Severe, large-scale bushfire threatens metapopulation function of quokka (Setonix brachyurus) in south-western Australia
Karlene Bain A * , Merril Halley B and Adrian Wayne CA Python Ecological Services, PO Box 355, Walpole, WA 6398, Australia.
B Deceased. Formerly of World Wildlife Fund Australia, PO Box 2167, Churchlands, WA 6018, Australia.
C Department of Biodiversity Conservation and Attractions, Parks and Wildlife Service, Locked Bag 2, Manjimup, WA 6258, Australia.
International Journal of Wildland Fire 32(8) 1175-1186 https://doi.org/10.1071/WF23008
Submitted: 23 January 2023 Accepted: 13 May 2023 Published: 20 June 2023
© 2023 The Author(s) (or their employer(s)). Published by CSIRO Publishing on behalf of IAWF. This is an open access article distributed under the Creative Commons Attribution-NonCommercial-NoDerivatives 4.0 International License (CC BY-NC-ND)
Abstract
Background: The risk of large, severe bushfires is increasing in Australia, and little is known about their impact on threatened species.
Aims: This study aimed to investigated the abundance, occupancy and metapopulation structure of the quokka (Setonix brachyurus) for 5 years following a large, severe bushfire in Western Australia.
Methods: Data collected across spatiotemporally replicated transects were used to estimate probabilities of occupancy and abundance, and to evaluate the influence of covariates.
Key results: Quokka abundance was reduced to 16% of pre-fire levels, with distances up to 74 km separating subpopulations. Recolonising quokkas occupied habitat in parts of the landscape that had retained some vertical vegetation structure and contained a sedge-dominated understorey, and where damage by feral pigs was low. This coincided with topographic refugia that consisted of deep, mesic riparian systems or perched wetland systems. After 5 years, abundance had increased to 46% of pre-fire levels, with distances of 5–30 km separating subpopulations.
Conclusion: Quokka recovery rate is slower than expected, and the metapopulation remains vulnerable to further fragmentation arising from disturbances that affect habitat patch suitability and movement corridors.
Implications: Proactive protection of refugia and spatiotemporal habitat connectivity within and surrounding the fire scar is critical for metapopulation recovery.
Keywords: bushfire, fire refugia, fire severity, habitat connectivity, metapopulation structure, occupancy, recolonisation patterns, threatened species.
Introduction
Fire is an important ecological process in many Australian ecosystems, and its effects on fauna are thought to depend largely on the scale and severity of the fire (Gill and Catling 2002; Bradstock 2008; Lindenmayer et al. 2013). Less intense fires are generally slower moving, with their effects limited to the ground cover and understorey, and they usually result in unburnt patches that provide refuge habitat (refugia) for fauna to survive the fire. More intense fires often move rapidly and affect all elements of the vegetation structure, resulting in larger scale and more severe impacts (Gill and Catling 2002; Bain et al. 2016).
The survival of species following large and intense fires is often dependent on the availability and connectivity of refugia, which provide essential resources while the surrounding landscape is recovering (Robinson et al. 2013; Bain et al. 2016; Driscoll et al. 2021). Following high intensity fires, these refugia are often intermittently distributed, relatively small and occur in parts of the landscape that are most protected by edaphic barriers to fire (Leonard et al. 2014; Berry et al. 2015; Bain et al. 2016). The conservation of threatened species in these altered landscapes is challenging, particularly where large distances separate patches of suitable habitat and metapopulation structure has been disrupted (Nimmo et al. 2019; Driscoll et al. 2021).
The expected increase in the occurrence of large-scale and intense bushfires in response to drying climatic conditions is of concern in this context (Cary and Banks 2000; Clarke et al. 2011; Abram et al. 2021). Also of concern is the potentially cumulative effects of land management practices, such as prescribed burning, which may inadvertently further disrupt connectivity between habitat patches.
In south-western Australia, prescribed burning is a management tool that is applied widely to reduce the scale and impact of uncontrolled bushfires on human life, property, infrastructure and biodiversity (Burrows and McCaw 2013; Howard et al. 2020). A nominal 200 000 ha annual prescribed burning target is set between Perth and Albany to achieve these outcomes (Department of Biodiversity, Conservation and Attractions 2020). Individual prescribed burn areas can be up to 20 000 ha in size. Furthermore, adjoining areas are often burnt within 1–3 years to take advantage of low fuel loads that improve boundary security for successive burns (Department of Biodiversity, Conservation and Attractions 2023). The assumption used by fire managers is that fauna subpopulations will have recovered sufficiently in those areas already burnt, and that adequate refuge will be available for newly displaced fauna.
However, after particularly large and intense fires the recovery of vegetation and fauna may be limited within the first 1–3 years. On 28 January 2015, a large lightning-caused fire occurred in the Shannon National Park, east of Northcliffe, Western Australia (the Northcliffe Fire). Wind speeds reached up to 50 km per hour and flame heights of over 40 m were reported in the karri (Eucalyptus diversicolor) forest (Department of Fire and Emergency Services 2015). The fire burnt under these intense conditions for 6 days and then continued burning under variable intensities for another 6 days. The area directly impacted by the fire was up to 74 km long and 30 km wide and covered an area of approximately 98 000 ha (DFES 2015). Fire at this intensity and scale provides a number of challenges for the survival and recolonisation of threatened species such as the quokka (Setonix brachyurus), which is a small endemic browsing macropod that is often used as a focal species for fire management in this region.
The quokka is classified as vulnerable by the International Union for Conservation of Nature and has been lost from over 50% of its former range on the mainland since European settlement (Kitchener 1995; Woinarski et al. 2014). The southern forest of Western Australia, between Nannup and Albany, now supports the most extensive remaining quokka population on the mainland, and the most genetically diverse population of the species (Spencer et al. 2019). Quokkas in this region appear to be operating as a functional metapopulation, with genetic connectivity maintained between spatially discrete habitat patches and reports of frequent localised extinctions and colonisations (Bain et al. 2015; Spencer et al. 2019). Individuals move up to 10 km in a night within stable home ranges and have been recorded dispersing more than 14 km to establish a new home range (Bain et al. 2020). Movement across large distances is therefore possible for this species where suitable habitat and corridors are present.
