A step-by-step guide to ageing octopus
Erica D. Durante


A
B
C
Handling Editor: Max Finlayson
Abstract
Global octopus catch has doubled over the past four decades and is likely to grow in importance as many key fisheries continue to decline. Lack of age data is a critical limitation in assessing the resource status of octopus. Over the past ~30 years, studies have investigated various methods to age octopus, with some methods better suited to certain species than others. However, only a small number of researchers have the hands-on knowledge to execute these methods in the laboratory. Here, we present the first step-by-step guide to ageing octopus, as well as a decision tool, which should enable readers to carry out the ageing process and make an informed decision on the most suitable method for their species. We provide guidance on age validation, increment analysis of both beaks and stylets, materials needed, as well as avenues for further research. We hope this guide will provide a starting point for researchers new to octopus ageing, and for those working with octopus species that have never been aged before. We also encourage researchers to use this guide as a forum for open discussion to support the ongoing development of effective octopus ageing methods.
Keywords: age validation, beaks, cephalopods, fisheries, increment analysis, methods, octopus ageing, stylets.
Introduction
Fisheries are fundamental to the maintenance of global food security and contribute to the livelihoods of an estimated 600 million people (Food and Agriculture Organization of the United Nations 2022). However, decades of overfishing have resulted in the depletion of some finfish stocks (Food and Agriculture Organization of the United Nations 2022). Simultaneously, some cephalopod populations have proliferated and octopus fisheries have expanded, which may be, in part, due to diminished finfish supply (Caddy and Rodhouse 1998; Balguerías et al. 2000; Doubleday et al. 2016; Sauer et al. 2021). Octopus fisheries are expected to expand further as humanity strives to effectively meet the nutritional demands of a rising global population (Rodhouse et al. 2014; Sauer et al. 2021). However, many commercially harvested octopus species remain critically understudied and the potential impact of fishing on these populations is poorly understood (Martino et al. 2021; Sauer et al. 2021). Long-term maintenance of these fisheries will rely on sustainable management practices supported by a robust understanding of life history and population dynamics, such as maturation, mortality and recruitment, to which age and growth data are essential (Rodhouse et al. 2014).
A range of methods have been explored to estimate octopus age, including direct enumeration of growth increments in hard structures such as stylets (Doubleday et al. 2006) and beaks (Perales-Raya and Hernández-González 1998; Perales-Raya et al. 2010), and indirect methods that act as an age proxy, such as eye lens diameter or weight (Baqueiro-Cárdenas et al. 2011), stylet weight (Leporati and Hart 2015), and lipofuscin quantification (Doubleday and Semmens 2011). Stylet and beak increment analyses remain the most effective and broadly used octopus ageing methods and have been validated across different life stages for multiple species (Doubleday et al. 2006; Hermosilla et al. 2010; Rodríguez-Domínguez et al. 2013; Bárcenas et al. 2014; Perales-Raya et al. 2014a). However, because of species-specific variations in beak and stylet microstructure, not all preparation techniques can be applied to all species. Therefore, a period of method development that includes increment visualisation and validation of increment periodicity is usually required when ageing a species for the first time.
The following guide outlines common and successfully applied methods for stylet and beak preparation, increment analyses, and age validation, as well as guidance on selecting the most suitable method for different octopus species. Although we acknowledge that ageing methods will continue to evolve, we hope this guide will provide a starting point for researchers new to octopus ageing, and for those working with octopus species that have never been aged before.
Part 1: stylet increment analysis
Stylets are cartilaginous vestigial internal shells consisting of a pair of thin rod-like structures embedded within the muscle behind the two brachial hearts on either side of the mantle (Bizikov 2004). As octopus grow, the stylet is formed in layers and increments are periodically deposited, thus facilitating age estimation through increment analysis. Stylet increments were first discovered by Sousa-Reis and Fernandes (2002) and then validated as an ageing method by Doubleday et al. (2006) in which transverse sections were taken, embedded in Crystalbond 509, and polished. However, as stylets are sensitive to heat and drying out, Barratt and Allcock (2010) created a method for permanent stylet preparation using a low-viscosity resin. In both methods, growth increments are visualised under microscopy and counted through micrographs taken of the section. Thus far, these methods have been used for a variety of species including, but not limited to, Octopus pallidus (Doubleday et al. 2006), Octopus vulgaris (Hermosilla et al. 2010), Octopus maya (Rodríguez-Domínguez et al. 2013) and Octopus huttoni (Donlon et al. 2019). However, stylet shape, consistency and increment readability vary between species. Thus, stylet increment analysis may not be suitable for all species.
Dissection and storage
Stylets are embedded within the mantle musculature where the mantle abductor muscles attach to the mantle (Fig. 1), and can be dissected through the following method (Fig. 2):
Begin from the ventral side of the octopus.
Adjacent to the muscular septum, make a vertical incision from the base (anterior) to the top (posterior) of the mantle.
Make a horizontal incision through the muscular septum.
Peel back the ventral mantle wall to separate from the visceral sac and gill, and on one side, locate the stylet at the base of the abductor muscle and branchial heart (Fig. 3).
Make an incision into the mantle muscle where the mantle abductor muscle and stylet adjoin as close to the stylet elbow as possible.
Carefully remove the stylet from the mantle and preserve in 70% ethanol until ready for use.
Repeat steps 4–6 to retrieve the stylet on the opposite side.
Stylet dissection process involving (a) a vertical incision from the base (anterior) to the top (posterior) of the mantle, (b) a horizontal incision through the muscular septum, (c) stylet location, (d) an incision to separate the stylet and mantle abductor muscle, and (e) removal of the stylet from the mantle.
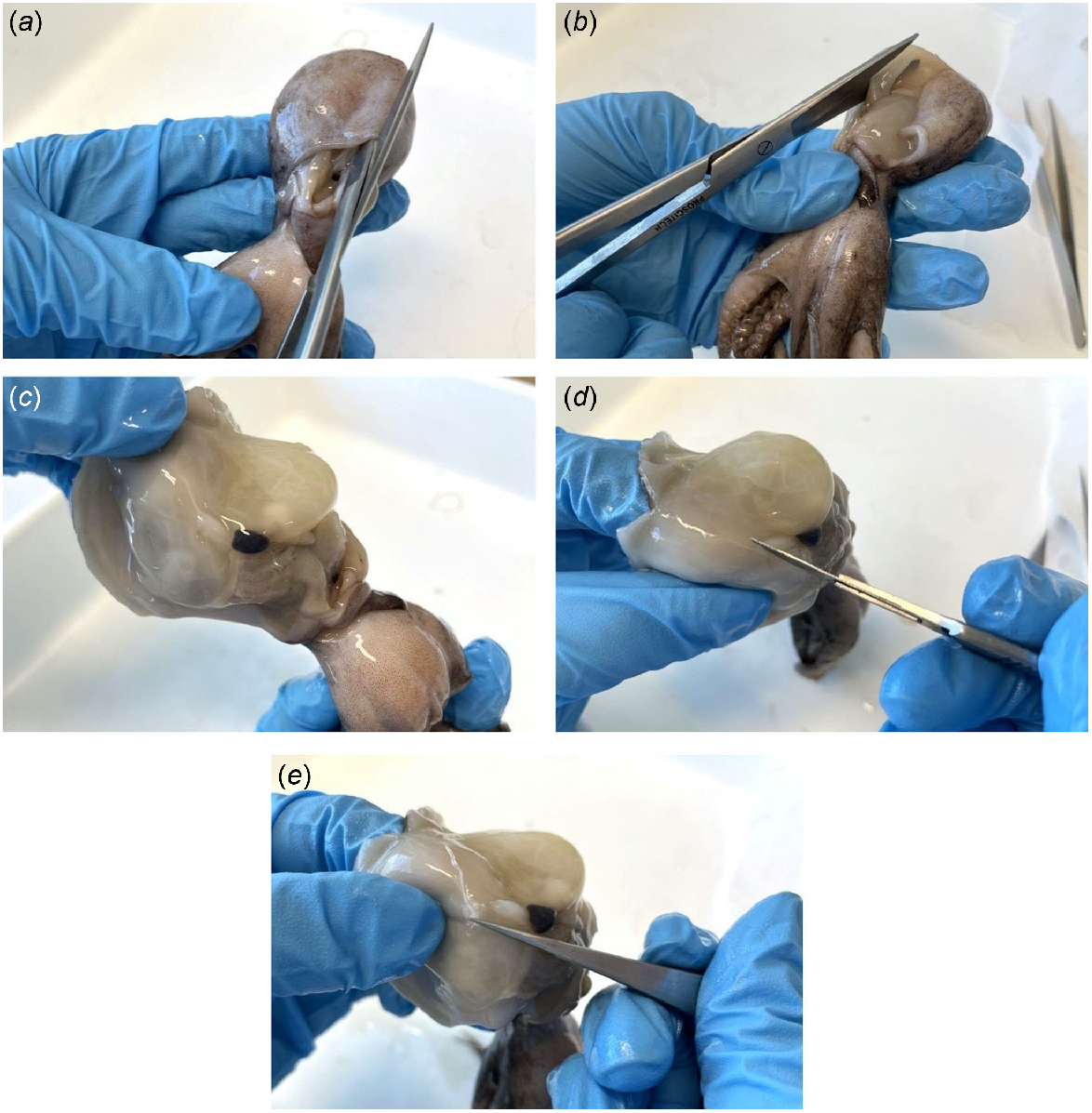
Preparing stylets from adults and large individuals
A permanent stylet preservation method developed by Barratt and Allcock (2010) using a low-viscosity resin has been utilised in multiple octopus ageing studies (Barratt and Allcock 2010; Leporati and Hart 2015; Durante et al. 2023). In these studies, LR White resin was chosen as it can be cold cured to prevent the exothermic reaction that often damages stylet sections. The resin infiltration process using LR White resin can be undertaken through the following method:
Using a single-sided razor blade, transversely section the post-rostral zone of the stylet (region of increment analysis in Fig. 4) into ~1-mm lengths, preparing up to three lengths for each stylet.
