of the United Kingdom 73, 979–982.
| Statolith microstructure and maximum age of Loligo gahi (Myopsida: Loliginidae) on the Patagonian Shelf.Crossref | GoogleScholarGoogle Scholar |
Arkhipkin, A. I. (2005). Statoliths as ‘black boxes’ (life recorders) in squid. Marine and Freshwater Research 56, 573–583.
| Statoliths as ‘black boxes’ (life recorders) in squid.Crossref | GoogleScholarGoogle Scholar |
Arkhipkin, A. I., and Middleton, D. A. J. (2003). In-situ monitoring of the duration of embryonic development in the squid Loligo gahi (Cephalopoda: Loliginidae) on the Falkland shelf. The Journal of Molluscan Studies 69, 123–133.
| In-situ monitoring of the duration of embryonic development in the squid Loligo gahi (Cephalopoda: Loliginidae) on the Falkland shelf.Crossref | GoogleScholarGoogle Scholar |
Arkhipkin, A. I., and Roa-Ureta, R. (2005). Identification of ontogenetic growth models for squid. Marine and Freshwater Research 56, 371–386.
| Identification of ontogenetic growth models for squid.Crossref | GoogleScholarGoogle Scholar |
Arkhipkin, A. I., and Shcherbich, Z. N. (2012). Thirty years’ progress in age determination of squid using statoliths. Journal of the Marine Biological Association of the United Kingdom 92, 1389–1398.
| Thirty years’ progress in age determination of squid using statoliths.Crossref | GoogleScholarGoogle Scholar |
Arkhipkin, A. I., Grzebielec, R., Sirota, A. M., Remeslo, A. V., Polishchuk, I. A., and Middleton, D. A. (2004). The influence of seasonal environmental changes on ontogenetic migrations of the squid Loligo gahi on the Falkland shelf. Fisheries Oceanography 13, 1–9.
| The influence of seasonal environmental changes on ontogenetic migrations of the squid Loligo gahi on the Falkland shelf.Crossref | GoogleScholarGoogle Scholar |
Arkhipkin, A. I., Middleton, D. A., and Barton, J. (2007). Management and conservation of a short-lived fishery resource: Loligo gahi around the Falkland Islands. In ‘Reconciling Fisheries with Conservation: Proceedings of the Fourth World Fisheries Congress’, 2–6 May 2004, Vancouver, BC, Canada. (Eds J. Nielson, J. Dodson, K. Friedland, T. Hamon, J. Musick, and E. Verspoor.) pp. 587–596. (American Fisheries Society: Bethesda, MD, USA.)
Arkhipkin, A. I., Hatfield, E. M. C., and Rodhouse, P. G. (2013). Doryteuthis gahi, Patagonian long-finned squid. In ‘Advances in Squid Biology, Ecology and Fisheries. Part I’. (Eds R. Rosa, G. J. Pierce, and R. K. O’Dor.) pp. 123–157. (Nova Biomedical: New York, NY, USA.)
Arkhipkin, A. I., Weis, R., Mariotti, N., and Shcherbich, Z. (2015a). ‘Tailed’ cephalopods. The Journal of Molluscan Studies 81, 345–355.
| ‘Tailed’ cephalopods.Crossref | GoogleScholarGoogle Scholar |
Arkhipkin, A., Argüelles, J., Shcherbich, Z., and Yamashiro, C. (2015b). Ambient temperature influences adult size and life span in jumbo squid (Dosidicus gigas). Canadian Journal of Fisheries and Aquatic Sciences 72, 400–409.
| Ambient temperature influences adult size and life span in jumbo squid (Dosidicus gigas).Crossref | GoogleScholarGoogle Scholar |
Baylis, A. M., Zuur, A. F., Brickle, P., and Pistorius, P. A. (2012). Climate as a driver of population variability in breeding gentoo penguins Pygoscelis papua at the Falkland Islands. The Ibis 154, 30–41.
| Climate as a driver of population variability in breeding gentoo penguins Pygoscelis papua at the Falkland Islands.Crossref | GoogleScholarGoogle Scholar |
Box, G. E., and Cox, D. R. (1964). An analysis of transformations. Journal of the Royal Statistical Society – B. Statistical Methodology 26, 211–243.
| An analysis of transformations.Crossref | GoogleScholarGoogle Scholar |
Boyle, P. R., Pierce, G. J., and Hastie, L. C. (1995). Flexible reproductive strategies in the squid Loligo forbesi. Marine Biology 121, 501–508.