Given the scale and severity of the Northcliffe fire, we hypothesised that it would take longer than 3 years for quokkas to recover to pre-fire abundance and distribution. This project investigated the abundance, occupancy and recolonisation patterns of quokkas in the Northcliffe fire area for 5 years following the fire. The intent of the project was to determine whether effective recolonisation had occurred within core areas of the fire-affected landscape after 3 years, particularly in those areas most intensely burnt, and if not, the likely implications for metapopulation function. The project also aimed to identify factors driving occupancy in a post-fire environment to improve capacity for effective habitat protection and reestablishment of connectivity between habitat patches.
Methods
Study area
South-western Australia has a relatively intact fauna compared with other parts of Australia, having experienced unusually low rates of mammal extinction in the past 200 years (McKenzie et al. 2007). The area is characterised by high annual rainfall (800–1400 mm), dense vegetation and the occurrence of moist and stable ecosystems that have remained virtually unchanged for millions of years (Hopper 1979; McKenzie et al. 2007). These moist and stable ecosystems are among those most likely to be protected from fire by virtue of their differential moisture from the surrounding landscape (Robinson et al. 2013; Meddens et al. 2018).
The Northcliffe fire occurred in a large area of forest between the towns of Northcliffe and Walpole, Western Australia (Fig. 1). Forests in the region are dominated by jarrah (Eucalyptus marginata), marri (Corymbia calophylla) and karri (Eucalyptus diversicolor), with the karri growing up to 80 m tall. About 96% of the area that burnt in the fire is National Park or State Forest, vested in the Conservation Commission for the purpose of conservation and multiple use. The remaining area is private property. This project focused on the areas of the Northcliffe fire that are vested in the Conservation Commission, as well as a 1 km strip of conservation land adjacent to the fire scar (Fig. 1).
Site selection
In total, 126 sites were surveyed for quokka occupancy and abundance. Site selection was based on:
Occupation prior to the fire: these sites were identified using GIS-based historical records provided by the Department of Biodiversity Conservation and Attractions, Parks and Wildlife Service. High-confidence records dated within 3 years prior to the fire were used.
Site independence: quokkas in this region have a stable home range of up to 70 ha (maximum recorded diameter 3 km) (Bain et al. 2020). Where possible, survey transects were separated by at least 5 km to reduce the likelihood of more than one transect occurring within an animal’s home range. This was not always possible without sacrificing large numbers of records. Where sites were too close together to ensure independence, these sites were surveyed on the same day. Surveys were completed within a single survey period in summer/autumn to reduce the likelihood of dispersal and emigration movements.
Topographical gradients: riparian systems were targeted as a starting point for surveys; however, transect alignments traversed the full available range of topography because quokkas in this region have been found to spend approximately 40% of their time in riparian vegetation and the remainder of their time in other ecotypes (Bain et al. 2020).
Distribution across a range of fire intensities, including canopy intact, canopy scorched and canopy defoliated.
Systematic coverage of the fire scar and surrounds, including areas within 1 km of the edge of the fire (40 sites), areas greater than 1 km from the edge of the fire (52 sites) and unburnt areas adjoining the fire scar (34 sites).
Quantifying abundance and occupancy
Counting fresh faecal pellet groups has been demonstrated to be an effective method for rapidly determining a quantitative estimate of abundance for quokkas in the southern forest (Bain et al. 2014). The technique is widely used by land managers in this region due to the ability for the method to be applied at large spatial scales with a result that is highly comparable to the outcomes of more intensive methods, such as mark–recapture techniques (Bain et al. 2014).
At each site, two transects 1 km in length were surveyed for quokkas, and the occurrences of all fresh faecal pellet groups (deposited overnight) within 2.5 m of the transect were recorded (Bain et al. 2014). The transect surveys were repeated twice within a 2-month period for each site. Surveys were undertaken between February and March in 2016, 2018 and 2020.
Habitat assessment
A suite of habitat variables was measured based on previous work (Bain et al. 2015, 2016, 2020), including: number of vegetation layers (vegetation structure); understorey density; canopy cover; density of woody debris; sedge cover; grass cover; degree of canopy scorch/defoliation (fire severity); area damaged by feral pigs (Sus scrofa); distance to unburnt canopy; distance to fire edge; and distance to nearest creek line (Table 1). The variables were recorded every 200 m along each transect and averaged for each site for each year of the survey.
