Impact of water stress during reproductive development on seed dormancy in Bromus diandrus Roth and Lolium rigidum Gaudin
Zarka Ramiz

A
Abstract
Bromus diandrus Roth and Lolium rigidum Gaudin are important weeds of cereal-based cropping systems of Australian agriculture. Adaptation to environmental stresses, protracted seed germination and herbicide resistance have made these weeds serious threats to crop production.
Studies were undertaken to determine the impact of moisture stress during reproductive development on the extent of seed dormancy and the expression of genes involved with gibberellic acid and abscisic acid synthesis.
A pot study was undertaken at two locations, with two populations each of B. diandrus and L. rigidum. Water stress was applied from either the GS31 or GS60 stage until seed maturation, along with a well-watered treatment. Seeds of stressed vs well-watered treatments were assessed for seed dormancy and the expression of ABA1 and GA20ox genes using quantitative polymerase chain reaction.
The seeds from GS31 treatment, where plants experienced the longest water stress, were most dormant in both weed species. Water stress treatments altered the expression of the GA20ox gene, which was correlated with the level of dormancy in seeds of B. diandrus and L. rigidum.
This investigation has provided clear evidence of the impact of water stress on seed dormancy and on expression of genes involved in regulating seed dormancy in these weed species.
As spring rainfall in the Australian agricultural landscape is highly variable, weeds are likely to experience variable levels of water stress during reproductive development, which in turn is likely to influence seed dormancy, weed seedling emergence and effectiveness of pre-sowing weed management next season.
Keywords: ABA1, Annual Ryegrass, crop–weed competition, dormancy, GA20ox, genetic variation, Great Brome, seed germination, water stress.
Introduction
Weed seed dormancy is associated with the development of persistent seed banks (Ghersa et al. 1997). Seed dormancy also causes difficulties in predicting the timing of seedling emergence and can reduce the effectiveness of weed control measures (Ghersa and Holt 1995). Both genetic factors and the maturation environment experienced by the parent plants play important roles in setting the level of seed dormancy (Fenner and Thompson 2005). In particular, the maturation environment experienced by the parent plant has been shown to play a fundamental role in determining seed dormancy in weeds (Steadman et al. 2003; Steadman 2004). Several environmental factors, such as photoperiod, water availability, temperature, light, and nutrient supply, are known to influence seed quality, including seed size, dormancy, and germinability (Fenner 1991; Hilhorst and Toorop 1997; Baskin and Baskin 1998; Gutterman 2000). Among these, water availability is an important limiting factor that effects plant growth and reproductive development and often prevents the plants from reaching the maximum growth potential in rain-fed environments (Patterson 1995; Jaleel et al. 2009). Inadequate soil moisture affects crop–weed competition significantly and generally weeds dominate under stress due to greater plasticity as compared to the crops (Chauhan and Mahajan 2014).
Spring rainfall in southern Australia can be quite variable, and this variation in rainfall pattern and increased aridity consistent with a warming climate, has the potential to alter weed distribution and their impact on crop production (Ramesh et al. 2017). Therefore, it is important to understand how water stress experienced by weeds during seed development will influence the expression of seed dormancy in important weeds. Low spring rainfall during the reproductive phase of weeds could also have an impact on the quality of weed seeds, in particular their dormancy status. Sharif-Zadeh and Murdoch (2000) showed that seed dormancy of Cenchrus ciliaris L. increased when water stress was present during seed maturation. In contrast, water stress applied at the onset of flowering until completion of flowering resulted in a significant reduction in plant biomass, seed production and quick dormancy release in wild radish (Eslami et al. 2010).
Previous studies have shown that early maturing seeds have higher levels of seed dormancy as compared to the late maturing seeds (Barton 1962; Frost 1971; Pourrat and Jacques 1975; Ceccato et al. 2015). This variation is partially facilitated by maternal control of seed coat properties (Kendall et al. 2011; MacGregor et al. 2015). For example, variations in the seed coat thickness have been reported in the genus Chenopodium (Ceccato et al. 2015). However, in Arabidopsis, the maternal environment is known to modify the contents of abscisic acid (ABA) and gibberellic acid (GA) in mature seeds, which affects physiological behaviour of the embryo (Footitt et al. 2011). Among the different plant hormones, ABA and GA are considered vital for the regulation of seed dormancy and germination (Tuan et al. 2018). In mature seeds and buds, the biosynthesis of ABA has been shown to be regulated by ABA1, NCED3 and ABA2 genes (Arora et al. 2003; Ruttink et al. 2007; Wang et al. 2016). GA releases seed dormancy and helps in seed germination, and its biosynthesis is regulated by GA20-oxidase (GA20ox) and GA3-oxidase (GA3ox) genes (Tuan et al. 2018). As ABA1 and GA20ox genes are involved in the initial steps of biosynthesis pathways of these plant hormones, they are known to have a significant impact on the expression of seed dormancy induction and release (Arora et al. 2003; Tuan et al. 2018).
Bromus diandrus Roth (Great Brome) is a winter annual weed commonly found in agricultural areas characterised by cool wet winters and hot dry summers (Kon and Blacklow 1988) and is a serious weed of crops in South Australia (Kleemann and Gill 2006). Both B. diandrus and L. rigidum can be serious weeds of crop production in other countries with a Mediterranean climate. For example, B. diandrus has become a problematic weed in northeastern Spain since the adoption of no-till farming (Royo-Esnal et al. 2018). Lolium rigidum Gaudin (Annual Ryegrass) is regarded as the most problematic weed prevalent in grain growing regions of South Australia (Kloot 1983). L. rigidum is also one of the most common weed species in winter cereals in Spain, where it infests more than 6 million hectares of winter cereal crops (Cirujeda and Taberner 2009). Both weed species can be highly competitive with crops, including wheat, and can also be contaminants of harvested grains (Gill and Blacklow 1985; Gill et al. 1987; Gill 1996; Steadman et al. 2004a; Llewellyn et al. 2016). The life cycle of both weeds is similar to many annual grass species in southern Australia; they germinate in autumn–winter, grow rapidly during spring and set seeds in summer (Steadman et al. 2003; Kleemann and Gill 2006). Upon maturation seeds are mostly dormant, which prevents fatal germination during infrequent rainfall events that occur during summer in Australia (Steadman et al. 2004a).