| Flexible reproductive strategies in the squid Loligo forbesi.Crossref | GoogleScholarGoogle Scholar |
Brickle, P., Olson, P. D., Littlewood, D. T. J., Bishop, A., and Arkhipkin, A. I. (2001). Parasites of Loligo gahi from waters off the Falkland Islands, with a phylogenetically based identification of their cestode larvae. Canadian Journal of Zoology 79, 2289–2296.
| Parasites of Loligo gahi from waters off the Falkland Islands, with a phylogenetically based identification of their cestode larvae.Crossref | GoogleScholarGoogle Scholar |
Carvalho, G. R., and Pitcher, T. J. (1989). Biochemical genetic studies on the Patagonian squid Loligo gahi D’Orbigny. II. Population structure in Falkland waters using isozymes, morphometrics and life history data. Journal of Experimental Marine Biology and Ecology 126, 243–258.
| Biochemical genetic studies on the Patagonian squid Loligo gahi D’Orbigny. II. Population structure in Falkland waters using isozymes, morphometrics and life history data.Crossref | GoogleScholarGoogle Scholar |
Collins, M. A., Boyle, P. R., Pierce, G. J., Key, L. N., Hughes, S. E., and Murphy, J. (1999). Resolution of multiple cohorts in the Loligo forbesi population from the west of Scotland. ICES Journal of Marine Science 56, 500–509.
| Resolution of multiple cohorts in the Loligo forbesi population from the west of Scotland.Crossref | GoogleScholarGoogle Scholar |
Falkland Islands Government (2017). Fisheries Department fisheries statistics. Technical Report 21, Falkland Islands Fisheries Department, Stanley, Falkland Islands.
Forsythe, J. W. (2004). Accounting for the effect of temperature on squid growth in nature: from hypothesis to practice. Marine and Freshwater Research 55, 331–339.
| Accounting for the effect of temperature on squid growth in nature: from hypothesis to practice.Crossref | GoogleScholarGoogle Scholar |
Fox, J. (2003). Effect displays in R for generalised linear models. Journal of Statistical Software 8, 1–27.
| Effect displays in R for generalised linear models.Crossref | GoogleScholarGoogle Scholar |
Gilly, W. F., Markaida, U., Baxter, C. H., Block, B. A., Boustany, A., Zeidberg, L., Reisenbichler, K., Robison, B., Bazzino, G., and Salinas, C. (2006). Vertical and horizontal migrations by the jumbo squid Dosidicus gigas revealed by electronic tagging. Marine Ecology Progress Series 324, 1–17.
| Vertical and horizontal migrations by the jumbo squid Dosidicus gigas revealed by electronic tagging.Crossref | GoogleScholarGoogle Scholar |
Guerra, A., and Castro, B. G. (1994). Reproductive–somatic relationships in Loligo gahi (Cephalopoda: Loliginidae) from the Falkland Islands. Antarctic Science 6, 175–178.
| Reproductive–somatic relationships in Loligo gahi (Cephalopoda: Loliginidae) from the Falkland Islands.Crossref | GoogleScholarGoogle Scholar |
Guerra, A., Castro, B. G., and Nixon, M. (1991). Preliminary study on the feeding by Loligo gahi (Cephalopoda: Loliginidae). Bulletin of Marine Science 49, 309–311.
Hall, K. C., and Hanlon, R. T. (2002). Principal features of the mating system of a large spawning aggregation of the giant Australian cuttlefish Sepia apama (Mollusca: Cephalopoda). Marine Biology 140, 533–545.
| Principal features of the mating system of a large spawning aggregation of the giant Australian cuttlefish Sepia apama (Mollusca: Cephalopoda).Crossref | GoogleScholarGoogle Scholar |
Hanlon, R. T., and Messenger, J. B. (1998). ‘Cephalopod Behaviour’. (Cambridge University Press: Cambridge, UK.)
Hanlon, R. T., Smale, M. J., and Sauer, W. H. (2002). The mating system of the squid Loligo vulgaris reynaudii (Cephalopoda, Mollusca) off South Africa: fighting, guarding, sneaking, mating and egg laying behavior. Bulletin of Marine Science 71, 331–345.
Hatfield, E. M. C. (1991). Post-recruit growth of the Patagonian squid Loligo gahi (D’Orbigny). Bulletin of Marine Science 49, 349–361.