Variable | Code | Description |
---|---|---|
Vegetation structure | VL | Number of vegetation layers including ground cover, understorey, multiple midstorey layers and overstorey. |
Canopy cover | CC | Average percentage of ground covered by the crown foliage, measured using digital cover estimation techniques (Macfarlane et al. 2000, 2007). |
Understorey density | UD | Density of understorey vegetation measured using a modified digital cover estimation technique involving use of a white sheet 2 m from the photographer and an image of the intervening vegetation taken horizontally. |
Sedge cover | SC | Average percentage of ground covered by sedges within the families of Restionaceae, Cyperaceae or Anarthriaceae, measured using digital cover estimation techniques (Macfarlane et al. 2000, 2007). |
Fire severity | FS | A categorical judgement of the relative severity of the fire, based on the status of the canopy in the first month following the fire. Categories: Unburnt (0) (vegetation completely unburnt); Canopy (1) (overstorey foliage not burnt by the fire); Canopy/Scorch (2) (overstorey foliage a mix of green canopy and scorched canopy); Scorch (3) (overstorey foliage browned as a result of radiant heat during the fire); Scorch/Defoliation (4) (overstorey foliage a mix of scorched and defoliated); Defoliated (5) (overstorey foliage completely removed during the fire). |
Damage by pigs | PD | Area of feral pig damage (m2) occurring within a 10 m radius of the survey point. Feral pig damage was identified through distinctive digging and wallowing. The extent of the damage was mapped in the field using a GPS, and the area estimated from the arising polygon in QGIS. Areas of damage were GPS’d outside of the 10 m radius, where the damage originated within and extended beyond this area. |
Years of pig damage | YP | The number of years that pig damage was detected at a site. |
Distance to unburnt canopy | DU | Shortest distance to an area with unburnt canopy, defined as overstorey foliage that remained undisturbed by the fire. Distances were measured in QGIS using the ruler function over an enhanced compression file of the fire mosaic that had been generated from satellite imagery (Department of Biodiversity, Conservation and Attractions 2015). |
Distance to edge of fire | DF | The shortest distance to the edge of the fire. Distances were measured in QGIS using the ruler function over an enhanced compression file of the fire mosaic that had been generated from satellite imagery (Department of Biodiversity, Conservation and Attractions 2015) |
Distance to a riparian system | DR | The shortest distance to a riparian system (river, creek line, swamp). Distances were measured in QGIS using the ruler function over aerial imagery with major and minor hydrology layers overlaid (Department of Biodiversity, Conservation and Attractions 2015; Department of Water and Environmental Regulation 2018) |
Understorey height | UH | Average height of vegetation from the ground to the lowest vegetation layer (m). |
Depth of woody debris | WH | Height of the near-surface woody debris on the forest floor above the leaf litter (m). Consists of suspended leaves, twigs, branches and bark from the understorey, midstorey and overstorey vegetation. |
Grass cover | GC | Average percentage of ground covered by grasses from Poaceae. Measured using digital cover estimation techniques (Macfarlane et al. 2000, 2007). |
Analysis
To reduce the number of habitat variables to a suitable level, the selection procedure of Hosmer and Lemeshow (2000) was used with a stricter cut-off of P > 0.10. Three habitat variables were removed from further analysis as a result. Models were then developed in PRESENCE (Hines 2006) to estimate probabilities of occupancy (Ψ) and detection (P), and to evaluate the influence of covariates for each year of the survey (MacKenzie et al. 2017, Table 2). Encounter histories were generated for each site by converting survey counts to detection and non-detection data. Spatially and temporally replicated transects were treated as occasions. Akaike’s Information Criterion with a small sample size correction (AICc) was used for model selection, and models with delta AICc values ≤2 were considered to have strong support (Burnham and Anderson 2002). Pearson Chi-Square goodness-of-fit tests were used to assess the fit of the occupancy models to the data (MacKenzie and Bailey 2004).
Model | ∆AICc | w | Model likelihood | k | |
---|---|---|---|---|---|
Year 1 | Ψ(VL DU DF SC FS PD DR) P(.)A | 0.0 | 0.6 | 1.0 | 8 |
Ψ(Full) P(.) | 2.7 | 0.2 | 0.3 | 9 | |
Ψ(VL DU DF SC PD) P(.) | 3.5 | 0.1 | 0.2 | 6 | |
Ψ(DU DF SC FS PD) P(.) | 12.5 | 0.0 | 0.0 | 6 | |
Ψ(CC DU DF SC FI) P(.) | 15.4 | 0.0 | 0.0 | 6 | |
Ψ(.) P(.) | 127.1 | 0.0 | 0.0 | 2 | |
Ψ(.) P(t) | 131.6 | 0.0 | 0.0 | 5 | |
Year 3 | Ψ(VL DU FS PD DR) P(.)A | 0.0 | 0.6 | 1.0 | 6 |
Ψ(VL DU SC FS PD DR) p(.)A | 1.7 | 0.3 | 0.4 | 7 | |
Ψ(VL DU DF SC FS PD) P(.) | 3.6 | 0.1 | 0.2 | 7 | |
Ψ(CC VL DU DF SC FS PD) P(.) | 5.3 | 0.0 | 0.1 | 8 | |
Ψ(Full) P(.) | 5.8 | 0.0 | 0.1 | 9 | |
Ψ(VL DU SC FI) P(.) | 24.3 | 0.0 | 0.0 | 5 | |
Ψ(VL PD DU FI) P(.) | 55.4 | 0.0 | 0.0 | 5 | |
Year 5 | Ψ(VL SC FS PD) P(.)A | 0.0 | 0.7 | 1.0 | 5 |
Ψ(VL SC PD DU FI) P(.) | 3.1 | 0.1 | 0.2 | 6 | |
Ψ(Full) P(.) | 3.5 | 0.1 | 0.2 | 10 | |
Ψ(PD VL DU SC YP) P(.) | 5.7 | 0.0 | 0.1 | 6 | |
Ψ(PD VL DU FI) P(.) | 16.4 | 0.0 | 0.0 | 5 | |
Ψ(VL SC DU) P(.) | 19.6 | 0.0 | 0.0 | 4 | |
Ψ(VL SC FI) P(.) | 22.0 | 0.0 | 0.0 | 4 |
Variables modelled: CC, VL, PD, DU, DF, DR, SC, FS plus YP in Year 5. See Table 1 for explanation of model variables. Akaike’s Information Criterion corrected for small sample size and relative to the best model (∆AICc), Akaike weights (w) and number of model parameters (k) are presented.
AModels with strong support.
To construct models, we first assessed whether detection probability was constant or varied throughout the survey season (Ψ(.), P(.) vs Ψ(.), P(t)) or with fire severity. Then we added site covariates to the model with the most support. We analysed each year separately based on pooled data for the transects encompassing each site. We opted for separate analyses over a multi-season framework because we were interested in the influence of covariate data on temporal occupancy patterns as the fire area recovered.
Eight site covariates were included in the models: (1) pig damage; (2) vegetation structure; (3) canopy cover; (4) distance to unburnt canopy; (5) distance to edge of fire; (6) distance to closest creek; (7) sedge cover; and (8) fire severity. For the 2020 model set (year five), the additional variable of number of years of pig damage was included.
We estimated the abundance of quokkas in the surveyed habitat by multiplying the faecal pellet count for each site by the detection probability generated by the highest ranked occupancy model. These abundance estimates are not intended to be extrapolated outside of the surveyed area.
Results
Factors influencing occupancy
In all years surveyed, fire severity, sedge cover, vegetation structure and pig damage had the strongest influence on the probability of occupancy of post-fire habitat by quokkas (Table 2). Distance to unburnt canopy and distance to riparian systems were also important variables for the first and third years following the fire. In the first year following the fire, distance to fire edge was important.