Prepare three tubes (with lids) per sample following the solutions outlined in Table 1.
Dehydrate and impregnant the stylets lengths following Table 1, making sure to blot excess solution from each length using a tissue before placing in the next solution. It is especially important to ensure all excess ethanol is removed before placing in the resin for 24 h. Solutions can be reused up to three times, although ideally they should be changed after each sample as solutions can be diluted over time as ethanol evaporates and some may mix in the resin solution.
Mount stylet lengths vertically (cut side down) onto a glass base with double-sided tape. Any double-sided tape is suitable as long as it has enough stick.
Place cylindrical moulds over the top of each group of lengths on the tape (Fig. 5). Here, we have cut the bottoms from 5-mL plastic sample tubes and used the tops cut-side up. However, any shape mould is suitable. In our experience, silicone moulds do not work, and hard plastic (polyethylene) moulds are best. Be sure to clean tubes with ethanol and wipe down after each use to ensure they adhere to the tape.
Mix a new aliquot of catalysed resin with accelerator (5 mL of resin per 1 drop of accelerator) in a disposable cup or jar and mix well by pipetting up and down with a disposable pipette. Prepare enough to cover all stylet pieces.
Carefully pipette resin mixture into the mould until stylet lengths are covered. Transfer to a fridge and leave to set for at least 2 h.
Remove the resin block from its mould and wipe away excess resin with paper towel.
Remove any sticky residue from the tape by carefully scraping with a razor blade, ensuring not to cut any resin. The idea is to form a smooth, flat surface for polishing. For stubborn residue, surface-safe adhesive removers may be useful.
Using wet 1000 grit sandpaper, followed by 15-, 6- and 3-µm lapping film, sand and buff the bottom of the block until the stylet end is visible. Regular checks under a microscope will help visualise progress. The surface should be as flat as possible. Using a slab of glass as the working surface under the sandpaper and lapping film is best, but a motorised turntable would also work.
Using clear Gorilla glue, affix the block polished-side down to a clean microscope slide and leave to fully dry for 24 h (Fig. 6a). In our experience, superglue is not adequate as it is not waterproof and degrades during polishing; therefore, water-resistant glue is best.
Using a cutting device such as a diamond saw, remove excess resin to make 100–200-μm-thick sections. Alternatively, a motorised turntable or rotary tool (e.g. Dremel) with sandpaper may be useful. It is important to make the surface as evenly flat as possible, which is more difficult with a handheld Dremel.
Grind and polish the remaining resin block using wet 1000 grit sandpaper followed by 15-, 6- and 3-µm lapping film until a thin section of the stylet is visible (Fig. 6b). Extra scratches can then be buffed out with 0.5 μm of aluminium oxide powder and a carwash chamois or any smooth, soft cloth.
Image showing the region of increment analysis on the post-rostral zone of an Octopus pallidus stylet.
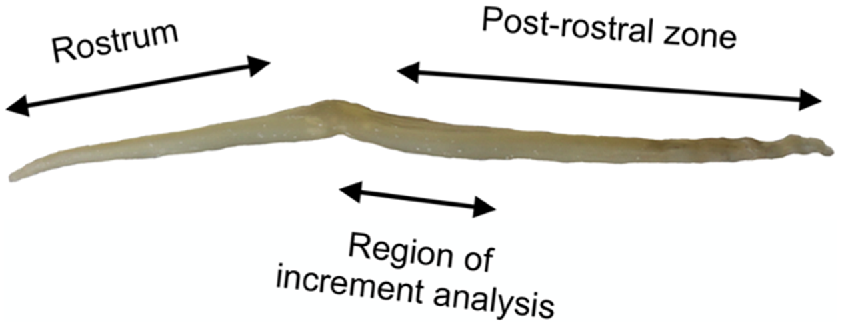
Solution | Duration (h) | |
---|---|---|
90% ethanol | 1 | |
100% ethanol | 1 | |
LR white resin (medium grade) | 24 |
The LR white resin solution was catalysed prior to adding accelerator (hardener).
Preparing stylets from hatchlings and juveniles
If stylets can be readily dissected and removed from a juvenile or hatchling, they can be prepared as described above, but it should be noted that often increments in small stylets are difficult to read because of the loss of resolution at high magnifications. If stylets cannot be removed from very young hatchlings, they may be identified using histological methods, but again increment visualisation may be impossible.
Visualising and counting growth increments
Stylet growth increments can be visualised using transmitted brightfield microscopy and either counted directly through the eyepiece while under the microscope, from an enlarged digital image on an attached computer screen, or from a single or series of saved digital images using an image analysis software application such as ImageJ (Fig. 7). The best viewing magnification will vary for each octopus species, although resolution is often lost at higher magnifications. For example, for Octopus berrima, stylet increments were best viewed between 200 and 400× magnification (Durante et al. 2023) and for Robsonella huttoni (Octopus huttoni), increments were best viewed between 400 and 1000× (oil immersion) magnification (Donlon et al. 2019).
Micrograph of Macroctopus maorum stylet section showing growth increments at 100× magnification.
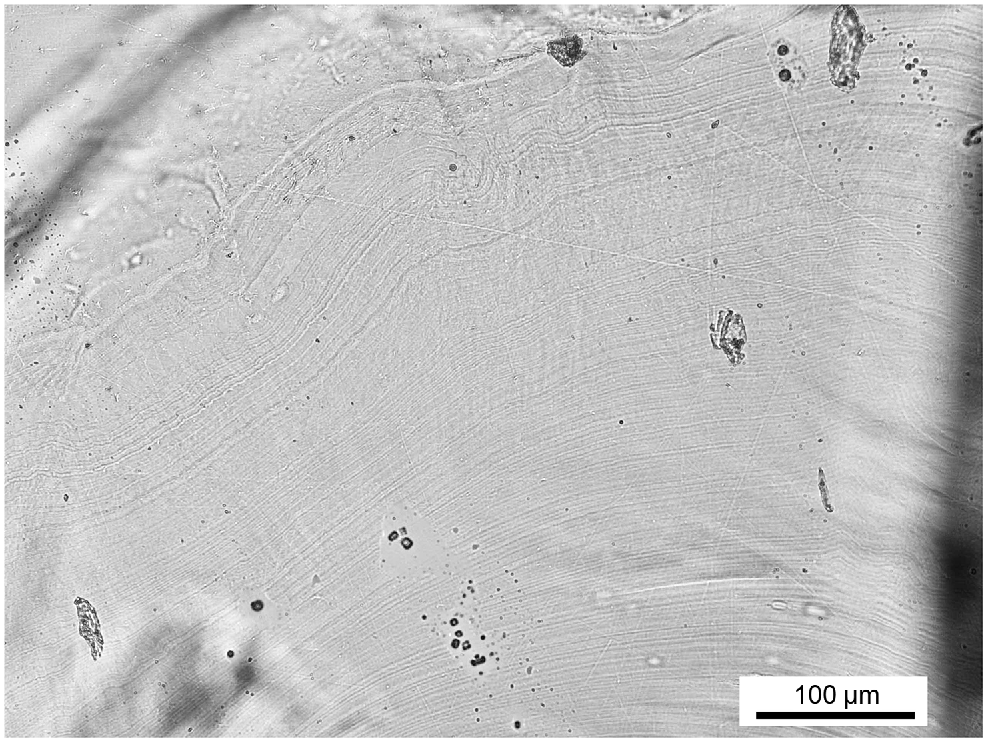
Ideally, increments, from the core to the edge, should be counted at least twice, non-consecutively, by one or more trained readers, with the average of multiple counts used to define age (if increment periodicity is known). Ageing precision is typically measured by taking the percentage difference between counts. Then, if the counts differ by more than a set percentage for a single stylet section (i.e. >10% is a typical standard), the section is discarded (Barratt and Allcock 2010; Perales-Raya et al. 2010; Leporati and Hart 2015). We refrain here from recommending a set number of consecutive counts, number of readers and percentage cut off for precision, because these may need to vary based on species, number of samples available and application. However, we suggest that practitioners refer to published methods, particularly if their species has been aged before.