Lolium rigidum has high genetic variability (Owen et al. 2007), which can lead to large variations in germination responses of populations. Previous studies by Steadman et al. (2004b) on L. rigidum confirmed that seed dormancy is affected by moisture and temperature stress; seeds produced under cool temperatures and moisture stress were quick to lose dormancy as compared to the seeds collected from well-watered plants. None of the previous studies examined the impact of moisture stress on seed dormancy variation and expression of genes responsible for controlling this variation in B. diandrus and L. rigidum. At present it is unclear what effect water stress during reproductive development will have on seed dormancy in B. diandrus and L. rigidum. Therefore, studies were undertaken with the following objectives:
Materials and methods
Seed collection
Mature seeds of two populations each of Lolium rigidum and Bromus diandrus were collected in December 2019 from crop fields in South Australia. The L. rigidum populations (RAC1 and RAC3) were collected from a single farm at Roseworthy, South Australia (34.524807°S, 138.686362°E) and B. diandrus populations were collected from two different farms located near the towns of Mallala (34.4379°S, 138.5099°E) and Riverton (34.1584°S, 138.7477°E), South Australia. The collected seeds were oven dried, cleaned and stored in a laboratory until used in the experiments (April to November 2020).
Effect of water stress during reproductive development on seed dormancy
This experiment was laid out in completely randomised design and replicated at two locations, Roseworthy campus (R) (34.5274°S, 138.6881°E) and the Waite campus of The University of Adelaide (W) (34.9688°S, 138.6339°E) during April to November 2020. These two experimental sites are 50 km apart. The experiment was replicated at these two sites to assess the consistency of the effect of water stress treatments on seed dormancy of two populations each of L. rigidum and B. diandrus. The factorial combinations of weed populations and water stress treatments were replicated three times at each location.
Weed plants were exposed to water stress by ceasing irrigation at the GS31 (first node) and GS60 stage (flowering). The control treatment, in which plants were irrigated regularly (approximately 550 mL/pot) to prevent water stress, was included to compare the effects of water stress. The control pots were maintained at the field capacity throughout the experiment whereas soil water content of water stress treatments was allowed to decline to 25% of the field capacity (FC) based on regular assessment of pot weight. Thereafter, pots in the water stress treatments were maintained close to 25% FC by regular watering. The field capacity was determined using the method described by Steadman et al. (2004b). Briefly, the pots containing dry potting mixture were weighed and then watered until water started to drain at the bottom of the pots. Pots were then allowed to drain for 6 h and then reweighed to determine FC.
Seeds of L. rigidum and B. diandrus populations (three replicates/population and stress treatment) were placed in pots (8 L capacity with 250 mm diameter) containing a potting mix (Handreck and Black 2002; Boutsalis et al. 2012). When seedlings reached the two leaf stage, plant density was reduced to five plants/pot for L. rigidum and three plants/pot for B. diandrus. The pots were kept outdoors (May–June) under natural environmental conditions (the average day/night temperature in Roseworthy and Waite for May–June is approximately 15/6°C (Bureau of Meteorology 2022)) and well-watered until the start of water stress treatments. When the plants reached the GS31 (first node) stage, all the pots were moved into the glasshouse (August) to prevent rainfall affecting water stress and to ensure consistency of temperature and light conditions for all the treatments. The glasshouses used at both the locations were illuminated by sunlight, but day/night temperature was set at 20/10°C. Water content for the first stress treatment (GS31) was allowed to decline to 25% of the FC and then maintained at this level until maturity. The other pots were irrigated regularly and maintained close to the FC until the plants reached GS60 (flowering stage) and the second water stress treatment was imposed. Plants allocated to water stress treatments were weighed twice a week and the soil moisture raised to 25% of the FC. Throughout the experiment, pots of the no stress treatment (control) were well-watered and maintained close to the FC.
Harvest maturity was defined as the loss of green colour of stems and seeds. At maturity, seeds of each stress treatment from the two study sites were collected and placed in separate paper bags. The seeds were cleaned and stored in a laboratory at standard temperature conditions of 20/10°C until used for seed dormancy and gene expression studies (December 2020 to August 2021). After cleaning, seeds were counted to determine seed production and samples of 100 seeds were weighed to determine seed mass.
Seeds of different water stress treatments were assessed for seed dormancy (germinability) every 2 months from December 2020 to August 2021. Seeds (25) were placed in 15 cm diameter Petri dishes lined with Whatman No 1 filter paper; there were three replicates of each treatment. The filter paper was moistened with 5 mL of distilled water and the 25 seeds spread over the surface. The Petri dishes were placed in an incubator set at 20/10°C, with 12 h photoperiod for L. rigidum. As germination in B. diandrus seeds can be inhibited by exposure to light, Petri dishes containing its seeds were covered with aluminium foil to ensure complete darkness. Seed germination was assessed after 14 days and the experiment was terminated.
Expression of target genes associated with seed dormancy
After a careful review of the literature, we focused on the expression of the genes ABA1 and GA20ox in seeds of B. diandrus and L. rigidum and its relationship with changes in seed dormancy during the after-ripening period.
At the same time as the investigation of seed dormancy in water stress treatments, weed seeds were also used to extract the RNA and determine the expression of target genes (ABA1 and GA20ox). For each treatment there were three replicates of 25 seeds/population of L. rigidum and 15 seeds/population of B. diandrus tested at 30, 90 and 150 days after maturity to determine changes in the seed dormancy and gene expression levels during the after-ripening period. Quantitative polymerase chain reaction (qPCR) analysis of the expression of housekeeping genes (αTubulin and ELF) and target genes (ABA1 and GA20ox) was conducted at each assessment to determine the pattern of candidate gene expression during dormancy maintenance and release.
RNA extraction and cDNA synthesis from seeds
The RNA extraction and complementary DNA (cDNA) synthesis was performed using the method described by Ramiz et al. (2022a, 2022b).
Gene expression for seed dormancy was analysed via quantitative PCR (qPCR) using similar methods to that described by Malone et al. (2016); Adu-Yeboah et al. (2020). A Sensimix (Bioline, Meridian Bioscience) assay using Dual-Labelled BHQ FRET probes (Bioresearch Technologies, Petaluma, CA, USA) was used. Primers and probes for the candidate genes ABA1 and GA20ox and control genes αTubulin and ELF were designed using Real Time Design qPCR assay design software (Bioresearch Technologies). cDNA templates were amplified in a 10 μL reaction mixture containing 5 μL of Sensimix (Bioline, Meridian Bioscience), 0.4 μL of each target and control primers, and 0.1 μL of each target and control probe, 1.5 μL of H2O and 2.5 μL of cDNA. Reactions were run on a Rotor Gene 6000 real-time thermal cycler with the following parameters: 3 min at 95°C, followed by 45 cycles of 15 s at 95°C and 16 s at 60°C. Primer efficiencies were calculated and found to range from >96% to <99%. Each qPCR reaction containing the cDNA from the control (no stress), water stress at the GS31 and GS60 stage from both the populations of L. rigidum and B. diandrus was run with a gene of interest (ABA1 and GA20ox) and two control genes (αTubulin and ELF). This protocol was repeated with seeds at 90 and 120 days after maturity.