The probability of occupancy of habitat by quokkas in all years of the survey was dependent on the severity of fire that the sites had experienced. Sites with some canopy left unburnt were occupied by quokkas more frequently and earlier than areas with scorched or defoliated canopy (Table 3). Sites within the fire scar where canopy had been retained were, on average, occupied within 2.5 years, and by 2020 had an occupancy estimate of 0.85. Sites with a mix of scorched canopy and unburnt canopy were, on average, occupied within 2.8 years, and by 2020 had an occupancy estimate of 0.65. Sites where the canopy had been completely scorched were, on average, occupied within 3.8 years, and by 2020 had an occupancy estimate of 0.64. Sites defoliated by the fire were occupied within 4.7 years, but the occupancy estimate for these sites in 2020 was only 0.24 (Fig. 2).
Variable | Year | P | t | Occupied | Unoccupied | ||
---|---|---|---|---|---|---|---|
Mean (s.e.) | Maximum | Mean (s.e.) | Maximum | ||||
Fire severity | 1 | 0.00 | 6.2 | 1.1 (0.2) | 4.0 | 3.1 (0.2) | 6.0 |
3 | 0.00 | 5.5 | 1.7 (0.2) | 6.0 | 3.4 (0.3) | 6.0 | |
5 | 0.00 | 2.9 | 2.0 (0.2) | 6.0 | 3.1 (0.3) | 6.0 | |
Distance to unburnt canopy | 1 | 0.00 | 4.3 | 12.6 (14.2) | 183.2 | 1237.9 (204.8) | 11 249.8 |
3 | 0.00 | 4.7 | 219.9 (79.7) | 530.5 | 1485.1 (274.3) | 11 249.8 | |
5 | 0.00 | 3.2 | 428.7 (121.6) | 4826.8 | 1334.0 (290.0) | 11 249.8 | |
Distance to fire edge | 1 | 0.00 | 4.3 | 528.9 (148.3) | 4334.3 | 2362.8 (297.4) | 12 706.2 |
3 | 0.04 | 2.1 | 1291.4 (251.1) | 12 706.2 | 2173.1 (351.8) | 11 750.5 | |
5 | 0.41 | 0.8 | 1549.8 (264.6) | 12 706.2 | 1907.2 (357.3) | 11 750.5 | |
Distance to riparian | 1 | 0.00 | 3.3 | 57.7 (12.6) | 126.7 | 126.0 (28.8) | 2358.8 |
3 | 0.06 | 1.9 | 73.9 (8.6) | 323.4 | 148.6 (40.8) | 2358.8 | |
5 | 0.38 | 0.9 | 163.7 (13.2) | 541.2 | 221.9 (74.2) | 2358.8 | |
Sedge cover | 1 | 0.00 | −4.7 | 22.7 (1.2) | 43.7 | 15.6 (0.9) | 29.4 |
3 | 0.00 | −8.9 | 39.2 (1.4) | 59.4 | 17.6 (2.0) | 55.3 | |
5 | 0.00 | −16.4 | 44.6 (1.2) | 61.3 | 12.5 (1.6) | 45.8 | |
Vegetation structure | 1 | 0.00 | −9.3 | 3.4 (0.1) | 4.80 | 2.1 (0.1) | 4.4 |
3 | 0.00 | −8.1 | 3.7 (0.1) | 5.1 | 2.7 (0.1) | 4.8 | |
5 | 0.00 | 12.5 | 4.5 (0.1) | 6.8 | 2.5 (0.1) | 3.9 | |
Pig damage | 1 | 0.06 | 1.8 | 1.2 (0.5) | 22.1 | 8.6 (2.9) | 140.0 |
3 | 0.01 | 2.6 | 12.1 (5.5) | 150.3 | 253.1 (93.6) | 4604.6 | |
5 | 0.00 | 4.0 | 3.5 (1.3) | 81.9 | 481.0 (118.1) | 4718.2 |
Mean (standard error), Maximum, t-test and P-values are presented for occupied vs unoccupied sites. N = 126.
The effect of fire severity within the Northcliffe Fire area on habitat availability and occupancy estimates for quokkas in relation to (a) the relative proportion of sites within the fire scar for which each of the fire severity categories was recorded and (b) the average number of years taken for recolonisation of areas with different fire intensities. Occupancy estimates reported (Ψ) are for the fifth year post-fire and have been corrected using the detection probability estimated during data modelling. Sites with more than one category listed contained patches of both fire intensities. Canopy refers to areas where the overstorey canopy remained unburnt during and following the fire. Crown scorch is defined as the browning of leaves in the overstorey canopy that occurred as a result of heat generated from the fire. Defoliation refers to situations where the overstorey canopy experienced a complete loss of leaves during the fire.
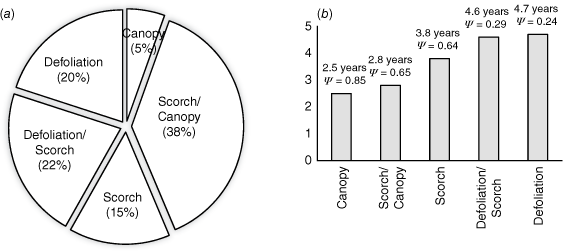
Distance to unburnt canopy was important for at least the first 3 years following the fire: all sites occupied by quokkas were within 183 m (mean 12.6 m ± s.e. 4.2) of unburnt canopy in the first year post-fire and within 530 m (mean 220.0 m ± s.e. 80.0) in the third year post-fire (Table 3). This variable was less important in the fifth year post-fire (Tables 2 and 3).
In the first year following the fire, all quokka activity was restricted to the edge of the fire scar or to a small number of internal sites where low densities of quokkas had been isolated from the edge in marginal, and in some cases unsuitable, habitat. Occupied sites were within 4.3 km (mean = 528.9 m ± s.e. 148.3) from the edge of the fire in the first year following the fire (Table 3). Distance to fire edge was not important in occupancy models for the third and fifth years post-fire (Table 2).
Only habitats within 323 m of riparian systems were occupied for the first 3 years following the fire, with an average distance of 57.7 m ± s.e. 12.5 in the first year and 73.9 m ± s.e. 8.6 in the third year (Table 3). In the fifth year post-fire, this variable was not significant in occupancy models (Table 2).