Determining age using stylet weight
Once increment periodicity is validated and stylet increment analysis undertaken, there is potential to take the ageing method further by determining if stylet weight (or another morphometric measure) can be used as a proxy for age. For example, Leporati and Hart (2015) found that there was a strong relationship between age and stylet weight in Octopus djinda (formally Octopus cf. tetricus), suggesting that stylet weight can be used as a rapid, cost-effective and reliable ageing method.
Part 2: beak increment analysis
Beaks are composed of a mixture of chitin and protein and embedded within the buccal mass (mouth musculature) located at the centre of the arms on the ventral side of the octopus (Bizikov 2004). As octopus grow, beak increments are periodically deposited on the edge of the rostrum and lateral wall, thus facilitating age determination through increment analysis. Beak increment analysis was first explored in octopus by Perales-Raya and Hernández-González (1998) and can be prepared through a range of methodologies such as the rostrum sagittal section (RSS), lateral wall surface (LWS) or lateral rostrum surface (LRS) (Arkhipkin et al. 2018). Of these methods, the LWS appears to be a more accurate age indicator than the RSS (Perales-Raya et al. 2014a), but the most recent suggestion is to analyse both LWS and RSS of upper and lower beaks of new species to determine the best reading location (Xavier et al. 2022). The LRS is typically only used on hatchling, paralarvae or translucent adult beaks in which increments are only visible in this area (Perales-Raya et al. 2014a, 2018; Franco-Santos et al. 2016; Arkhipkin et al. 2018).
We provide a detailed outline of the steps involved for beak increment analyses using the LWS. For methods using the RSS and LRS, refer to Perales-Raya et al. (2010, 2014a, 2018) and Franco-Santos et al. (2016).
Dissection and storage
Octopus beaks are embedded within the buccal mass on the ventral side of the octopus (Fig. 8). Dissection can be undertaken through the following method (Fig. 9) and is best performed after the octopus or entire buccal mass has been previously frozen:
Begin on the ventral side of the octopus between the arms.
Make an incision to both sides of the mouth musculature to expose the beak.
Using tweezers, carefully remove the upper and lower beak.
Diagram of an octopus from a ventral viewpoint, indicating the beak embedded within the buccal mass.
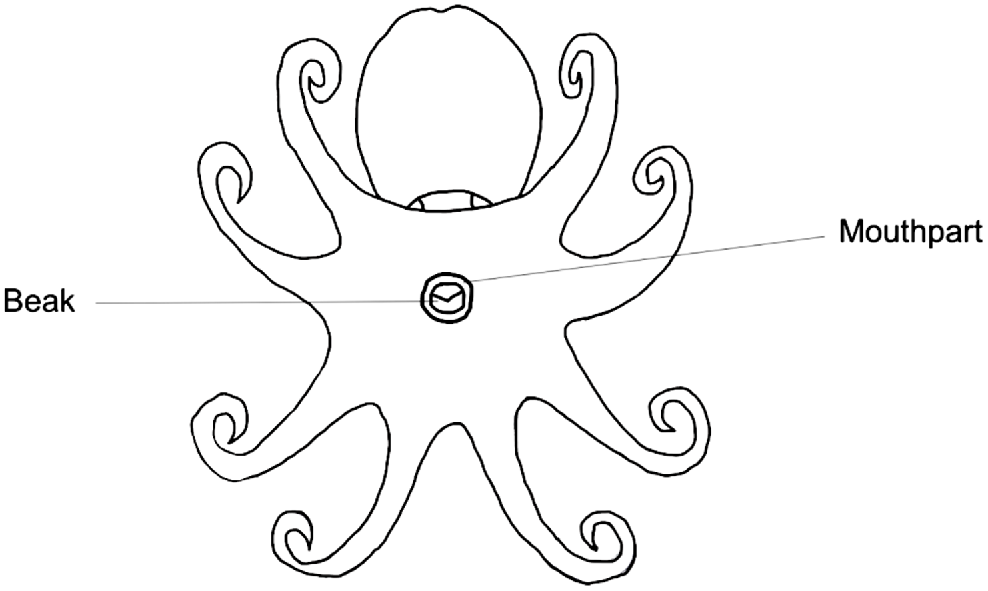
Beak dissection process involving (a) an incision to one side of the mouth musculature to expose the beak, (b) another incision to the other side, and (c) removal of the beak from the buccal mass.
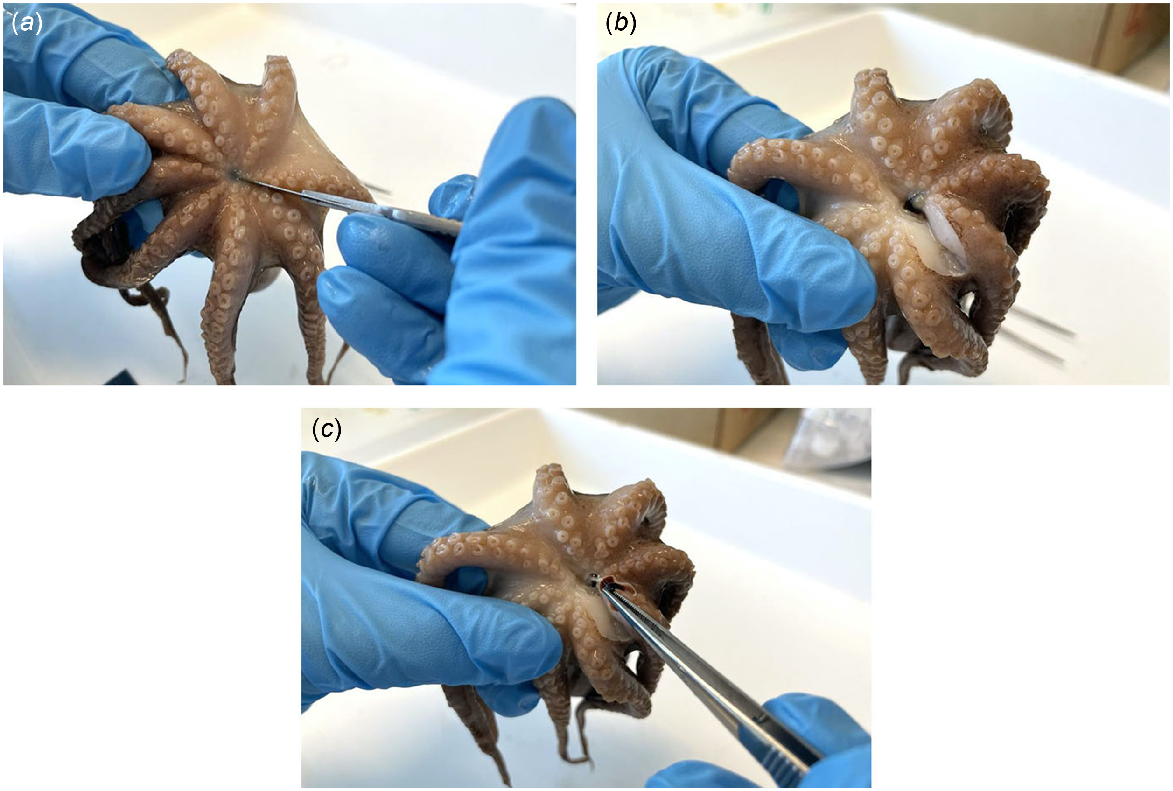
After the majority of tissue is cleaned, beaks can be preserved indefinitely in 70% ethanol until ready for use or, if analysis occurs shortly after, they can be preserved in distilled water at 4°C. The latter preservation method has been found to better preserve the microstructure, but trials should always be done for each species to determine whether ethanol significantly degrades the microstructure or not.