Statistical analysis
An analysis of variance (ANOVA) was performed on the data for weed seed production, seed mass and germination with GenStat (edition 19, VSN International, https://vsni.co.uk/). As the trial location cannot be replicated, treatments were nested within a location. Prior to the combined analysis, the homogeneity of the variance was assessed using Bartlett’s test of homogeneity. The distribution of the data was checked from the normal probability plot of the residuals. All regression analyses were performed with the software GraphPad Prism version 9 (GraphPad Software, San Diego, CA).
For gene expression analysis, the candidate gene runs for qPCR were compared with both housekeeping genes (αTubulin and ELF) to normalise the data. The average of the CT values of two control genes was used and a modified version of the ΔCT (2 = −ΔCT) method was used to analyse data from the quantitative PCR (Livak and Schmittgen 2001; Gaines et al. 2010). The calculated CT values were then used to run ANOVA. A correlation analysis between the gene expression levels and seed germination (%) of both the weed species was performed to determine the relationship between seed germination and gene expression levels during the after-ripening periods.
Results
Impact of water stress on seed production and seed mass
A significant (P < 0.001) impact of water stress treatments on seed production and seed mass was observed in B. diandrus (Table 1) and L. rigidum (Table 2). As expected, plants growing without water stress (control) produced more seeds and seeds of greater mass. Seed production in B. diandrus was much more sensitive to water stress imposed at GS31 stage than at GS60 (panicle emergence) (Table 1). Conversely, seed mass was more responsive to water stress imposed at GS60 than GS31. The response of seed number and seed mass to water stress was similar in L. rigidum but the impact was lower than observed in B. diandrus.
Water stress timing | Seeds per plant | Seed mass (g/100 seed) | |
---|---|---|---|
Control (no stress) | 870.9 | 1.81 | |
GS31 | 509.4 (41.5) A | 1.50 (17.3) | |
GS60 | 800.2 (8.1) | 1.25 (31.1) | |
P-value | <0.001 | <0.001 | |
l.s.d. (P = 0.05) | 1.54 | 0.015 |
Water stress timing | Seeds per plant | Seed mass (g/100 seed) | |
---|---|---|---|
Control (no stress) | 331.9 | 1.004 | |
GS31 | 250.6 (24.5) A | 0.872 (13.1) | |
GS60 | 279.1 (15.9) | 0.824 (17.9) | |
P-value | <0.001 | <0.001 | |
l.s.d. (P = 0.05) | 1.33 | 0.035 |
Effect of water stress treatments on seed dormancy
As the main aim of this study was to investigate the effect of water stress on seed dormancy and expression of key genes regulating dormancy, ANOVA was performed separately for data collected at 30, 60 and 90 days after maturity (DAM). As there was no site × timing × population interaction, data for the two sites were pooled. The water stress treatments had a significant impact on seed dormancy in B. diandrus populations. Plants grown in well-watered conditions produced seeds with a lower level of dormancy as compared to plants that experienced water stress at the earliest stage of development (GS31) (P < 0.05). At 30 DAM, some germination was observed in the control (20%) and water stress imposed at the flowering stage (11%). However, there was no seed germination observed in plants exposed to water stress at GS31 (Fig. 1). During the after-ripening period, there was a gradual increase in seed germination in the control as well as water stress treatments. Significant differences were observed in seed germination between water stress and control treatments until 90 DAM (P < 0.05). However, at 150 DAM, seed germination in both the water stressed and control treatments was similar in both populations of B. diandrus (P = 0.08; Fig. 1).
Germination pattern of two B. diandrus ((a) Mallala (b) Riverton) populations under water stress and no stress treatments. The error bars show ± s.e., and the symbols show significant differences at P < 0.05 (*) or P < 0.01 (**). As there was no site × population × stress timing interaction, the results for the two sites were pooled.
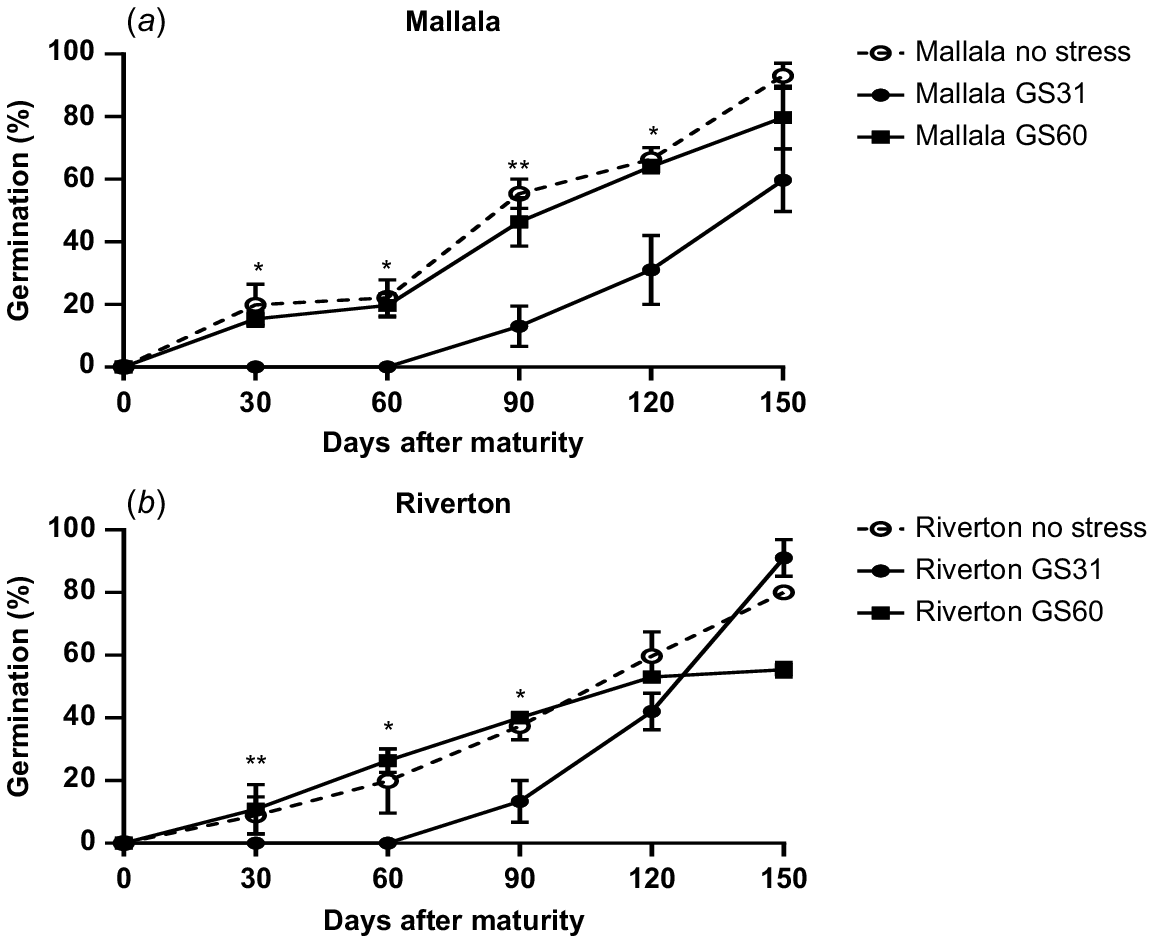
L. rigidum populations also showed significant differences in seed germination pattern in response to water stress treatments (Fig. 2). The seeds from the no stress treatment were less dormant and started germination at 30 DAM (Fig. 2). Water stress imposed at GS60 had little effect on seed dormancy, whereas the seeds of plants exposed to water stress from GS31 were most dormant and took longer to start germination (Fig. 2). For instance, at 90 DAM for GS31 only 8% and 22% seed germination was observed in RAC1 and RAC3, compared to 50% germination in the no stress treatment of both the populations, respectively (P < 0.05; Fig. 2). Significant differences in germination of different water stress treatments during the after-ripening period indicate strong impacts of water stress on seed dormancy of L. rigidum and B. diandrus populations.