Sites containing a higher ground cover of sedges (Restionaceae, Cyperaceae or Anarthriaceae) were more likely to be occupied than sites where sedges were absent or less abundant. In the first year post-fire, occupied sites had a mean sedge cover of 22.7% ±s.e. 1.2 (Table 3). For occupied sites in the third and fifth years following fire, the mean sedge cover had increased to 39.2% ±s.e. 1.4 and 44.6% ±s.e. 1.2 respectively (Table 3). In particular, the presence of fine sedges such as Empodisma gracillimum and Anarthria prolifera seemed to be important. Other common sedge species included Lepidosperma effusum, Lepidosperma tetraquetrum and Gahnia decomposita.
The vegetation structure (number of vegetation layers) was an important explanatory variable for quokka occupancy in all survey years (Table 2). The vegetation structure was variable throughout the surveyed sites, largely due to components of the midstorey and overstorey that had survived the fire, and to the rate of recovery of understorey, midstorey and overstorey layers. Of the sites that were occupied in the first 5 years post-fire, 99% contained habitat with three vegetation layers or more. Habitats containing two or fewer vegetation layers were occupied only in the first year following the fire (mean 3.4 layers ±0.1), and by the fifth year post-fire, 71% of occupied sites contained >4 vegetation layers (mean 4.5 layers ±0.1, Table 3).
In total, 47 sites sustained pig damage in the first 5 years following the fire, with damage ranging from isolated diggings on less than 1 m2 through to extensive diggings up to 4718 m2. Quokkas were present in areas where pigs were active, but sites were occupied by quokkas only where the damage was less than 150 m2 (Table 3).
Feral pig activity was greatest 3 years post-fire, with a total of 15 479 m2 damaged across 28 sites compared with 766 m2 of damage across 26 sites in the first year (Fig. 3). Pig damage detected in the third year was an extension of damage recorded in the first year for 68% of sites. Damage was also recurrent within 77% of sites in the fifth year post-fire (Fig. 3).
Spatial and temporal occupancy
Prior to the Northcliffe fire, 92 sites within the fire scar were known to be occupied by quokkas, based on high confidence records from the 3 years prior to the fire. Of these sites, 38 were located within 1 km of the edge of the fire and 54 were located more than 1 km from the edge. In the first year following the fire, 17 (18%) of these sites were occupied by quokkas and only five of these sites were more than 1 km from the edge of the fire (Figs 4 and 5). Occupancy estimates increased most between years 1 and 3 for burnt sites both near the edge and in the core of the fire scar (Fig. 4). In the third year following the fire, 54% of burnt sites (core and edge) were occupied, increasing slightly to 59% in the fifth year. Occupancy remained stable over time at the 34 unburnt sites near the fire edge (Fig. 4).
Number of sites occupied by quokkas within and adjacent to the Northcliffe fire area in the first, third and fifth year post-fire. Core sites were internal refuge areas >1 km from the edge of the fire (N = 54), burnt edge sites were within 1 km of the edge of the fire (N = 38) and unburnt edge sites were sites adjoining the fire scar (N = 34). Occupancy estimates (Ψ) presented have been corrected using detection probabilities estimated during data modelling.
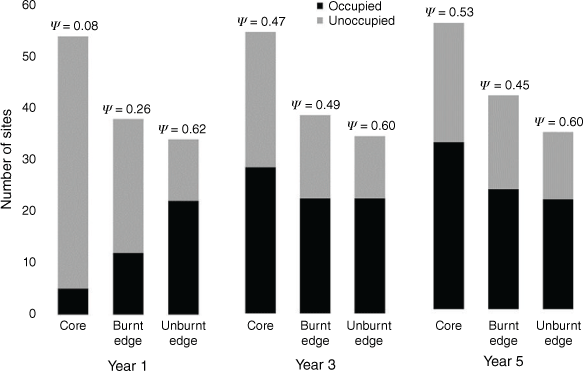
Occupancy of sites by quokkas in the first year, third year and fifth year following the Northcliffe fire. The year each site was first occupied by quokkas is shown, as well as significant riparian corridors mapped from aerial imagery and field truthing.
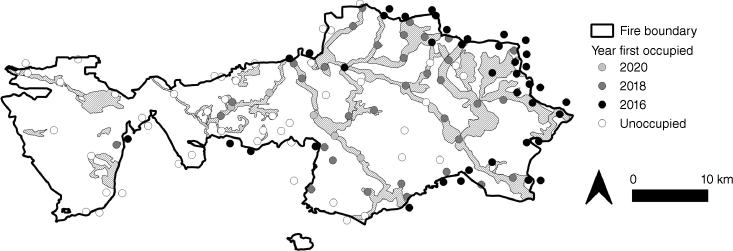
The marked increase in occupancy of burnt sites in the third year post-fire resulted from 33 additional sites becoming occupied, all within 12.7 km from the fire edge, and all within 7 km of sites that had been occupied in the first year post-fire. In the fifth year only five additional sites were occupied, and all were within 5 km of sites that were occupied in the third year. In all cases, newly occupied sites were linked by riparian corridors to previously occupied sites (Fig. 5). Within the fire scar, a total of 41 sites remained unoccupied in the fifth year post-fire and all of these sites were located in the core, southern and western parts of the fire, between 5 and 30 km from the closest occupied site (Fig. 5) in areas that had been severely affected (defoliated) by the fire.
Recovery to pre-fire abundance
Although occupancy estimates provide an indication of the spatial pattern of recolonisation, in many instances burnt sites were occupied by less than five individuals, and for this reason, a measure of abundance was also established for each of the sites based on Bain et al. (2014) and corrected for detection probability. In the first 12 months following the fire, 97 quokkas were estimated to be present within the Northcliffe fire scar (burnt sites only), and by the fifth year post-fire, this estimate had increased to 272 individuals (Fig. 6). It is estimated that approximately 594 individuals were present within the fire scar prior to the fire, based on the number of known occupied sites and the average density of quokkas in this area. Quokka recovery within the Northcliffe fire scar had subsequently reached approximately 46% of pre-fire abundance levels by the fifth year post-fire.
Linear projection of increasing quokka abundance based on data collected in the first, third and fifth year post-fire.