Preparing the LWS of beaks from adults and large individuals
Using scissors, cut the upper beak in half to obtain two sagittal sections (Fig. 10). Select the flattest half for sample preparation.
Remove any remaining tissue from the beak using distilled water and scrub gently with the tip of a plastic pipette. For stubborn tissue, place beak halves in a tube with 5% hydrogen peroxide in an ultrasonic cleaner for ~5 min and scrub again with pipette tip. Rinse with water.
If the beak drying out is a concern, they can be stored in water at 4°C and then placed under the microscope when counting. To keep the beak flat, we suggest placing the beak between two pieces of glass secured with an adhesive tape during counting.
If it is determined that increments are not compromised with the beak dry, we suggest using an appropriate adhesive to fix your beak section to a microscope slide, flattening the section as much as possible with a wide, flat scalpel or knife (Fig. 11). Our preferred adhesive is Crystalbond 509 because it can be reheated to reshape mounts and cures quickly as it cools. The slide can then be easily referred to when needed.
Image of a Macroctopus maorum beak indicating the rostral tip, lateral wall and beak edge according to Clarke (1986). The counting line indicates the direction for counting of growth increments (from edge to rostral tip), and the scissors indicate where to section if using the lateral wall surface.
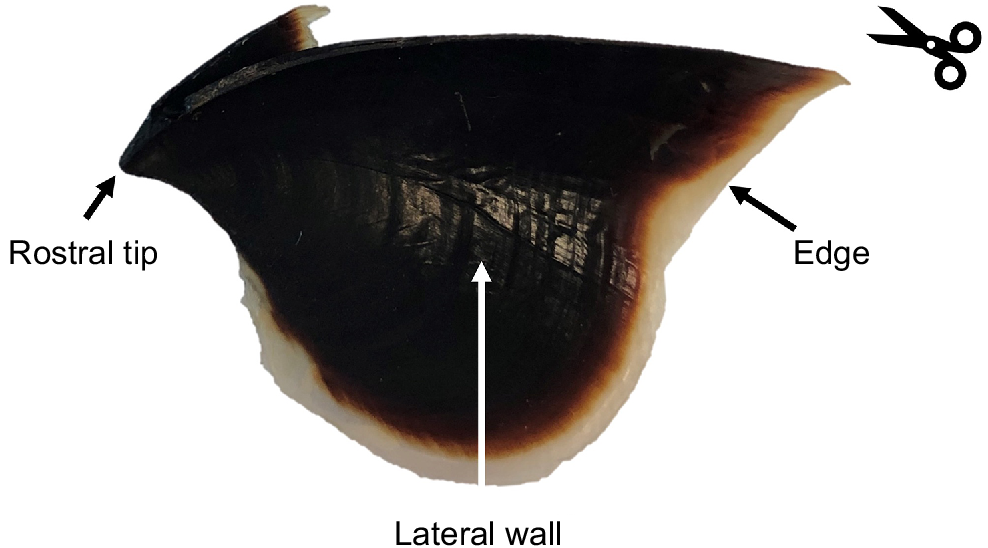
Preparing the LWS of beaks from hatchling and juveniles
Extra small and thin beaks, such as those in hatchlings, are carefully dissected, cleaned with water and a plastic pipette, butterflied with the inside facing up, and mounted to a slide in warmed glycerol gelatin and a coverslip. Slightly larger hatchling beaks are cut in half sagittally, as in adult octopus, and mounted face up on a slide with glycerol gelatin and a coverslip. The beak should be completely covered by the gelatin before placing the cover slip, and overheating of the gelatin should be avoided to prevent air bubbles from forming.
Visualising and counting growth increments
Beak growth increments can be visualised through microscopy (Fig. 12). Increments on thicker, larger beaks are more visible using reflective light, and increments on thinner, smaller beaks are more visible with transmitted light, but this varies with each species, and both and a combination of both should be trialled.
Micrograph of Octopus tetricus beak section (lateral wall surface) showing growth increments at 100× magnification. White lines highlight a few growth increments that can be seen.
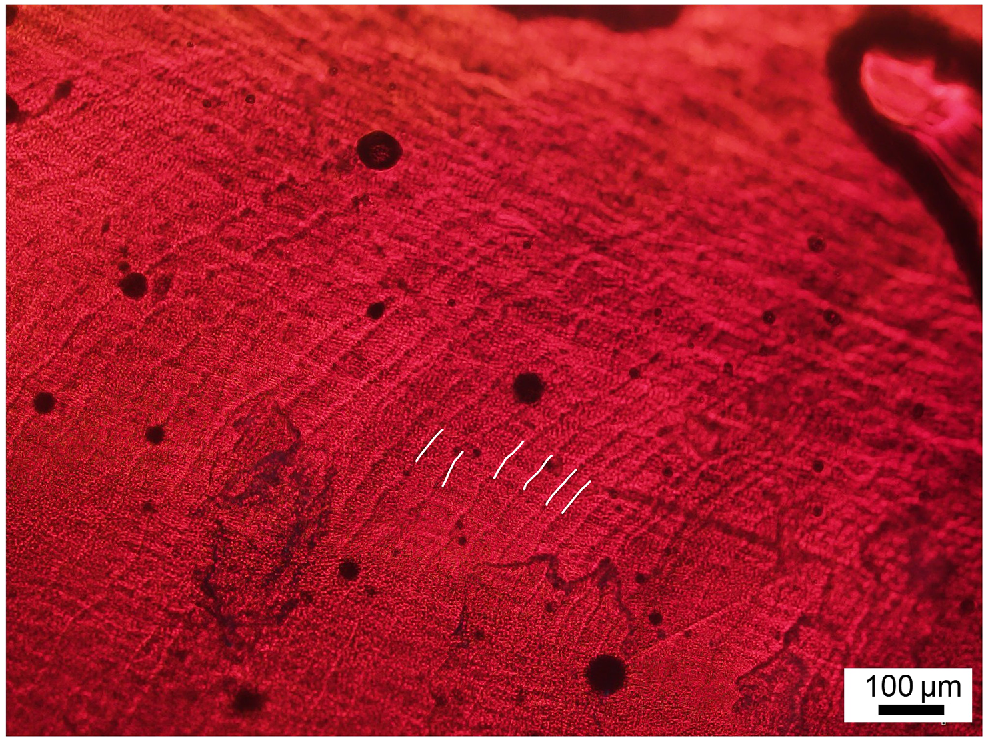
If good micrographs can be taken, increments can be successfully counted from a series of digital images that are individually focused and later stitched together. These images can then be easily referred back to and measurements, such as increments width, can be taken. In our experience, it is sometimes easier to count increments on beaks directly through the eyepiece while LWS sections are under the microscope because the three-dimensional surface profile of the increments require careful adjustment of the field of view across the section. Often, the edge of the beak needs to be scanned to find the area in which more increments are visible to find a starting point. As other studies have pointed out (Perales-Raya et al. 2010, 2014a), there are many scratches near the rostral tip due to feeding on hard-shelled crustaceans, making it difficult to read this area. Similarly, with stylets, we recommend multiple non-consecutive counts per trained reader, with data treated as described above.
Determining age using beak morphometrics
As with stylets, beak morphometrics such as weight and various measurements can also be used as a proxy of age, but increment periodicity first needs to be validated to determine the relationship between age and beak morphometrics. This methodology has been applied to Octopus vulgaris in which Perales-Raya et al. (2010) found well-fitted power relationships (R2 = 0.76) between the number of beak increments and beak mass as well as hood length. Although periodicity was not validated in this study, it was later validated as daily by Perales-Raya et al. (2014a). These data suggest that beak morphometrics have the potential to be effective proxies of age.