Germination pattern of two L. rigidum ((a) RAC1 (b) RAC3) populations under water stress and no stress treatments. The error bars show ± s.e., and the symbols show significant differences at P < 0.05 (*) or P < 0.01 (**). As there was no site × population × stress timing interaction, the results for the two sites were pooled.
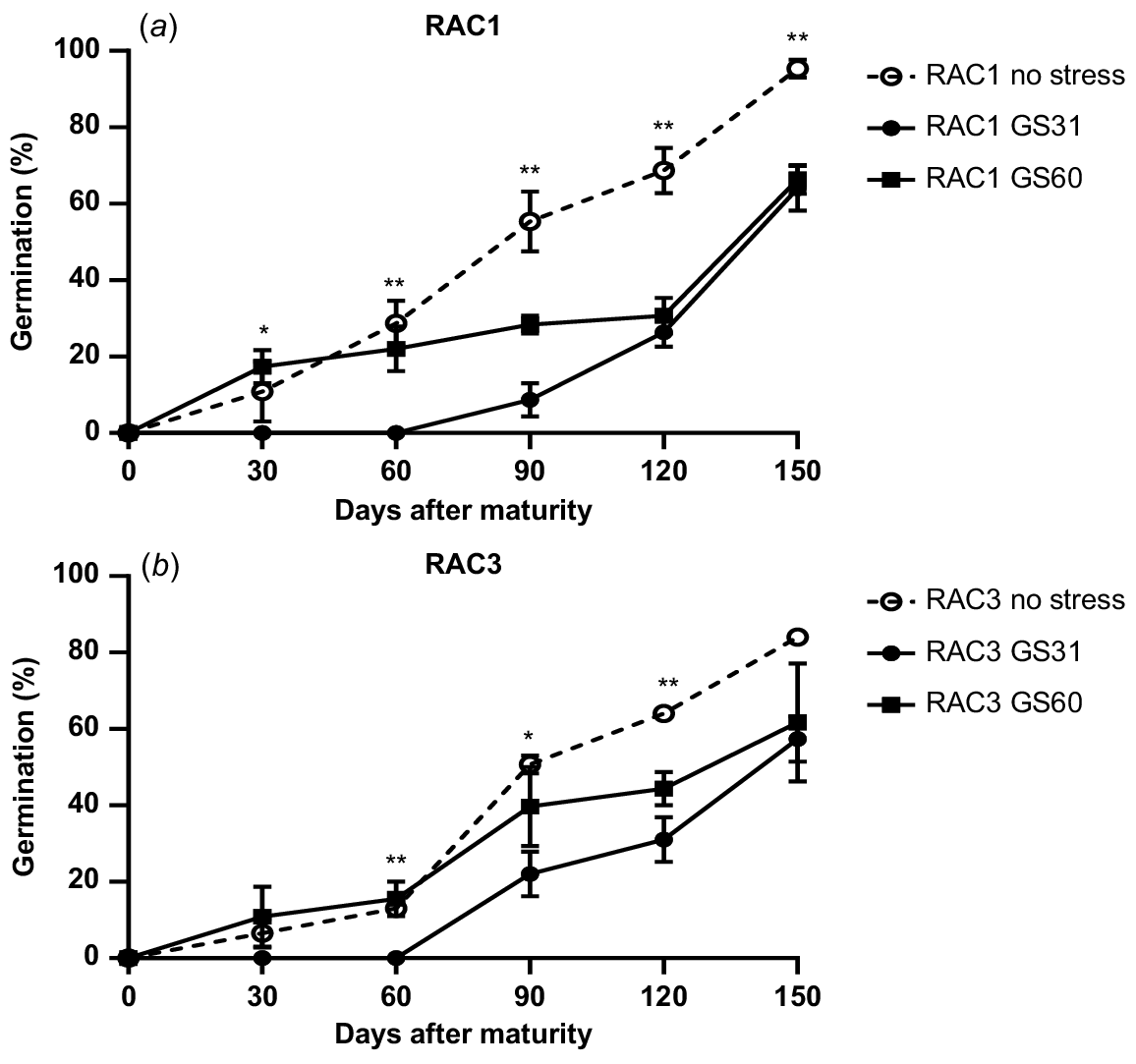
ABA1 gene expression
There were significant differences in the expression levels of ABA1 between the water stress treatments in Mallala (P = 0.01) and Riverton (P < 0.001) populations of B. diandrus. During the after-ripening period, the ABA1 gene expression levels with no stress and water stress at GS60 declined in both B. diandrus populations. The differences in the ABA1 expression levels among the treatments were more noticeable at 90 DAM. Higher ABA1 expression levels were observed for Mallala and Riverton in the no stress treatments (113%, 104%) as compared to GS31 (85%, 74.5%) and GS60 (96%, 0%) (Fig. 3a, b). The expression of ABA1 gene declined at 150 DAM as compared to earlier assessments at 30 and 90 DAM. At 150 DAM no detectable expression was observed in the water stress at GS31 and GS60 treatments of Riverton (P = 0.56), nor the GS31 treatment of Mallala (P = 0.76). The differences in the expression levels of GS31 and GS60 were non-significant.
Relative ABA1 gene expression analysis (αTubulin and ELF were the control genes) of the seeds of stress vs control treatments of two B. diandrus (a, b) and two L. rigidum (c, d) populations at 30, 90 and 150 DAM. The symbols show significant differences at P < 0.05 (*), or P < 0.01 (**) or P < 0.001 (***).