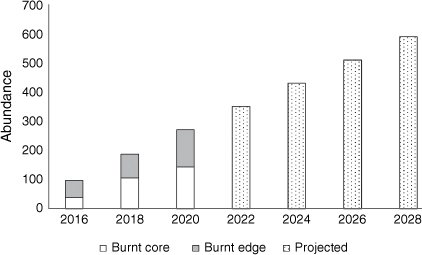
A linear projection based on the 5 years of data suggests that the abundance of quokkas will reach pre-fire levels 12 years post fire (Fig. 6). At year five, a linear relationship was the best fit for the data; however, quokka recolonisation/recovery patterns are likely to be affected by changes in the rate of recovery of the vegetation and the continuing availability of suitable habitat and habitat connectivity.
Discussion
The Northcliffe fire in south-western Australia resulted in substantial loss of vertical vegetation structure across a large area (98 000 ha), affecting extensive areas of quokka habitat and resulting in distances of up to 74 km separating occupied patches of habitat. The fire resulted in an 82% reduction in site occupancy and an 84% reduction in the abundance of quokka. Our results support our hypothesis that quokkas would take longer than 3 years to recolonise the more intensely burnt parts of this landscape. After 5 years, only 46% of the pre-fire abundance of quokkas had been reached and 41% of previously occupied habitat patches remained unoccupied, all of which were sites that had been defoliated during the fire. Sites where quokkas persisted occurred in parts of the landscape that had retained some vertical vegetation structure, contained an understorey dominated by sedge species and where damage by feral pigs was low. These findings have profound implications for the recovery of this species and the management of the region, particularly regarding the critical habitat.
Spatiotemporal recolonisation patterns
For the first year, few quokkas within the fire scar were greater than 1 km from the fire edge. In fire-prone forests burnt under extreme fire conditions, the presence of fire refugia has been shown to be limited to sheltered parts of the landscape with extremes in topography and wetness (Robinson et al. 2013; Leonard et al. 2014; Berry et al. 2015). In the Northcliffe fire area, the few occupied refugia coincided with deep, mesic riparian systems low in the profile, or perched wetland systems retaining high levels of summer moisture. These areas burnt less intensely than the surrounding forest and contributed to small habitat patches where canopy and midstorey vegetation and tiny pockets of understorey vegetation had been left unburnt by the fire. In most cases, these patches were less than 0.5 ha in size, and in all cases the abundance of survivors was low.
The abundance and spatial distribution of survivors strongly influences post-fire recovery following a major disturbance, in conjunction with the effect of the fire on the distribution of resources that are important to the species and the sensitivity of the species to the disturbance (Robinson et al. 2013; Banks et al. 2017; Meddens et al. 2018). The distinction between recolonisation from in situ survivors and recolonisation from the fire edge as starting points for recovery therefore has implications for understanding ongoing demographic and metapopulation processes. The first 3 years of population recovery were predominantly restricted to topographic refugia. Spatial recolonisation patterns were likely to have been limited by the low availability of refugia within the fire scar and the low abundance of individuals persisting in these. Although recovery from in situ subpopulations was possible, post-fire recolonisation from the fire edge was more likely contributing to the bulk of the observed recovery patterns, given the connection to occupied sites around the eastern and southern fire boundary, where likely source animals were located (Fig. 5).
The presence of fire refugia contributed to the availability of resources for animals residing within and moving through the fire scar. These refugia were even more valuable when considered in the context of the relatively small distances moved from unburnt canopy by quokkas in the post-fire environment. Despite quokkas being able to move significant distances (up to 10 km in a night) in the southern forest (Bain et al. 2020), the average distance from refugia (unburnt canopy) at which quokkas were detected was 13 m (maximum 183 m) in the first year following the fire. These small distances are likely to be associated with perceived safety, stress levels and/or predator pressure, and are consistent with previous studies for this species in the southern forest (Bain et al. 2016). The increase in the average distance from refugia in the fifth year to 429 m (maximum 4826.8 m) corresponds with an increase in the availability of shelter vegetation in the surrounding landscape.
The sites that remained uncolonised were those that had been defoliated during the fire. These sites were still mostly absent of green vegetation in the first year, and the understorey vegetation recovery was slower than in sites that had been less intensely burnt, possibly due to increased soil temperatures and lower availability of seed and lignotuber material in the soil (Auld and Bradstock 1996; Penman et al. 2008; Nicholson et al. 2017). The midstorey and overstorey components of living vegetation were completely lost in these areas, and in the karri forest, these elements of the vegetation structure may take more than 10 years to re-establish (Bradshaw and Rayner 1997).
Vegetation structure is important for quokkas in this region (Bain et al. 2015). Multiple vegetation layers provide quokkas shelter from the elements and protection from predators. Vegetation structure is likely to vary in the post-fire environment as a result of topography, soil temperature, weather and the varying growth rates of floristic species present (Lentile et al. 2007; Littlefield 2019). In the southern forest, the re-establishment of vegetation structure occurs most rapidly in areas with topographic moisture, such as within riparian systems. Riparian systems also provide greater ground-level shelter resources for quokkas in the post-fire environment due to the presence of unburnt vegetation and shading associated with rocks, steep banks and fallen trees. Sedges within these systems are associated with moister conditions and are often the first element of the vegetation to recover following fire, rapidly forming a dense understorey layer that provides increased cover.
The rapid regeneration of a dense sedge understorey layer also reduces the availability of exposed substrate to feeding and wallowing behaviours of the introduced feral pig. The wallowing, digging and feeding behaviours of this species contributed to a total of 26 044 m2 of area with altered soil structure, physical disturbance of vegetation that was recovering from the fire and removal of propagules. This disturbance was sustained across multiple years and in most cases was an extension of damage recorded in the first year. This suggests that the first year post-fire was the most important in allowing the pigs access to the freshly disturbed areas.
Landscape-scale management of metapopulation structure
This project has demonstrated the complexity of recovery of a threatened species in an environment that has been impacted by an intense and large bushfire. The rate of recovery of quokkas in this landscape has been slow, and despite 5 years of vegetation recovery, the quokka subpopulation has yet to reach pre-fire abundance and distribution. The disruption to the metapopulation is likely to be a conservation and management issue for at least 12 years post fire and will need careful consideration during planning to ensure that any additional disturbance activities do not contribute to additional habitat fragmentation.