Part 3: validating periodicity of growth increments
Stylet and beak increment analysis are undertaken through counting growth increments, each of which often represent a single day of life (Donlon et al. 2019). However, increment deposition may be influenced by various abiotic and biotic factors, and non-daily periodicity has been observed in Octopus berrima stylets and beaks, with periodicity varying between the two structures (Xavier et al. 2022; Durante et al. 2023). Thus, daily growth ring deposition cannot be assumed. Consequently, validation of growth increment is a crucial first step in the ageing process for each species and each ageing structure. Age validation can be achieved through the analysis of known-age individuals, chemical staining or stress marking of the hard structures to mark time at liberty or in captivity when hatch date is unknown (e.g. for wild caught octopus). Determining the age and location of the first increment is also crucial for validation to determine if any increments are formed before hatching or there is a delay in which the first increment in formed (e.g. at 3 days old instead of at hatching) (Campana 2001; Doubleday et al. 2011; Lourenço et al. 2015). Only after both periodicity and the identification of the first increment have been described, can precise age estimates be made (if validation assumptions, discussed below, hold true).
At present, beak increment periodicity has been validated in a variety of species, including Octopus maya (Rodriguez-Domínguez et al. 2013), Octopus vulgaris (Perales-Raya et al. 2014a), Octopus insularis (Batista et al. 2021) and Octopus berrima (Durante et al. 2023). In addition, stylet increment periodicity has been validated in Octopus vulgaris (Hermosilla et al. 2010), Octopus djinda (previously Octopus cf. tetricus) (Leporati and Hart 2015), Robsonella huttoni (previously Octopus huttoni) (Donlon et al. 2019), Octopus pallidus (Doubleday et al. 2006) and Octopus australis (Nuttall 2009).
Validation assumptions
Validation that involves laboratory-reared animals obviously assumes that captivity does not influence increment periodicity; as such, age calculated from wild-caught individuals should always be regarded as an estimate. It has also been observed in one species of octopus (Octopus berrima) that periodicity may vary with factors such as temperature and rearing density (Durante et al. 2023). Although periodicity should ideally be validated throughout the life cycle of an individual, this is rarely feasible, and validation methods also generally assume that increment periodicity remains constant throughout an individual’s life. However, periodicity can be validated in juvenile stages using known-age methods and adult stages using chemical marking methods (Durante et al. 2023).
Known-age method
The known-age method can be used for ageing octopus with a known hatch date (e.g. for octopus raised in captivity). Age in days is compared with the number of growth increments counted on a structure (e.g. stylet or beak) to validate the periodicity of increment deposition (Hernández-López et al. 2001; Doubleday et al. 2006; Barratt and Allcock 2010; Bárcenas et al. 2014). To validate periodicity in known-age individuals, sample preparation and visualisation methods follow those described in Parts 1 and 2. A disadvantage of this method may be that somatic growth rates and increment deposition in individuals held in captivity may differ from individuals collected from the wild (Campana 2001). However, because the best way to have known-age octopus is to raise them in captivity, conditions should be as natural as possible, including seawater quality, temperature and ambient light.
Marking method (chemical staining and stress marking)
There are two well-known methods of marking hard parts: chemical staining (Hermosilla et al. 2010; Canali et al. 2011; Perales-Raya et al. 2014a; Leporati and Hart 2015; Batista et al. 2021) and stress marking (Perales-Raya et al. 2014a, 2014b). Stress marking can be done by either the stress of handling and capture (Perales-Raya et al. 2014b) or by thermal stress (Canali et al. 2011). The chemical staining method uses fluorescent stains to mark growing hard structures in individuals where hatch date is unknown. After staining, individuals are held for a known amount of time prior to euthanasia. Alternatively, marked animals could be released into the wild and recaptured after a designated time period, but this would be logistically challenging and has yet to be achieved for octopus. To determine increment periodicity, the total number of growth increments deposited after marking is compared with the total number of days held or at liberty (Perales-Raya et al. 2014a).
This method relies on the method effectively marking the hard part to the extent that a mark can be visualised through microscopy. With chemical staining, often the mark is fluorescent and requires a microscope with light of an appropriate wavelength. It is also essential that the stain is not toxic to the octopus.
Several stains have been successfully used to mark stylets and beaks, whereas others have been unsuccessful (Table 2). However, success is not always consistent among species or structures. For example, we found that Calcofluor white, a fluorescent stain that binds to cellulose and chitin in cell walls, effectively stained the stylets, but surprisingly, not the beaks of O. berrima (Durante et al. 2023), whereas Perales-Raya et al. (2014a) reported that it successfully marked O. vulgaris beaks. Tetracycline hydrochloride is a commonly used stain but can cause adverse effects on octopus health in some species (e.g. injection in adults can trigger arm autophagy) (Durante et al. 2023; Karina Hall, pers. comm.). Therefore, we do not recommend tetracycline as a stain for new species due to potential adverse effects. In this guide, we will describe how to chemically mark octopus using Calcofluor white.
Chemical stain | Structure | Species | Reference | |
---|---|---|---|---|
Tetracycline hydrochloride | Stylet | Octopus berrima: clear stain mark, but adverse effect on octopus health | Durante et al. (2023) | |
Octopus huttoni: clear stain mark | Donlon et al. (2019) | |||
Octopus vulgaris: clear stain mark | Hermosilla et al. (2010) | |||
Octopus australis: diffused stain mark | Nuttall (2009) | |||
Beak | Octopus berrima: diffused stain mark | Durante et al. (2023): | ||
Calcofluor White | Stylet | Octopus berrima: clear stain mark | Durante et al. (2023) | |
Beak | Octopus vulgaris: clear stain mark | Perales-Raya et al. (2014a) | ||
Octopus berrima: diffused stain mark | Durante et al. (2023) | |||
Congo red | Beak | Octopus vulgaris: diffused stain mark | Perales-Raya et al. (2014a) | |
Alizarin Complexone | Stylet | Octopus australis: clear stain mark | Nuttall (2009) | |
Alizarin Red S | Stylet | Octopus australis: clear stain mark | Nuttall (2009) | |
Calcine | Stylet | Octopus dijinda (Octopus cf. tetricus): clear stain mark | Leporati and Hart (2015) |
Injection is the most widely practised and recommended method of chemical stains for octopus. Submersion in a seawater bath containing the chemical stain has also been explored. However, adequate levels of chemical may not be absorbed and there is also a risk of the chemical becoming oxidised and losing its fluorescent ability (Donlon et al. 2019). Euthanased octopus that have undergone chemical staining should be stored and dissected in the dark. Similarly, stylet and beak samples must be stored, prepared and embedded in a darkened room to prevent stain oxidation.
A stock solution of Calcofluor can be prepared following the methods outlined in Perales-Raya et al. (2014a). This solution is concentrated to 50 mg mL−1 to minimise injection volume. However, the concentration can be altered as required for different sized octopus.
Add 750 mg of Calcofluor White to 15 mL of autoclaved seawater, place on a magnetic stir plate with a stir bar and heat to 30°C.
Add 15 drops of potassium hydroxide to increase solubility and 3.75 mL of 0.2-M phosphate buffer solution (pH 6.8).
Wrap solution in tin foil, allow to cool to room temperature and store in the dark at 4°C until use.
For chemical staining, octopus have been sedated through cold water immersion (Perales-Raya et al. 2014a; Donlon et al. 2019) or anesthetised through chemical solution immersion prior to the injection process (Fiorito et al. 2015). In our experience, octopus sedated with cold water are stiff, making it difficult to inject staining solution into the muscle. In comparison, octopus anaethetised with magnesium chloride have relaxed muscles, which may make it easier for injections (E. Durante, pers. comm.). Magnesium chloride is also one the most widely used sedatives for octopus. However, we recommend referring to the following guides for comprehensive information on the care and welfare of cephalopods in the laboratory, including sedation: Andrews et al. (2013), Fiorito et al. (2015) and Doubleday et al. (2022). We also highly recommend that researchers review the latest best-practice procedures for chemical staining and sedation of octopus in the literature, as well as through their local animal ethics committees.
Once sedated, place octopus on tared scale and record weight. This does not need to be exact as it is just to calculate the quantity of stain to inject.
Return octopus to water and calculate injection volume required (y, mL) following recommended injection concentration as per Perales-Raya et al. (2014a) and formula below:
where total weight is the mass in grams, stain concentration is the mass in milligrams, and concentration of stock solution is 50 mg mL−1.
Inject solution intramuscularly at the base of the thickest arm (usually a ventral arm). Some researchers suggest injecting in the mantle, but the site of injection had not been investigated thoroughly and is currently based off of what worked for previous studies.