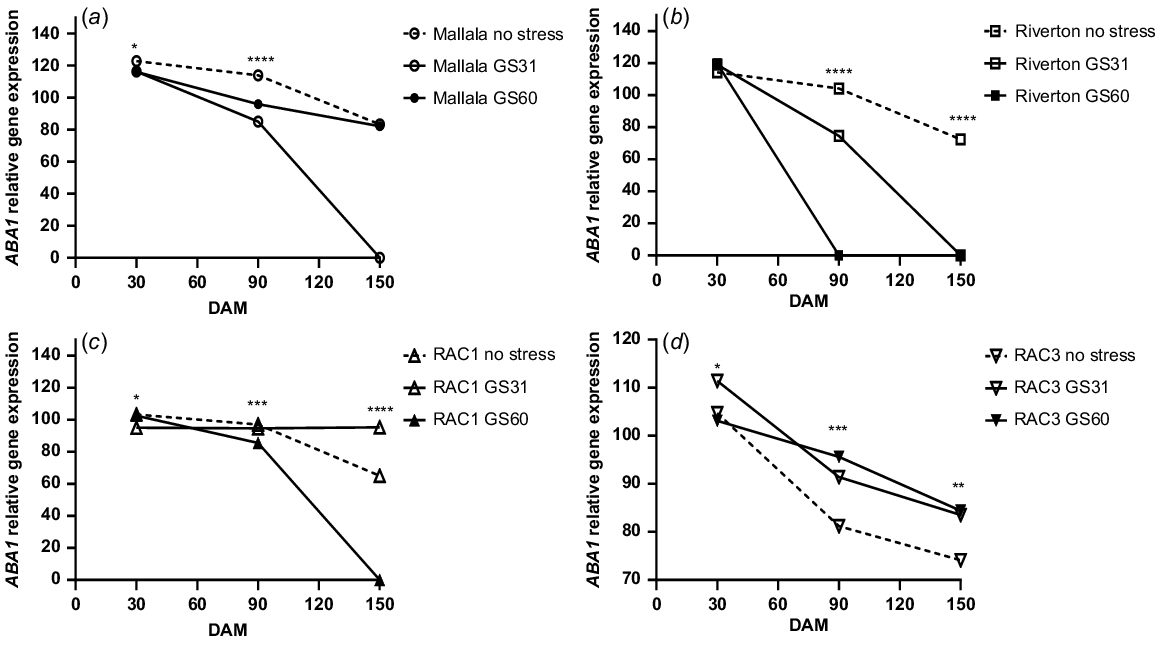
The analysis of ABA1 gene expression in L. rigidum populations showed significant differences between the seeds produced by plants exposed to water stress at the GS31, GS60 and no stress treatments. The no stress treatments of both L. rigidum populations (RAC1 and RAC3) were less dormant and had lower ABA1 expression levels, and the water stress applied at GS31 had a significant effect on ABA1 expression levels (Fig. 3c, d). A significant decline in the ABA1 expression levels were observed in all the treatments from 30 DAM to 150 DAM (Fig. 3c, d).
GA20ox gene expression
In contrast to ABA1, GA20ox gene expression showed a significant increase during the after-ripening period. Water stress treatments of both populations of B. diandrus had a lower GA20ox expression at 30 DAM, but there was an increase over time in all treatments at 150 DAM (Fig. 4a, b). For example, at 30 DAM, GA20ox gene expression for Mallala was 107% for the no stress treatment, whereas at 150 DAM the GA20ox expression had increased to 132% (Fig. 4a). Similar trends showing increase in GA20ox gene expression levels over time were observed in the Riverton population as well, where GA20ox expression increased significantly between 30 and 150 DAM (160%) (Fig. 4b). Consistent with the Mallala population, GA20ox expression levels were higher for the seeds of well-watered plants than those under water stress at the GS31 stage in Riverton B. diandrus, especially at 150 DAM.
Relative GA20ox gene expression (αTubulin and ELF were the control genes) analysis of the seeds of stress vs control treatments of two B. diandrus (a, b) and two L. rigidum (c, d) populations at 30, 90 and 150 DAM. The symbols show significant differences at P < 0.05 (*), or P < 0.01 (**) or P < 0.001 (***).
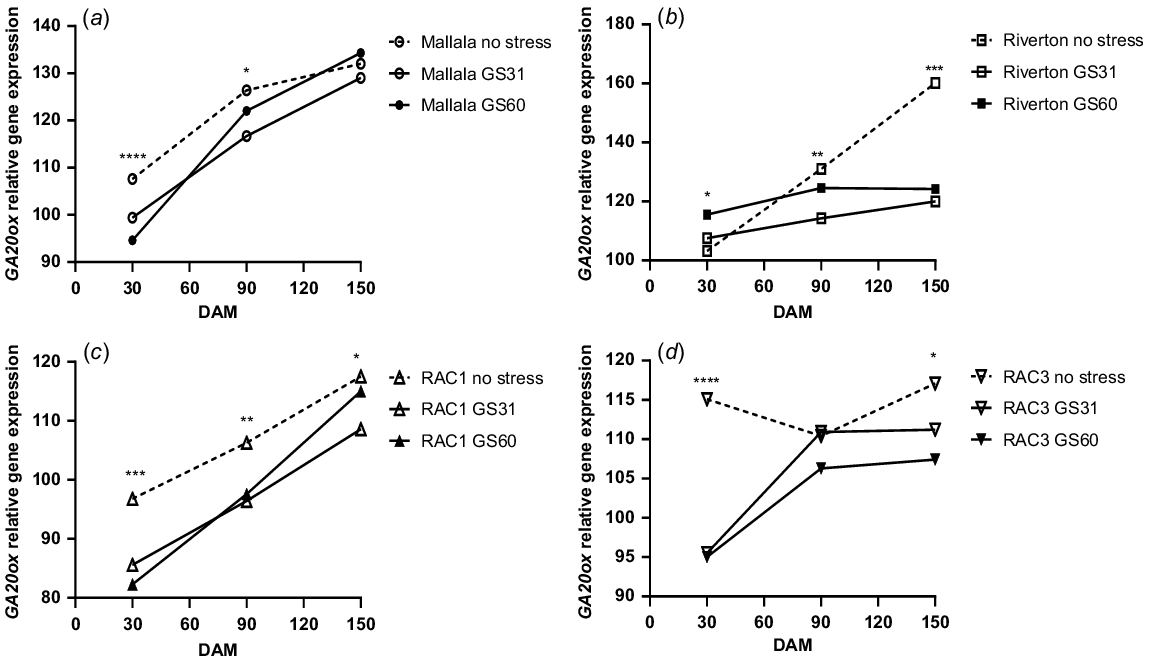
During the after-ripening period in L. rigidum populations, GA20ox gene expression increased and ABA1 gene expression declined. Significantly higher GA20ox gene expression levels (P < 0.001) were observed in the no stress (control) treatment, as compared to the water stress treatment at GS31 (Fig. 4c, d). At 30 DAM, RAC1 and RAC3 had lower GA20ox expression for all the stress treatments, followed by a gradual increase in the gene expression over time (Fig. 4c, d). At 150 DAM a similar trend of increase in GA20ox expression levels during the after-ripening period was observed in all the stress treatments of RAC3 (Fig. 4d). The increase in GA20ox expression levels from 30 DAM to 150 DAM suggests a contribution of this gene to the loss of seed dormancy during the after-ripening period. The correlation analysis between seed germination and GA20ox gene expression showed a significant positive relationship for all water stress treatments in both populations of B. diandrus (P = 0.001) and L. rigidum (P = 0.007; Fig. 5).