Hatfield, E. M. C. (2000). Do some like it hot? Temperature as a possible determinant of variability in the growth of the Patagonian squid, Loligo gahi (Cephalopoda: Loliginidae). Fisheries Research 47, 27–40.
| Do some like it hot? Temperature as a possible determinant of variability in the growth of the Patagonian squid, Loligo gahi (Cephalopoda: Loliginidae).Crossref | GoogleScholarGoogle Scholar |
Hatfield, E. M. C., Rodhouse, P. G., and Porebski, J. (1990). Demography and distribution of the Patagonian squid (Loligo gahi d’Orbigny) during the austral winter. ICES Journal of Marine Science 46, 306–312.
| Demography and distribution of the Patagonian squid (Loligo gahi d’Orbigny) during the austral winter.Crossref | GoogleScholarGoogle Scholar |
Iwata, Y., Sakurai, Y., and Shaw, P. (2015). Dimorphic sperm-transfer strategies and alternative mating tactics in loliginid squid. The Journal of Molluscan Studies 81, 147–151.
| Dimorphic sperm-transfer strategies and alternative mating tactics in loliginid squid.Crossref | GoogleScholarGoogle Scholar |
Iwata, Y., Sauer, W. H. H., Sato, N., and Shaw, P. (2018). Spermatophore dimorphism in the chokka squid Loligo reynaudii associated with alternative mating tactics. The Journal of Molluscan Studies 84, 157–162.
| Spermatophore dimorphism in the chokka squid Loligo reynaudii associated with alternative mating tactics.Crossref | GoogleScholarGoogle Scholar |
Jackson, G., and Forsythe, J. (2002). Statolith age validation and growth of Loligo plei (Cephalopoda: Loliginidae) in the north-west Gulf of Mexico during spring/summer. Journal of the Marine Biological Association of the United Kingdom 82, 677–678.
| Statolith age validation and growth of Loligo plei (Cephalopoda: Loliginidae) in the north-west Gulf of Mexico during spring/summer.Crossref | GoogleScholarGoogle Scholar |
Jackson, G., and Moltschaniwskyj, N. (2002). Spatial and temporal variation in growth rates and maturity in the Indo-Pacific squid Sepioteuthis lessoniana (Cephalopoda: Loliginidae). Marine Biology 140, 747–754.
| Spatial and temporal variation in growth rates and maturity in the Indo-Pacific squid Sepioteuthis lessoniana (Cephalopoda: Loliginidae).Crossref | GoogleScholarGoogle Scholar |
Jackson, G., Arkhipkin, A. I., Bizikov, V., and Hanlon, R. (1993). Laboratory and field corroboration of age and growth from statoliths and gladii of the loliginid squid Sepioteuthis lessoniana (Mollusca: Cephalopoda). In ‘Recent Advances in Cephalopod Fisheries Biology’. (Eds T. Okutani, R. K. O’Dor, and T. Kubodera.) pp. 189–199. (Tokai University Press: Tokyo, Japan.)
Jackson, G. D., Forsythe, J. W., Hixon, R. F., and Hanlon, R. T. (1997). Age, growth, and maturation of Lolliguncula brevis (Cephalopoda: Loliginidae) in the northwestern Gulf of Mexico with a comparison of length-frequency versus statolith age analysis. Canadian Journal of Fisheries and Aquatic Sciences 54, 2907–2919.
| Age, growth, and maturation of Lolliguncula brevis (Cephalopoda: Loliginidae) in the northwestern Gulf of Mexico with a comparison of length-frequency versus statolith age analysis.Crossref | GoogleScholarGoogle Scholar |
Jones, J. B., Arkhipkin, A. I., Marriott, A. L., and Pierce, G. J. (2018). Using statolith elemental signatures to confirm ontogenetic migrations of the squid Doryteuthis gahi around the Falkland Islands (Southwest Atlantic). Chemical Geology 481, 85–94.
| Using statolith elemental signatures to confirm ontogenetic migrations of the squid Doryteuthis gahi around the Falkland Islands (Southwest Atlantic).Crossref | GoogleScholarGoogle Scholar |
Jones, J. B., Pierce, G. J., Saborido-Rey, F., Brickle, P., Kuepper, F. C., Shcherbich, Z. N., and Arkhipkin, A. I. (2019). Size-dependent change in body shape and its possible ecological role in the Patagonian squid (Doryteuthis gahi) in the Southwest Atlantic. Marine Biology 166, 54.
| Size-dependent change in body shape and its possible ecological role in the Patagonian squid (Doryteuthis gahi) in the Southwest Atlantic.Crossref | GoogleScholarGoogle Scholar |
Lipinski, M. (1979). Universal maturity scale for the commercially important squids. The results of maturity classification of the Illex illecebrosus population for the years 1973–1977. ICNAF Research Document 79/II/38. (International Commission of the Northwest Atlantic Fisheries.) Available at https://www.nafo.int/icnaf/library/docs/1979/res-38.pdf [Verified 4 March 2019].