Return octopus to a solitary container and flush fresh seawater into the mantle and over the gills until octopus movement recovers. Octopus are considered fully recovered when breathing returns to a normal rate, skin colouration returns, octopus respond to stimuli and all arms are functioning. When recovered, they can be returned back to their original housing.
To analyse stained stylets and beaks, follow the same procedures as outlined in parts 1 and 2. However, all work must be carried out in the dark to prevent oxidisation of the stain. Visualisation of the fluorescent mark also requires a microscope fitted with an ultraviolet filter or other light source of an appropriate wavelength (~380–475 nm).
Take an image of the stained section under a fluorescent microscope to locate the mark (Fig. 13).
Take another image in the same position under white light to visualise increments.
Aligning the two images, count the number of growth increments in the second image from the edge of the chemical stain to the edge of the section.
Repeat to produce at least two, non-consecutive counts as with unstained sections.
Average the counts and compare with the number of days from staining to euthanasia to validate growth increment periodicity.
(a) Micrograph of a Calcofluor-stained Octopus berrima stylet section showing the edge of the stain mark and the edge of the stylet and (b) micrograph of the lateral wall of a Octopus tetricus upper beak that has been stained with tetracycline. Brackets indicate the section in which the fluorescent mark was formed from the tetracycline.
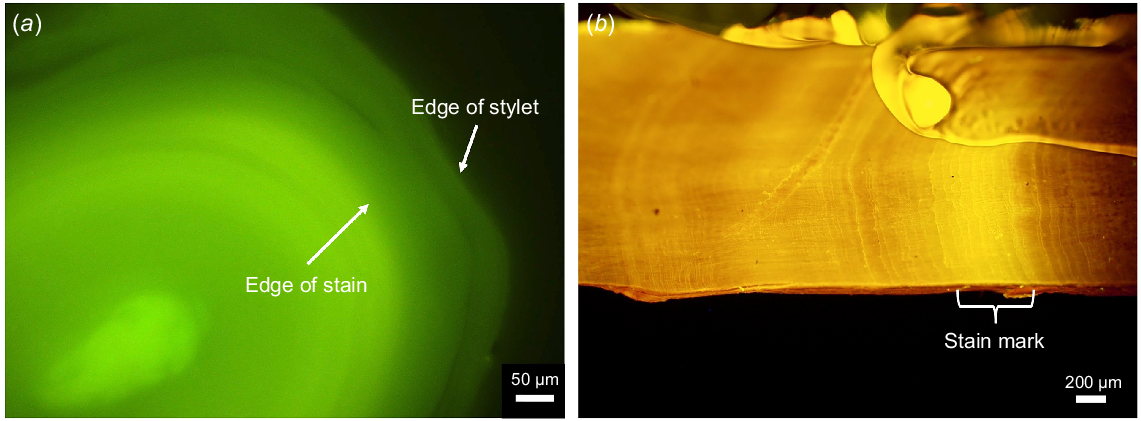
Identifying first post-hatch increment in stylets and stylet core
To estimate the position of the first post-hatch increment or size of the stylet core, as well as determine if stylets are present immediately post-hatching, whole hatchlings can be sectioned using histological methods outlined in Lourenço et al. (2015) and summarised below (Fig. 14):
Fix whole hatchlings in a mixture of formalin acetic acid calcium chloride (FAACC) for 48 h then transfer to 70% ethanol and store for at least 24 h before processing. FAACC comprised:
Process samples following the paraffin-embedding sequence outlined in Tables 3.
Trim paraffin blocks until a cross-section of the mantle is seen and cut 5-μm sections. Additional trimming may be required if the stylet is not visible post-staining and mounting.
Using a warm water bath, place sections on a slide, flatten under filter paper soaked with 20% ethanol and a roller, and leave to dry for a few hours or overnight.
Dewax and stain samples following the sequence outlined in Tables 4. Alternative stains can also be used (e.g. Lourenço et al. 2015 used Masson’s trichrome stain), but we found methyl blue to be sufficient.
Cover slip with slide mounting medium DPX.
Using a microscope, observe sections and measure the diameter of the stylet cross-section and any visible increments.
Micrograph of a 3-day-old Octopus berrima hatchling cross section at 20× magnification. Stylet section is indicated within the box.
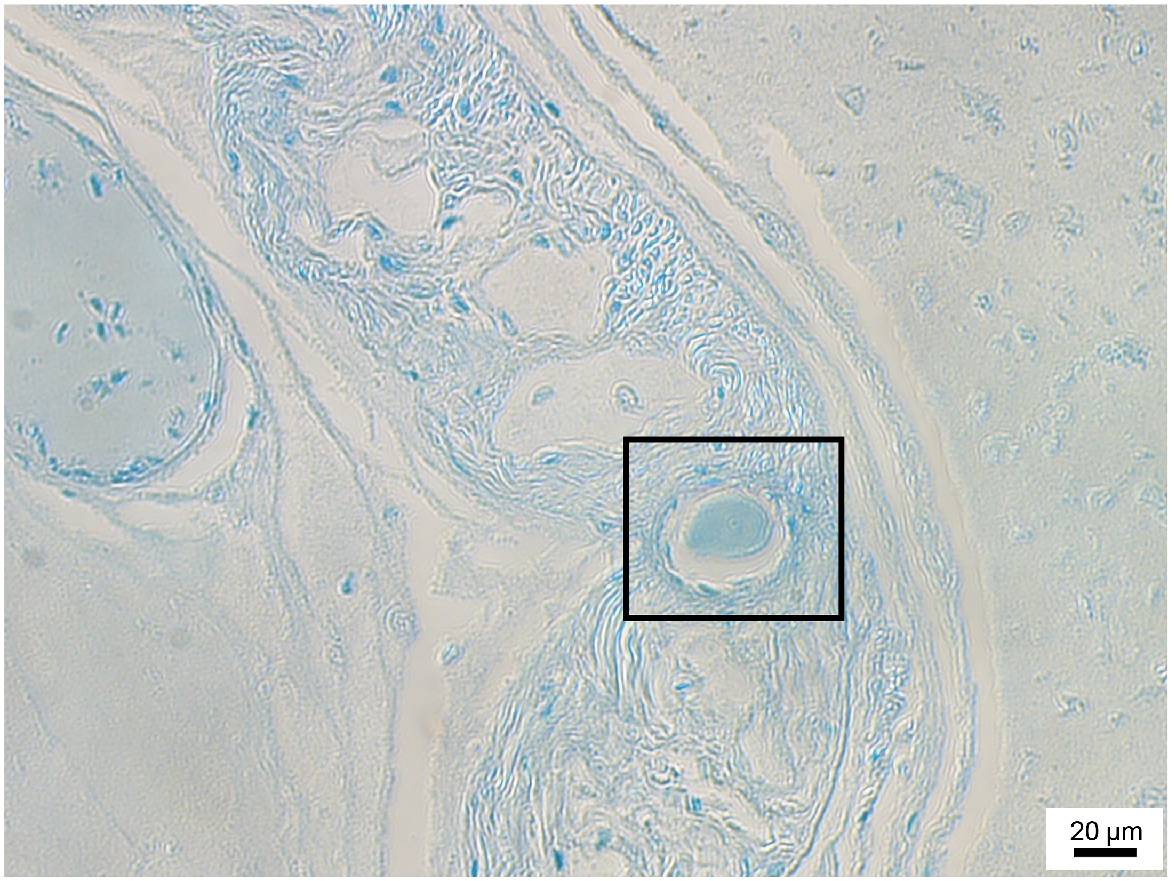
Identifying first post-hatch increment in beaks
As with stylets, it is important to know when the first beak increment was formed and how many, if any, they hatch with. This is done by using the methods for small beaks described above to closely observe freshly hatched hatchlings or paralarvae to determine if any increments are present. Everyday thereafter, beaks of individuals raised in captivity should be observed to determine at what age the first increment forms.
Part 4: potential ageing methods: avenues for further research
In some instances, increment analysis of stylets and beaks may not be a suitable ageing method due to poor increment readability or variable increment periodicity. Further research is needed to develop ageing methods for application in such instances. We present two additional potential avenues below.