Discussion
Studies on climate change have shown that the increasing temperatures in Australia associated with global warming will result in reduced rainfall events and more frequent droughts (Department of the Environment and Energy (DEE), Australian Government 2017). In South Australia, the crop growing areas already experience variable climatic conditions especially in spring where spring rainfall is highly variable. The ecological consequence of this climatic variability in weed seed dormancy are unclear. Weed population dynamics are greatly influenced by seed production and the success of weed management systems is highly dependent on preventing replenishment of the soil seed bank overtime (Norris 2003). However, there have been limited investigations of the effect of moisture stress on weed seed dormancy and the genetic regulation of seed dormancy in response to water stress remains unclear.
In this study, water stress during reproductive development had a significant effect on seed production and seed mass of B. diandrus and L. rigidum populations. Earlier onset of water stress at GS31 had a larger effect on seed production but a smaller effect on seed mass than later water stress from GS60 (panicle emergence) in both weed species compared to the well-watered plants (Tables 1 and 2). Similar findings were reported by Eslami et al. (2010), where the plants of wild radish (Raphanus raphinistrum) exposed to different levels of water stress showed 9–85% reduction in the number of seeds/plant as compared to the non-stressed plants. The ability of L. rigidum and B. diandrus to only show modest reduction in seed production under reduced soil moisture availability (25% FC) shows that both weed species can survive and reproduce under dry climatic conditions often experienced in spring in Australia.
Analysis of seed dormancy through germination assessments showed that seeds produced in no water stress treatments lost seed dormancy more quickly than plants exposed to water stress from the GS31 (Figs 1 and 2). Plants exposed to water stress from GS60 onwards produced seeds with similar seed dormancy as the control in B. diandrus. Even though water stress applied at GS60 had a significant effect on seed mass of B. diandrus populations compared to the no stress control, this late stress did not have a significant effect on seed dormancy. In contrast, water stress applied at GS31 not only reduced seed production and seed mass, it also produced seeds with higher dormancy than no stress and late stress (GS60). However, L. rigidum plants that experienced water stress at GS60 showed a significant reduction in seed mass and intermediate level of seed dormancy relative to the control and water stress at GS31. Importantly, both water stress treatments had greater seed dormancy in L. rigidum than the no stress treatment. According to Sharif-Zadeh and Murdoch (2000), water stress imposed on plants of Cenchrus ciliaris at anthesis and caryopses maturation stages increased dormancy in C. ciliaris seeds. In their study, seeds exposed to water stress treatments had 14–19% germination as compared to 67% for the control (no water stress) treatment. From these results they concluded that greater dormancy in seed produced by water stressed plants in C. ciliaris might be associated with reduced water permeability of glumes in response to water stress. According to Sharif-Zadeh and Murdoch (2000), reduced water permeability in seeds of water stressed plants may not only protect seeds from drought stress but also impose dormancy, which is itself an important survival mechanism.
In our study, earlier onset of water stress after the first node stage, applied to B. diandrus and L. rigidum plants, resulted in the production of fewer but more dormant seeds. In contrast, seeds produced by plants exposed to water stress later, from flowering onward, had lower levels of dormancy and germinated earlier than seeds from plants exposed to stress after the first node stage. These results differ from the findings of Steadman et al. (2004b), where L. rigidum seeds produced under water stress in atypical cool conditions for spring had lower dormancy as compared to the seeds grown under warm and well-watered conditions. In our study, pots were maintained in the greenhouse under typical warm spring temperature conditions during reproductive development, similar to what would be experienced in the field. These differences in ambient temperature conditions during water stress may explain the disparity in response to water stress between our study and Steadman et al. (2004b).
Some of the differences in the influence of water stress on weed seed dormancy between our research and published literature may be related to the methodology used to impose water stress. In our study, water stress was imposed by cessation of watering at a pre-determined growth stage and pots were rewatered up to 25% of the field capacity. It is highly likely that plants of B. diandrus and L. rigidum would have experienced severe water stress after several days of the end of irrigation. However, in a study on Avena fatua by Peters (1982), plants were exposed to water stress after panicle emergence, and the pots were only watered when the flag leaf started showing signs of wilting. Similarly, a study on Sorghum halepense subjected plants to cycles of drought by withholding water for 5 days and then rewatering the plants again (Benech Arnold et al. 1992). In contrast, water stress to Bromus tectorum plants was imposed by changing polyethylene glycol (PEG) solutions, where the onset of water stress would have been instantaneous (Thill et al. 1979). Given the disparity in the methodology used to expose weed plants to water stress, it is understandable to see the differences in its effect on seed dormancy.
Most of the previous studies on the impact of water stress on weed seed dormancy have not investigated mechanisms associated with seed dormancy. In our studies, seeds of water stressed plants of B. diandrus and L. rigidum were not only assessed for loss of seed dormancy during the after-ripening period but also for changes in the expression of two important genes known to regulate seed dormancy. ABA is a plant hormone well known to be linked with dormancy induction (Bentsink et al. 2018). Kanno et al. (2010) verified that ABA was synthesised in both maternal and zygotic tissues during seed development, and maternal ABA can be translocated to the embryos and induce primary seed dormancy. Gibberellin (GA) is the other major plant hormone involved in the regulation of seed dormancy and germination (Finch-Savage and Leubner-Metzger 2006). The level of biologically active GA in plant tissues is determined by the balance between its biosynthesis and inactivation (Yamaguchi 2008). The biosynthesis of GA is regulated mainly by reactions catalysed by GA 20-oxidase (GA20ox) and GA 3-oxidase (GA3ox), while its inactivation is controlled primarily by GA 2-oxidase (GA2ox). In general ABA and GA play antagonistic roles in regulating seed dormancy (Wang et al. 2016).
In our studies the qPCR analysis of seeds of water stress treatments showed higher ABA1 levels soon after seed maturity (30 DAM) followed by a general decline as seed became non-dormant. The clearest effect of water stress on ABA1 was observed in RAC3 population of L. rigidum, which showed significantly lower ABA1 expression in no stress treatment than the two water stress treatments (Fig. 3d). In B. diandrus populations and RAC1 L. rigidum, there was no clear difference in ABA1 expression between water stress treatments. Over the after-ripening period, there was a decline in ABA1 expression and a sharp increase in GA20ox gene expression levels in all the treatments (Figs 3 and 4). The large observed increase in GA20ox gene expression levels during the after-ripening period suggests variation in seed dormancy between the water stress treatments was strongly linked with GA20ox expression. This was reflected in a significant positive correlation between GA20ox expression and seed germination in B. diandrus and L. rigidum (Fig. 5). Even though there was a negative association between ABA1 expression and loss of seed dormancy, the relationship was not as strong as for GA20ox. The results of this study showed that GA20ox expression plays a significant role in the regulation of seed dormancy in these two weed species. When seeds of these weed species developed without water stress, they showed higher expression levels of GA20ox than those that experienced water stress during reproductive development.