Lipinski, M., Durholtz, M., and Underhill, L. (1998). Field validation of age readings from the statoliths of chokka squid (Loligo vulgaris reynaudii D’Orbigny, 1845) and an assessment of associated errors. ICES Journal of Marine Science 55, 240–257.
| Field validation of age readings from the statoliths of chokka squid (Loligo vulgaris reynaudii D’Orbigny, 1845) and an assessment of associated errors.Crossref | GoogleScholarGoogle Scholar |
Mangold, K. (1987). Reproduction. In ‘Cephalopod Life Cycles. Vol. II’. (Ed. P. R. Boyle.) pp. 157–200. (Academic Press: London, UK.)
Meredith, M. P., Murphy, E. J., Hawker, E. J., King, J. C., and Wallace, M. I. (2008). On the interannual variability of ocean temperatures around South Georgia, Southern Ocean: forcing by El Niño–Southern Oscillation and the southern annular mode. Deep-sea Research – II. Topical Studies in Oceanography 55, 2007–2022.
| On the interannual variability of ocean temperatures around South Georgia, Southern Ocean: forcing by El Niño–Southern Oscillation and the southern annular mode.Crossref | GoogleScholarGoogle Scholar |
Mesnil, B. (1977). Growth and life cycle of squid, Loligo pealei and Illex illecebrosus, from the northwest Atlantic. International Commission for the Northwest Atlantic Fisheries Selected Papers 2, 55–69.
Moltschaniwskyj, N. A., and Semmens, J. M. (2000). Limited use of stored energy reserves for reproduction by the tropical loliginid squid Photololigo sp. Journal of Zoology 251, 307–313.
| Limited use of stored energy reserves for reproduction by the tropical loliginid squid Photololigo sp.Crossref | GoogleScholarGoogle Scholar |
Moreno, A., Azevedo, M., Pereira, J., and Pierce, G. J. (2007). Growth strategies in the squid Loligo vulgaris from Portuguese waters. Marine Biology Research 3, 49–59.
| Growth strategies in the squid Loligo vulgaris from Portuguese waters.Crossref | GoogleScholarGoogle Scholar |
O’Dor, R. (1998). Can understanding squid life-history strategies and recruitment improve management? South African Journal of Marine Science 20, 193–206.
| Can understanding squid life-history strategies and recruitment improve management?Crossref | GoogleScholarGoogle Scholar |
Patterson, K. R. (1988). Life history of Patagonian squid Loligo gahi and growth parameter estimates using least-squares fits to linear and von Bertalanffy models. Marine Ecology Progress Series 47, 65–74.
| Life history of Patagonian squid Loligo gahi and growth parameter estimates using least-squares fits to linear and von Bertalanffy models.Crossref | GoogleScholarGoogle Scholar |
Pecl, G. T., Moltschaniwskyj, N. A., Tracey, S. R., and Jordan, A. R. (2004). Inter-annual plasticity of squid life history and population structure: ecological and management implications. Oceologica 139, 515–524.
| Inter-annual plasticity of squid life history and population structure: ecological and management implications.Crossref | GoogleScholarGoogle Scholar |
Pierce, G. J., and Guerra, A. (1994). Stock assessment methods used for cephalopod fisheries. Fisheries Research 21, 255–285.
| Stock assessment methods used for cephalopod fisheries.Crossref | GoogleScholarGoogle Scholar |
Pierce, G. J., Sauer, W., Allcock, A. L., Smith, J. M., Wangvoralak, S., Jereb, P., Hastie, L. C., and Lefkaditou, E. (2013). Loligo forbesii, veined squid. In ‘Advances in Squid Biology, Ecology and Fisheries. Part I’. (Eds R. Rosa, G. J. Pierce, and R. K. O’Dor.) pp. 73–108. (Nova Biomedical: New York, NY, USA.)