Eye lens analysis
Analysing growth increments in eye lenses has been explored as an ageing method when traditional ageing methods have yielded unsatisfactory readings. Lenses can be fixed in neutral formalin before being dehydrated, and either embedded in paraffin to produce histological slides (Luna 1968; Baqueiro-Cárdenas et al. 2011; Rodriguez-Domínguez et al. 2013) or embedded in synthetic resin to produce thin slides (Baqueiro-Cárdenas et al. 2011). Baqueiro-Cárdenas et al. (2011) found a correlation between the number of eye lens growth increments and age in Enteroctopus megalocyathus. However, subsequent validation of this method using O. maya indicated no relationship between number of eye lens increments and age (Rodriguez-Domínguez et al. 2013).
Lipofuscin quantification
Lipofuscin quantification involves quantification of age pigment lipofuscin using histological methods (Arkhipkin et al. 2018). Lipofuscin is generated during normal metabolism and accumulates within nervous tissue over time; thus, it may be used as a proxy for age (Doubleday and Semmens 2011). Lipofuscin quantification is currently the primary method used for ageing in crustaceans, having been successfully applied to a range of marine species (Kodama et al. 2006; Puckett et al. 2008; Matthews et al. 2009; Harvey et al. 2010). Lipofuscin quantification has been explored as an alternative ageing method in O. pallidus (Doubleday and Semmens 2011) and O. huttoni (Donlon et al. 2019), with mixed results. However, more research is needed on more individuals, across different life stages and species.
Part 5: choosing the best method
Given that periodicity validation experiments are usually costly and challenging to complete, the first step in developing an ageing method for a new octopus species is to ascertain whether any clear growth increments can be visualised in the hard structures. Initial trials to establish preparation methods can usually be achieved with a small number of specimens and at minimal expense using the steps outlined in this guide. Once an approach for increment visualisation and analysis has been established, it is essential to follow with some form of age validation to determine the periodicity of increment formation. Validation should be preferably done for different life stages (Campana 2001; Doubleday et al. 2006), as well as different ageing structures if multiple ageing structures are used (Durante et al. 2023). Only then can increment counts from hard structures be converted into accurate age estimates.
For some octopus species, stylet and beak increments have been detected but periodicity is yet to be validated, and for a handful of others, increment periodicity has been validated, and ageing methods successfully applied (Table 5). These past successes provide a valuable starting point for future ageing studies. However, for many octopus species, stylet and beak growth increments are yet to be visualised; therefore, an initial period of method development is required. To assist with the ageing process, we provide a flow chart indicating the main steps and decision points (Fig. 15).
Species | Increments visualised | Periodicity validated | Recommended ageing method(s) | Reference | |
---|---|---|---|---|---|
Holobenthic | |||||
Octopus berrima | Yes | Yes (non-daily) | Further validation required on different life history stages | Durante et al. (2023) | |
Octopus maya | Yes | Yes | Beak increment analysis | Rodriguez-Domínguez et al. (2013)Bárcenas et al. (2014) | |
Octopus pallidus | Yes | Yes | Stylet increment analysis | Doubleday et al. (2006)Leporati et al. (2008) | |
Merobenthic | |||||
Eledone cirhhosa | Yes | No | Barratt and Allcock (2010) | ||
Octopus djinda (Octopus cf. tetricus) | Yes | Yes | Stylet increment analysis Stylet weight | Leporati and Hart (2015) | |
Octopus huttoni | Yes | Yes | Further validation required on different life history stages | Donlon et al. (2019) | |
Octopus vulgaris | Yes | Yes | Stylet increment analysis Beak increment analysis | Barratt and Allcock (2010)Hermosilla et al. (2010)Hernández-López et al. (2001)Perales-Raya et al. (2014a) |
We also list species that have readable increments, but validation is still required.
Declaration of funding
We acknowledge funding support from the Fisheries Research and Development Corporation on behalf of the Australian Government, gained in collaboration with Natalie Moltschaniwskyj and Matt Broadhurst from NSW Department of Primary Industries and Brendan Kelaher from Southern Cross University.
Acknowledgements
We thank Justin Payne and Sofia Hassiotis (University of South Australia) for training and use of their laboratories and equipment. We also thank Kait Harris, Kyle Goodman, Nick Meadows, Anne-Marie Hegarty and staff at the Sydney Fish Markets, Coffs Harbour Fishermen’s Co-operative, Clarence River Fishermen’s Co-Operative Ltd, and several NSW commercial fishers for assistance in collecting and processing octopus samples.
References
Andrews PLR, Darmaillacq A-S, Dennison N, Gleadall IG, Hawkins P, Messenger JB, Osorio D, Smith VJ, Smith JA (2013) The identification and management of pain, suffering and distress in cephalopods, including anaesthesia, analgesia and humane killing. Journal of Experimental Marine Biology and Ecology 447, 46-64.
| Crossref | Google Scholar |
Arkhipkin AI, Bizikov VA, Doubleday ZA, Laptikhovsky VV, Lishchenko FV, Perales-Raya C, Hollyman PR (2018) Techniques for estimating the age and growth of molluscs: Cephalopoda. Journal of Shellfish Research 37(4), 783-792.
| Crossref | Google Scholar |
Balguerías E, Quintero ME, Hernández-González CL (2000) The origin of the Saharan Bank cephalopod fishery. ICES Journal of Marine Science 57(1), 15-23.
| Crossref | Google Scholar |
Baqueiro-Cárdenas ER, Correa SM, Contreras Guzman R, Barahona N, Briceño F, Villegas MJ, Paredes R (2011) Eye lens structure of the octopus Enteroctopus megalocyathus: evidence of growth. Journal of Shellfish Research 30(2), 199-204.
| Crossref | Google Scholar |
Barratt IM, Allcock AL (2010) Ageing octopods from stylets: development of a technique for permanent preparations. ICES Journal of Marine Science 67(7), 1452-1457.
| Crossref | Google Scholar |
Batista BB, Matthews-Cascon H, Marinho RA, Kikuchi E, Haimovici M (2021) The growth and population dynamics of Octopus insularis targeted by a pot longline fishery in north-eastern Brazil. Journal of the Marine Biological Association of the United Kingdom 101(6), 935-946.
| Crossref | Google Scholar |
Bizikov V (2004) The shell in Vampyropoda (Cephalopoda): morphology, functional role and evolution. Ruthenica 3, 1-88.
| Google Scholar |
Bárcenas GV, Perales-Raya C, Bartolomé A, Almansa E, Rosas C (2014) Age validation in Octopus maya (Voss and Solís 1966) by counting increments in the beak rostrum sagittal sections of known age individuals. Fisheries Research 152, 93-97.
| Crossref | Google Scholar |
Caddy JF, Rodhouse PG (1998) Cephalopod and groundfish landings: evidence for ecological change in global fisheries? Reviews in Fish Biology and Fisheries 8(4), 431-444.
| Crossref | Google Scholar |
Campana SE (2001) Accuracy, precision and quality control in age determination, including a review of the use and abuse of age validation methods. Journal of Fish Biology 59, 197-242.
| Crossref | Google Scholar |
Canali E, Ponte G, Belcari P, Rocha F, Fiorito G (2011) Evaluating age in Octopus vulgaris estimation, validation and seasonal differences. Marine Ecology Progress Series 441, 141-149.
| Crossref | Google Scholar |
Donlon EMY, Damsteegt EL, McKinnon J, Higgins FA, Lamare MD (2019) Growth and age of the midget octopus, Octopus huttoni. Aquatic Ecology 53(4), 689-706.
| Crossref | Google Scholar |
Doubleday ZA, Semmens JM (2011) Quantification of the age-pigment lipofuscin in known-age octopus (Octopus pallidus): a potential tool for age determination. Journal of Experimental Marine Biology and Ecology 397(1), 8-12.
| Crossref | Google Scholar |
Doubleday Z, Semmens JM, Pecl G, Jackson G (2006) Assessing the validity of stylets as ageing tools in Octopus pallidus. Journal of Experimental Marine Biology and Ecology 338(1), 35-42.
| Crossref | Google Scholar |
Doubleday ZA, White J, Pecl GT, Semmens JM (2011) Age determination in merobenthic octopuses using stylet increment analysis: assessing future challenges using Macroctopus maorum as a model. ICES Journal of Marine Science 68(10), 2059-2063.
| Crossref | Google Scholar |
Doubleday ZA, Prowse TAA, Arkhipkin A, Pierce GJ, Semmens J, Steer M, Leporati SC, Lourenço S, Quetglas A, Sauer W, Gillanders BM (2016) Global proliferation of cephalopods. Current Biology 26(10), R406-R407.