There is pressure for the land manager to undertake prescribed burning surrounding and within the Northcliffe fire scar to ensure that a bushfire of the scale and severity of the 2015 fire does not occur again. Prescribed burning is necessary to reduce the risk of such broadscale and intense bushfires; however, the current management paradigm in the southern forest of block-scale prescribed burning adjacent to bushfire scars within 2–3 years has the potential to be counter-productive to the recovery of the quokka and other species through the exacerbation of habitat fragmentation, displacement of threatened species, loss or disruption of metapopulation function and landscape-level population declines. This can be mitigated through explicit management of important corridors for movement, retention of patches of habitat in a matrix of burnt and unburnt vegetation that creates feeding, refuge and movement opportunities, proactive management of threats such as introduced predators and feral pigs and planning for spatial and/or temporal separation of burnt areas that facilitate effective recolonisation and recovery at a landscape scale prior to introducing additional disturbance.
In addition, prioritising the protection of critical habitat elements (e.g. potential fire refugia and important movement corridors) during a bushfire emergency may help to mitigate the detrimental effects of large fires (Lindenmayer et al. 2013; Robinson et al. 2013; Meddens et al. 2018). Statistical landscape models that predict the occurrence of potential fire refugia from landscape level variables, such as vegetation type, seral stage, fuel loads, climatic conditions and topography, may help land managers to identify and protect areas of the landscape of high conservation value (Mackey et al. 2012; Leonard et al. 2014).
Identification and protection of critical habitat elements, including managing their spatial and temporal configuration, composition and structure, is becoming increasingly important for effective conservation of threatened species under predictions of climate change (Gibson et al. 2010) and the associated predicted increase in frequency of large bushfires (Clarke et al. 2011; Abram et al. 2021). In this context, there is also an increasing need for a formalised approach to fire management and species conservation across the continent. In particular, there is a need for collaboration and consistency in fire management for ecological outcomes, the spatiotemporal management of habitat elements and the approach to monitoring and evaluation of management effectiveness across the geographical extent of those groups of species likely to be most vulnerable to fire regime effects.
Acknowledgements
In fond memory of Merril Halley, who was passionately committed to this project and contributed significant enthusiasm, expertise and field support prior to her unexpected and premature passing. This project was coordinated by Python Ecological Services in partnership with World Wildlife Fund Australia and completed in collaboration with the Western Australian Department of Biodiversity, Conservation and Attractions, Parks and Wildlife Service. We thank Brad Barton, Ian Wilson, Mark Virgo, Julia Wayne, Janine Liddelow and Alison McGilvray for sharing technical expertise and providing input throughout the planning and operational phases of the project, and Nic Slatter, Glenn Freeman, Michael Sawyer and Roslyn Burnside for assisting with field surveys. We also thank the anonymous reviewers for their contributions to the improvement of this manuscript.
References
Abram NJ, Henley BJ, Sen Gupta A, Lippmann TJR, Clarke H, Dowdy AJ, Sharples JJ, Nolan RH, Zhang T, Wooster MJ, Wurtzel JB, Meissner KJ, Pitman AJ, Ukkola AM, Murphy BP, Tapper NJ, Boer MM (2021) Connections of climate change and variability to large and extreme forest fires in southeast Australia. Communications Earth & Environment 2, 8.
| Crossref | Google Scholar |
Auld TD, Bradstock RA (1996) Soil temperatures after the passage of a fire: Do they influence the germination of buried seeds? Australian Journal of Ecology 21, 106-109.
| Crossref | Google Scholar |
Bain K, Wayne A, Bencini R (2014) Overcoming the challenges of measuring the abundance of a cryptic macropod: is a qualitative approach good enough? Wildlife Research 41, 84-93.
| Crossref | Google Scholar |
Bain K, Wayne A, Bencini R (2015) Risks in extrapolating habitat preferences over the geographical range of threatened taxa: a case study of the quokka (Setonix brachyurus) in the southern forests of Western Australia. Wildlife Research 42, 334-342.
| Crossref | Google Scholar |
Bain K, Wayne A, Bencini R (2016) Prescribed burning as a conservation tool for management of habitat for threatened species: the quokka (Setonix brachyurus) in the southern forests of Western Australia. International Journal of Wildland Fire 25, 608-617.
| Crossref | Google Scholar |
Bain K, Wayne AF, Bencini R (2020) Spatial ecology of the quokka (Setonix brachyurus) in the southern forests of Western Australia: implications for the maintenance, or restoration, of functional metapopulations. Australian Mammalogy 42, 38-47.
| Crossref | Google Scholar |
Banks SC, McBurney L, Blair D, Davies ID, Lindenmayer DB (2017) Where do animals come from during post-fire population recovery? Implications for ecological and genetic patterns in post-fire landscapes. Ecography 40, 1325-1338.
| Crossref | Google Scholar |
Berry LE, Driscoll DA, Stein JA, Blanchard W, Banks SC, Bradstock RA, Lindenmayer DB (2015) Identifying the location of fire refuges in wet forest ecosystems. Ecological Applications 25, 2337-2348.
| Crossref | Google Scholar |
Bradshaw FJ, Rayner ME (1997) Age structure of the karri forest: 1. Defining and mapping structural development stages. Australian Forestry 60, 178-187.
| Crossref | Google Scholar |
Bradstock RA (2008) Effects of large fires on biodiversity in south- eastern Australia: disaster or template for diversity? International Journal of Wildland Fire 17, 809-822.
| Crossref | Google Scholar |
Burrows N, McCaw L (2013) Prescribed burning in southwestern Australian forests. Frontiers in Ecology and the Environment 11, 26-34.
| Crossref | Google Scholar |
Clarke HG, Smith PL, Pitman AJ (2011) Regional signatures of future fire weather over eastern Australia from global climate models. International Journal of Wildland Fire 20, 550-562.