Reynolds, R. W., Rayner, N. A., Smith, T. M., Stokes, D. C., and Wang, W. (2002). An improved in situ and satellite SST analysis for climate. Journal of Climate 15, 1609–1625.
| An improved in situ and satellite SST analysis for climate.Crossref | GoogleScholarGoogle Scholar |
Shashar, N., and Hanlon, R. T. (2013). Spawning behavior dynamics at communal egg beds in the squid Doryteuthis (Loligo) pealeii. Journal of Experimental Marine Biology and Ecology 447, 65–74.
| Spawning behavior dynamics at communal egg beds in the squid Doryteuthis (Loligo) pealeii.Crossref | GoogleScholarGoogle Scholar |
Shaw, P. W., Arkhipkin, A. I., Adcock, G. J., Burnett, W. J., Carvalho, G. R., Scherbich, J. N., and Villegas, P. A. (2004). DNA markers indicate that distinct spawning cohorts and aggregations of Patagonian squid, Loligo gahi, do not represent genetically discrete subpopulations. Marine Biology 144, 961–970.
| DNA markers indicate that distinct spawning cohorts and aggregations of Patagonian squid, Loligo gahi, do not represent genetically discrete subpopulations.Crossref | GoogleScholarGoogle Scholar |
Sturrock, A. M., Trueman, C. N., Darnaude, A. M., and Hunter, E. (2012). Can otolith elemental chemistry retrospectively track migrations in fully marine fishes? Journal of Fish Biology 81, 766–795.
| Can otolith elemental chemistry retrospectively track migrations in fully marine fishes?Crossref | GoogleScholarGoogle Scholar | 22803735PubMed |
Trathan, P. N., and Murphy, E. J. (2003). Sea surface temperature anomalies near South Georgia: relationships with the Pacific El Niño regions. Journal of Geophysical Research – C. Oceans 108, 8075.
| Sea surface temperature anomalies near South Georgia: relationships with the Pacific El Niño regions.Crossref | GoogleScholarGoogle Scholar |
Villanueva, R. (2000). Differential increment-deposition rate in embryonic statoliths of the loliginid squid Loligo vulgaris. Marine Biology 137, 161–168.
| Differential increment-deposition rate in embryonic statoliths of the loliginid squid Loligo vulgaris.Crossref | GoogleScholarGoogle Scholar |
Wada, T., Takegaki, T., Mori, T., and Natsukari, Y. (2005). Alternative male mating behaviors dependent on relative body size in captive oval squid Sepioteuthis lessoniana (Cephalopoda, Loliginidae). Zoological Science 22, 645–651.
| Alternative male mating behaviors dependent on relative body size in captive oval squid Sepioteuthis lessoniana (Cephalopoda, Loliginidae).Crossref | GoogleScholarGoogle Scholar | 15988158PubMed |
Wagenmakers, E. J., and Farrell, S. (2004). AIC model selection using Akaike weights. Psychonomic Bulletin & Review 11, 192–196.
| AIC model selection using Akaike weights.Crossref | GoogleScholarGoogle Scholar |
Waluda, C. M., Trathan, P. N., and Rodhouse, P. G. (2004). Synchronicity in southern hemisphere squid stocks and the influence of the Southern Oscillation and Trans Polar Index. Fisheries Oceanography 13, 255–266.
| Synchronicity in southern hemisphere squid stocks and the influence of the Southern Oscillation and Trans Polar Index.Crossref | GoogleScholarGoogle Scholar |
Wood, S. N. (2003). Thin-plate regression splines. Journal of the Royal Statistical Society – B. Statistical Methodology 65, 95–114.
| Thin-plate regression splines.Crossref | GoogleScholarGoogle Scholar |
Wood, S. N. (2008). Fast stable direct fitting and smoothness selection for generalized additive models. Journal of the Royal Statistical Society – B. Statistical Methodology 70, 495–518.
| Fast stable direct fitting and smoothness selection for generalized additive models.Crossref | GoogleScholarGoogle Scholar |
Wood, S. N. (2011). Fast stable restricted maximum likelihood and marginal likelihood estimation of semiparametric generalized linear models. Journal of the Royal Statistical Society – B. Statistical Methodology 73, 3–36.
| Fast stable restricted maximum likelihood and marginal likelihood estimation of semiparametric generalized linear models.Crossref | GoogleScholarGoogle Scholar |
Zuur, A. F., and Ieno, E. N. (2016). A protocol for conducting and presenting results of regression‐type analyses. Methods in Ecology and Evolution 7, 636–645.
| A protocol for conducting and presenting results of regression‐type analyses.Crossref | GoogleScholarGoogle Scholar |
Zuur, A. F., Ieno, E. N., and Elphick, C. S. (2010). A protocol for data exploration to avoid common statistical problems. Methods in Ecology and Evolution 1, 3–14.
| A protocol for data exploration to avoid common statistical problems.Crossref | GoogleScholarGoogle Scholar |