| Crossref | Google Scholar | PubMed |
Durante ED, Grammer GL, Martino JC, Payne JL, Doubleday ZA (2023) Nondaily growth increments in the commercial species, Octopus berrima, and the importance of age validation. ICES Journal of Marine Science 81, 293-306.
| Crossref | Google Scholar |
Fiorito G, Affuso A, Basil J, Cole A, de Girolamo P, D’Angelo L, Dickel L, Gestal C, Grasso F, Kuba M, Mark F, Melillo D, Osorio D, Perkins K, Ponte G, Shashar N, Smith D, Smith J, Andrews PLR (2015) Guidelines for the care and welfare of cephalopods in research –a consensus based on an initiative by CephRes, FELASA and the Boyd Group. Laboratory Animals 49, 1-90.
| Crossref | Google Scholar | PubMed |
Food and Agriculture Organization of the United Nations (2022) ‘The state of world fisheries and aquaculture 2022. Towards blue transformation.’ (FAO: Rome, Italy) Available at https://www.fao.org/documents/card/en?details=cc0461en
Franco-Santos RM, Perales-Raya C, Almansa E, De Troch M, Garrido D (2016) Beak microstructure analysis as a tool to identify potential rearing stress for Octopus vulgaris paralarvae. Aquaculture Research 47, 3001-3015.
| Crossref | Google Scholar |
Harvey HR, Ju S-J, Son S-K, Feinberg LR, Shaw CT, Peterson WT (2010) The biochemical estimation of age in euphausiids: laboratory calibration and field comparisons. Deep-Sea Research – II. Topical Studies in Oceanography 57(7), 663-671.
| Crossref | Google Scholar |
Hermosilla CA, Rocha F, Fiorito G, González ÁF, Guerra Á (2010) Age validation in common octopus Octopus vulgaris using stylet increment analysis. ICES Journal of Marine Science 67(7), 1458-1463.
| Crossref | Google Scholar |
Hernández-López JL, Castro-Hernández JJ, Hernández-García V (2001) Age determined from the daily deposition of concentric rings on common octopus (Octopus vulgaris) beaks. Fishery Bulletin 99(4), 679-684.
| Google Scholar |
Kodama K, Shiraishi H, Morita M, Horiguchi T (2006) Verification of lipofuscin-based crustacean ageing: seasonality of lipofuscin accumulation in the stomatopod Oratosquilla oratoria in relation to water temperature. Marine Biology 150(1), 131-140.
| Crossref | Google Scholar |
Leporati SC, Hart AM (2015) Stylet weight as a proxy for age in a merobenthic octopus population. Fisheries Research 161, 235-243.
| Crossref | Google Scholar |
Leporati SC, Semmens JM, Pecl GT (2008) Determining the age and growth of wild octopus using stylet increment analysis. Marine Ecology Progress Series 367, 213-222.
| Crossref | Google Scholar |
Lourenço S, Moreno A, Narciso L, Pereira J, Rosa R, González ÁF (2015) Stylet (vestigial shell) size in Octopus vulgaris (Cephalopoda) hatchlings used to determine stylet nucleus in adults. Journal of the Marine Biological Association of the United Kingdom 95(6), 1237-1243.
| Crossref | Google Scholar |
Martino JC, Steer M, Doubleday ZA (2021) Supporting the sustainable development of Australia’s octopus industry: first assessment of an artisanal fishery. Fisheries Research 241, 105999.
| Crossref | Google Scholar |
Matthews TR, Maxwell KE, Bertelsen RD, Derby CD (2009) Use of neurolipofuscin to determine age structure and growth rates of Caribbean spiny lobster Panulirus argus in Florida, United States. New Zealand Journal of Marine and Freshwater Research 43(1), 125-137.
| Crossref | Google Scholar |
Perales-Raya C, Hernández-González CL (1998) Growth lines within the beak microstructure of the octopus Octopus vulgaris Cuvier, 1797. South African Journal of Marine Science 20(1), 135-142.
| Crossref | Google Scholar |
Perales-Raya C, Bartolomé A, García-Santamaría MT, Pascual-Alayón P, Almansa E (2010) Age estimation obtained from analysis of octopus (Octopus vulgaris Cuvier, 1797) beaks: improvements and comparisons. Fisheries Research 106(2), 171-176.
| Crossref | Google Scholar |
Perales-Raya C, Almansa E, Bartolomé A, Felipe BC, Iglesias J, Sánchez FJ, Carrasco JF, Rodríguez C (2014a) Age validation in Octopus vulgaris beaks across the full ontogenetic range: beaks as recorders of life events in octopuses. Journal of Shellfish Research 33, 481-493.
| Crossref | Google Scholar |
Perales-Raya C, Jurado-Ruzafa A, Bartolomé A, Duque V, Carrasco MN, Fraile-Nuez E (2014b) Age of spent Octopus vulgaris and stress mark analysis using beaks of wild individuals. Hydrobiologia 725(1), 105-114.
| Crossref | Google Scholar |
Perales-Raya C, Nande M, Roura A, Bartolomé A, Gestal C, Otero JJ, García-Fernández P, Almansa E (2018) Comparative study of age estimation in wild and cultured Octopus vulgaris paralarvae: effect of temperature and diet. Marine Ecology Progress Series 598, 247-259.
| Crossref | Google Scholar |
Puckett BJ, Secor DH, Ju S-J (2008) Validation and application of lipofuscin-based age determination for chesapeake bay blue crabs Callinectes sapidus. Transactions of the American Fisheries Society 137(6), 1637-1649.
| Crossref | Google Scholar |
Rodhouse PGK, Pierce GJ, Nichols OC, Sauer WHH, Arkhipkin AI, Laptikhovsky VV, Lipiński MR, Ramos JE, Gras M, Kidokoro H, Sadayasu K, Pereira J, Lefkaditou E, Pita C, Gasalla M, Haimovici M, Sakai M, Downey N (2014) Environmental effects on cephalopod population dynamics: implications for management of fisheries. In ‘Advances in marine biology. Vol. 67’. (Ed. EAG Vidal) pp. 99–233. (Academic Press) doi:10.1016/B978-0-12-800287-2.00002-0
Rodríguez-Domínguez A, Rosas C, Méndez-Loeza I, Markaida U (2013) Validation of growth increments in stylets, beaks and lenses as ageing tools in Octopus maya. Journal of Experimental Marine Biology and Ecology 449, 194-199.
| Crossref | Google Scholar |
Sauer WHH, Gleadall IG, Downey-Breedt N, Doubleday Z, Gillespie G, Haimovici M, Ibáñez CM, Katugin ON, Leporati S, Lipinski MR, Markaida U, Ramos JE, Rosa R, Villanueva R, Arguelles J, Briceño FA, Carrasco SA, Che LJ, Chen C-S, Cisneros R, Conners E, Crespi-Abril AC, Kulik VV, Drobyazin EN, Emery T, Fernández-Álvarez FA, Furuya H, González LW, Gough C, Krishnan P, Kumar B, Leite T, Lu C-C, Mohamed KS, Nabhitabhata J, Noro K, Petchkamnerd J, Putra D, Rocliffe S, Sajikumar KK, Sakaguchi H, Samuel D, Sasikumar G, Wada T, Zheng X, Tian Y, Pang Y, Yamrungrueng A, Pecl G (2021) World octopus fisheries. Reviews in Fisheries Science & Aquaculture 29(3), 279-429.
| Crossref | Google Scholar |
Sousa-Reis C, Fernandes R (2002) Growth observations on Octopus vulgaris Cuvier, 1797 from the Portuguese waters: growth lines in the vestigial shell as possible tools for age determination. Bulletin of Marine Science 71, 1099-1103.
| Google Scholar |
Xavier JC, Golikov AV, Queirós JP, Perales-Raya C, Rosas-Luis R, Abreu J, Bello G, Bustamante P, Capaz JC, Dimkovikj VH, González AF, Guímaro H, Guerra-Marrero A, Gomes-Pereira JN, Hernández-Urcera J, Kubodera T, Laptikhovsky V, Lefkaditou E, Lishchenko F, Luna A, Liu B, Pierce GJ, Pissarra V, Reveillac E, Romanov EV, Rosa R, Roscian M, Rose-Mann L, Rouget I, Sánchez P, Sánchez-Márquez A, Seixas S, Souquet L, Varela J, Vidal EAG, Cherel Y (2022) The significance of cephalopod beaks as a research tool: an update. Frontiers in Physiology 13, 1038064.
| Crossref | Google Scholar |