Conclusion
Soil moisture conditions in which plants of B. diandrus and L. rigidum undergo reproductive development and mature had a strong influence on the levels of seed dormancy in these two important weed species. Water stress treatments also had a significant effect on the expression of ABA1 and GA20ox genes, which are known to be involved in the regulation of seed dormancy. The results of this study showed greater differences in GA20ox between water stress treatments than ABA1.
As expected, water stressed plants produced fewer seeds than well-watered plants but had elevated levels of seed dormancy. As spring rainfall in Australian agricultural regions can be highly erratic, weeds are likely to not only produce different number of seed between seasons, but it is also likely that the seeds produced will differ in their dormancy status. Since the seeds of B. diandrus and L. rigidum collected from plants that experienced water stress at GS31 stage were the most dormant, it is likely dry conditions during the reproductive stage will result in replenishment of the seedbank with weed seeds of greater dormancy status. Therefore, growing season rainfall received during reproductive development of weeds in the previous season is likely to influence the timing of their plant establishment and effectiveness of pre-sowing weed control tactics in the next year.
Data availability
The data that support this study are available in the article in the form of figures and tables.
Conflicts of interest
The authors declare that they have no conflicts of interest. Christopher Preston is an Associate Editor of Animal Production Science but was blinded from the peer-review process for this paper.
Declaration of funding
Zarka Ramiz was supported by a PhD scholarship from the University of Adelaide Australia (Australian Government Research Training Program (RTP) Scholarship).
Acknowledgements
The authors acknowledge Benjamin Fleet, Malinee Thongmee and Geetha Velappan for technical support.
References
Adu-Yeboah P, Malone JM, Fleet B, Gill G, Preston C (2020) EPSPS gene amplification confers resistance to glyphosate resistant populations of Hordeum glaucum Stued (northern barley grass) in South Australia. Pest Management Science 76, 1214-1221.
| Crossref | Google Scholar | PubMed |
Arora R, Rowland LJ, Tanino K (2003) Induction and release of bud dormancy in woody perennials: a science comes of age. HortScience 38, 911-921.
| Crossref | Google Scholar |
Barton LV (1962) The germination of weed seeds. Weeds 10, 174-182.
| Crossref | Google Scholar |
Benech Arnold RL, Fenner M, Edwards PJ (1992) Changes in dormancy level in Sorghum halepense seeds induced by water stress during seed development. Functional Ecology 6, 596-605.
| Crossref | Google Scholar |
Boutsalis P, Gill GS, Preston C (2012) Incidence of herbicide resistance in rigid ryegrass (Lolium rigidum) across southeastern Australia. Weed Technology 26, 391-398.
| Crossref | Google Scholar |
Bureau of Meteorology (2022) Climate statistics for Australian locations. Available at http://www.bom.gov.au/climate/averages/tables/cw_023031.shtml [Accessed 23 December 2022]
Ceccato D, Bertero D, Batlla D, Galati B (2015) Structural aspects of dormancy in quinoa (Chenopodium quinoa): importance and possible action mechanisms of the seed coat. Seed Science Research 25, 267-275.
| Crossref | Google Scholar |
Cirujeda A, Taberner A (2009) Cultural control of herbicide-resistant Lolium rigidum Gaud. populations in winter cereal in Northeastern Spain. Spanish Journal of Agricultural Research 7(1), 146-154.
| Crossref | Google Scholar |
Department of the Environment and Energy (DEE), Australian Government (2017) Available at https://www.environment.gov.au/climate-change/climatescience/impacts/qld [Accessed 1 May 2022]
Eslami SV, Gill GS, McDonald G (2010) Effect of water stress during seed development on morphometric characteristics and dormancy of wild radish (Raphanus raphanistrum L.) seeds. International Journal of Plant Production 4, 159-168.
| Google Scholar |
Fenner M (1991) The effects of the parent environment on seed germinability. Seed Science Research 1, 75-84.
| Crossref | Google Scholar |
Finch-Savage WE, Leubner-Metzger G (2006) Seed dormancy and the control of germination. New Phytologist 171, 501-523.
| Crossref | Google Scholar |
Footitt S, Douterelo-Soler I, Clay H, Finch-Savage WE (2011) Dormancy cycling in Arabidopsis seeds is controlled by seasonally distinct hormone-signaling pathways. Proceedings of the National Academy of Sciences 108, 20236-20241.
| Crossref | Google Scholar |
Gaines TA, Zhang W, Wang D, Bukun B, Chisholm ST, Shaner DL, Nissen SJ, Patzoldt WL, Tranel PJ, Culpepper AS, Grey TL, Webster TM, Vencill WK, Sammons RD, Jiang J, Preston C, Leach JE, Westra P (2010) Gene amplification confers glyphosate resistance in Amaranthus palmeri. Proceedings of the National Academy of Sciences 107, 1029-1034.
| Google Scholar |
Ghersa CM, Holt JS (1995) Using phenology prediction in weed management: a review. Weed Research 35, 461-470.
| Crossref | Google Scholar |
Ghersa CM, Martinez-Ghersa MA, Benech-Arnold RL (1997) Seed dormancy implications in grain and forage production. Journal of Production Agriculture 10, 111-117.
| Crossref | Google Scholar |
Gill GS (1996) Why annual ryegrass is a problem in Australian agriculture. Plant Protection Quarterly 11, 193-195.
| Google Scholar |
Gill GS, Blacklow WM (1985) Variations in seed dormancy and rates of development of great brome, Bromus diandrus Roth., as adaptations to the climates of southern Australia and implications for weed control. Australian Journal of Agricultural Research 36, 295-304.
| Crossref | Google Scholar |
Gill GS, Poole ML, Holmes JE (1987) Competition between wheat and brome grass in Western Australia. Australian Journal of Experimental Agriculture 27, 291-294.
| Crossref | Google Scholar |
Hilhorst HWM, Toorop PE (1997) Review on dormancy, germinability and germination in crop and weed seeds. Advances in Agronomy 61, 112-165.
| Google Scholar |
Jaleel CA, Manivannan P, Wahid A, Farooq M, Al-Juburi HJ, Somasundaram R, Panneerselvam R (2009) Drought stress in plants: a review on morphological characteristics and pigments composition. International Journal of Agriculture and Biology 11, 100-105.
| Google Scholar |
Kanno Y, Jikumaru Y, Hanada A, Nambara E, Abrams SR, Kamiya Y, Seo M (2010) Comprehensive hormone profiling in developing Arabidopsis seeds: examination of the site of ABA biosynthesis, ABA transport and hormone interactions. Plant and Cell Physiology 51(12), 1988-2001.