| Crossref | Google Scholar |
Department of Biodiversity, Conservation and Attractions (2023) DBCA 3 Year Burn Options Program (DBCA-009). Available at https://catalogue.data.wa.gov.au/dataset/dbca-3-year-indicative-burn-program
Department of Water and Environmental Regulation (2018) Hydrography, Linear (Hierarchy) Dataset (DWER-031) Available at https://catalogue.data.wa.gov.au/dataset/598ba78e-e486-4731-a8f4-235ce5661ead/resource/9908c7d1-7160-4cfa-884d-c5f631185859/download/gda2020_db.gisdba.hydrography_hierarchy_dow.met
Driscoll DA, Armenteras D, Bennett AF, Brotons L, Clarke MF, Doherty TS, Haslem A, Kelly LT, Sato CF, Sitters H, Aquilué N, Bell K, Chadid M, Duane A, Meza-Elizalde MC, Giljohann KM, González TM, Jambhekar R, Lazzari J, Morán-Ordóñez A, Wevill T (2021) How fire interacts with habitat loss and fragmentation. Biological Reviews 96, 976-998.
| Crossref | Google Scholar |
Gibson L, McNeill A, de Tores P, Wayne A, Yates C (2010) Will future climate change threaten a range restricted endemic species, the quokka (Setonix brachyurus), in southwest Australia? Biological Conservation 143, 2453-2461.
| Crossref | Google Scholar |
Hines JE (2006) ‘PRESENCE- Software to estimate patch occupancy and related parameters.’ (USGS-PWRC) Available at http://www.mbr-pwrc.usgs.gov/software/presence.html
Hopper SD (1979) Biogeographical aspects of speciation in the southwest Australian flora. Annual Review of Ecology and Systematics 10, 399-422.
| Crossref | Google Scholar |
Howard T, Burrows N, Smith T, Daniel G, McCaw L (2020) A framework for prioritising prescribed burning on public land in Western Australia. International Journal of Wildland Fire 29, 314-325.
| Crossref | Google Scholar |
Lentile LB, Morgan P, Hudak AT, Bobbitt M J, Lewis SA, Smith AMS, Robichaud PR (2007) Post-fire burn severity and vegetation response following eight large wildfires across the Western United States. Fire Ecology 3, 91-108.
| Crossref | Google Scholar |
Leonard SWJ, Bennett AF, Clarke MF (2014) Determinants of the occurrence of unburnt forest patches: potential biotic refuges within a large, intense wildfire in south-eastern Australia. Forest Ecology and Management 314, 85-93.
| Crossref | Google Scholar |
Lindenmayer DB, Blanchard W, McBurney L, Blair D, Banks SC, Driscoll D, Smith AL, Gill AM (2013) Fire severity and landscape context effects on arboreal marsupials. Biological Conservation 167, 137-148.
| Crossref | Google Scholar |
Littlefield CE (2019) Topography and post-fire climatic conditions shape spatio-temporal patterns of conifer establishment and growth. Fire Ecology 15, 34.
| Crossref | Google Scholar |
Macfarlane C, Coote M, White DA, Adams MA (2000) Photographic exposure affects indirect estimation of leaf area in plantations of Eucalyptus globulus Labill. Agricultural and Forest Meteorology 100, 155-168.
| Crossref | Google Scholar |
Macfarlane C, Hoffman M, Eamus D, Kerp N, Higginson S, McMurtrie R, Adams M (2007) Estimation of leaf area index in eucalypt forest using digital photography. Agricultural and Forest Meteorology 143, 176-188.
| Crossref | Google Scholar |
MacKenzie DI, Bailey LL (2004) Assessing the fit of site-occupancy models. Journal of Agricultural, Biological and Environmental Statistics 9, 300-318.
| Crossref | Google Scholar |
McKenzie NL, Burbidge AA, Baynes A, Brereton RN, Dickman CR, Gordon G, Gibson LA, Menkhorst PW, Robinson AC, Williams MR, Woinarski JCZ (2007) Analysis of factors implicated in the recent decline of Australia’s mammal fauna. Journal of Biogeography 34, 597-611.
| Crossref | Google Scholar |
Mackey B, Berry S, Hugh S, Ferrier S, Harwood TD, Williams KJ (2012) Ecosystem greenspots: identifying potential drought, fire, and climate-change micro-refuges. Ecological Applications 22, 1852-1864.
| Crossref | Google Scholar |
Meddens AJH, Kolden CA, Lutz JA, Smith AMS, Cansler CA, Abatzoglou JT, Meigs GW, Downing WM, Krawchuk MA (2018) Fire Refugia: What Are They, and Why Do They Matter for Global Change? BioScience 68, 944-954.
| Crossref | Google Scholar |
Nicholson Á, Prior LD, Perry GLW, Bowman DMJS (2017) High post-fire mortality of resprouting woody plants in Tasmanian Mediterranean-type vegetation. International Journal of Wildland Fire 26, 532-537.
| Crossref | Google Scholar |
Nimmo DG, Avitabile S, Banks SC, Bliege-Bird R, Callister K, Clarke MF, Dickman CR, Doherty TS, Driscoll DA, Greenville AC, Haslem A, Kelly LT, Kenny SA, Lahoz‐Monfort JJ, Lee C, Leonard S, Moore H, Newsome TM, Parr CL, Ritchie EG, Schneider K, Turner JM, Watson S, Westbrooke M, Wouters M, White M, Bennett AF (2019) Animal movements in fire-prone landscapes. Biological Reviews 94, 981-998.
| Crossref | Google Scholar |
Penman TD, Binns D, Allen R, Shiels R, Plummer S (2008) Germination responses of a dry sclerophyll forest soil-stored seedbank to fire related cues. Cunninghamia 10, 547-555.
| Google Scholar |
Robinson NM, Leonard SWJ, Ritchie EG, Bassett M, Chia EK, Buckingham S, Gibb H, Bennett AF, Clarke MF (2013) REVIEW: Refuges for fauna in fire-prone landscapes: their ecological function and importance. Journal of Applied Ecology 50, 1321-1329.
| Crossref | Google Scholar |
Spencer PBS, Bain K, Hayward MW, Hillyer M, Friend JAT (2019) Persistence of remnant patches and genetic loss at the distribution periphery in island and mainland populations of the quokka. Australian Journal of Zoology 67, 38-50.
| Crossref | Google Scholar |