| Crossref | Google Scholar |
Kendall SL, Hellwege A, Marriot P, Whalley C, Graham IA, Penfield S (2011) Induction of dormancy in Arabidopsis summer annuals requires parallel regulation of DOG1 and hormone metabolism by low temperature and CBF transcription factors. The Plant Cell 23, 2568-2580.
| Crossref | Google Scholar | PubMed |
Kleemann SGL, Gill GS (2006) Differences in the distribution and seed germination behaviour of populations of Bromus rigidus and Bromus diandrus in South Australia: adaptations to habitat and implications for weed management. Australian Journal of Agricultural Research 57, 213-219.
| Crossref | Google Scholar |
Kloot PM (1983) The genus Lolium in Australia. Australian Journal of Botany 31, 421-435.
| Crossref | Google Scholar |
Kon KF, Blacklow WM (1988) Identification, distribution and population variability of great brome (Bromus diandrus Roth) and rigid brome (Bromus rigidus Roth). Australian Journal of Agricultural Research 39, 1039-1050.
| Crossref | Google Scholar |
Livak KJ, Schmittgen TD (2001) Analysis of relative gene expression data using real-time quantitative PCR and the 2−ΔΔCT method. Methods 25, 402-408.
| Crossref | Google Scholar | PubMed |
MacGregor DR, Kendall SL, Florance H, Fedi F, Moore K, Paszkiewicz K, Smirnoff N, Penfield S (2015) Seed production temperature regulation of primary dormancy occurs through control of seed coat phenylpropanoid metabolism. New Phytologist 205, 642-652.
| Crossref | Google Scholar | PubMed |
Malone JM, Morran S, Shirley N, Boutsalis P, Preston C (2016) EPSPS gene amplification in glyphosate-resistant Bromus diandrus. Pest Management Science 72, 81-88.
| Crossref | Google Scholar | PubMed |
Norris R (2003) Echinochloa crus-galli (barnyardgrass) seed rain under irrigated conditions. In ‘Seedbanks: determination, dynamics and management. Aspects of applied biology. Vol. 69’. (Eds RM Bekker, F Forcella, AC Grundy, NE Jones, EJP Marshall, AJ Murdoch) pp. 163–170. (Association of Applied Biologists, European Weed Research Society)
Owen MJ, Walsh MJ, Llewellyn RS, Powles SB (2007) Widespread occurrence of multiple herbicide resistance in Western Australian annual ryegrass (Lolium rigidum) populations. Australian Journal of Agricultural Research 58(7), 711-718.
| Crossref | Google Scholar |
Patterson DT (1995) Effects of environmental stress on weed/crop interactions. Weed Science 43, 483-490.
| Crossref | Google Scholar |
Peters NCB (1982) The dormancy of wild oat seed (Avena fatua L.) from plants grown under various temperature and soil moisture conditions. Weed Research 22, 205-212.
| Crossref | Google Scholar |
Pourrat Y, Jacques R (1975) The influence of photoperiodic conditions received by the mother plant on morphological and physiological characteristics of Chenopodium polyspermum L. seeds. Plant Science Letters 4, 273-279.
| Crossref | Google Scholar |
Ramesh K, Matloob A, Aslam F, Florentine SK, Chauhan BS (2017) Weeds in a changing climate: vulnerabilities, consequences, and implications for future weed management. Frontiers in Plant Science 8, 95.
| Crossref | Google Scholar | PubMed |
Ramiz Z, Malone J, Preston C, Gill G (2022a) Selection for seed dormancy within Bromus diandrus populations alters ABA1 and GA20ox gene expression. Crop & Pasture Science 73, 1416-1424.
| Crossref | Google Scholar |
Ramiz Z, Malone J, Preston C, Gill G (2022b) Genetic control of seed dormancy in Lolium rigidum and its association with GA20ox and ABA1 expression. Crop & Pasture Science 73, 1406-1415.
| Crossref | Google Scholar |
Royo-Esnal A, Recasens J, Garrido J, Torra J (2018) Rigput Brome (Bromus diandrus Roth.) management in a no-till field in Spain. Agronomy 8(11), 251.
| Crossref | Google Scholar |
Ruttink T, Arend M, Morreel K, Storme V, Rombauts S, Fromm J, Bhalerao RP, Boerjan W, Rohde A (2007) A molecular timetable for apical bud formation and dormancy induction in poplar. The Plant Cell 19, 2370-2390.
| Crossref | Google Scholar | PubMed |
Sharif-Zadeh F, Murdoch AJ (2000) The effects of different maturation conditions on seed dormancy and germination of Cenchrus ciliaris. Seed Science Research 10, 447-457.
| Crossref | Google Scholar |
Steadman KJ (2004) Dormancy release during hydrated storage in Lolium rigidum seeds is dependent on temperature, light quality, and hydration status. Journal of Experimental Botany 55, 929-937.
| Crossref | Google Scholar | PubMed |
Steadman KJ, Crawford AD, Gallagher RS (2003) Dormancy release in Lolium rigidum seeds is a function of thermal after-ripening time and seed water content. Functional Plant Biology 30, 345-352.
| Crossref | Google Scholar | PubMed |
Steadman KJ, Bignell GP, Michael PJ (2004a) Stimulating dormancy release and emergence of annual ryegrass (Lolium rigidum) seeds using short-term hydrated storage in darkness. Australian Journal of Agricultural Research 55, 787-795.
| Crossref | Google Scholar |
Steadman KJ, Ellery AJ, Chapman R, Moore A, Turner NC (2004b) Maturation temperature and rainfall influence seed dormancy characteristics of annual ryegrass (Lolium rigidum). Australian Journal of Agricultural Research 55, 1047-1057.
| Crossref | Google Scholar |
Thill DC, Schirman RD, Appleby AP (1979) Influence of soil moisture, temperature, and compaction on the germination and emergence of downy brome (Bromus tectorum). Weed Science 27, 625-630.
| Crossref | Google Scholar |
Tuan PA, Kumar R, Rehal PK, Toora PK, Ayele BT (2018) Molecular mechanisms underlying abscisic acid/gibberellin balance in the control of seed dormancy and germination in cereals. Frontiers in Plant Science 9, 668.
| Crossref | Google Scholar | PubMed |
Wang D, Gao Z, Du P, Xiao W, Tan Q, Chen X, Li L, Gao D (2016) Expression of ABA metabolism-related genes suggests similarities and differences between seed dormancy and bud dormancy of peach (Prunus persica). Frontiers in Plant Science 6, 1248.
| Crossref | Google Scholar | PubMed |
Yamaguchi S (2008) Gibberellin metabolism and its regulation. Annual Review of Plant Biology 59, 225-251.
| Crossref | Google Scholar |