The roles of non-structural carbohydrates in fruiting: a review focusing on mango (Mangifera indica)
Gerhard C. Rossouw
A
Abstract
Reproductive development of fruiting trees, including mango (Mangifera indica L.), is limited by non-structural carbohydrates. Competition for sugars increases with cropping, and consequently, vegetative growth and replenishment of starch reserves may reduce with high yields, resulting in interannual production variability. While the effect of crop load on photosynthesis and the distribution of starch within the mango tree has been studied, the contribution of starch and sugars to different phases of reproductive development requires attention. This review focuses on mango and examines the roles of non-structural carbohydrates in fruiting trees to clarify the repercussions of crop load on reproductive development. Starch buffers the plant’s carbon availability to regulate supply with demand, while sugars provide a direct resource for carbon translocation. Sugar signalling and interactions with phytohormones play a crucial role in flowering, fruit set, growth, ripening and retention, as well as regulating starch, sugar and secondary metabolites in fruit. The balance between the leaf and fruit biomass affects the availability and contributions of starch and sugars to fruiting. Crop load impacts photosynthesis and interactions between sources and sinks. As a result, the onset and rate of reproductive processes are affected, with repercussions for fruit size, composition, and the inter-annual bearing pattern.
Keywords: carbohydrate metabolism, carbon allocation, crop physiology, flowering, fruit development, hormonal regulation, starch, sugar sensing, yield.
Introduction
Plants use atmospheric carbon for biosynthesis of structural carbohydrates (SC) and non-structural carbohydrates (NSC). SC, such as cellulose and hemicellulose, are used for constructing and strengthening plant tissues, including stems and cells (Hartmann and Trumbore 2016). Shorter-chain NSC (i.e. starch and sugars) contribute to carbon translocation, energy production, metabolic processes, osmotic regulation and control of vegetative and reproductive development (Loescher et al. 1990; Smeekens et al. 2010; Dominguez and Niittylä 2022). Temporal variability in NSC concentrations is well-studied in deciduous trees and vines grown in temperate climates, notably fruit and nut crops (Boldingh et al. 2000; Holzapfel et al. 2010; Rossouw et al. 2017b; Mesa et al. 2019; Breen et al. 2020; Tixier et al. 2020). To a lesser extent, NSC dynamics have been explored for evergreen fruit trees in tropical or sub-tropical environments (Davie and Stassen 1997b; Olesen et al. 2008; Singh et al. 2017).
In mango (Mangifera indica L.), our understanding of NSC utilisation is incomplete. However, variability in starch across organs at key phenological stages has been described (Davie and Stassen 1997a; Stassen and Janse van Vuuren 1997; Davie et al. 1999; Normand et al. 2009), as has the abundance of major sugars (Normand et al. 2006; Prasad et al. 2014). Understanding NSC in mango trees is important for enhancing fruit productivity.
Starch, the bulk of NSC, is stored as reserves to be degraded and mobilised to increase the pool of usable sugars (Fig. 1). Leaf storage of starch is typically transient whereas storage in perennial woody structures such as roots and branches are more long term (Normand et al. 2009). Starch regulates carbon utilisation indirectly whereas sugars influence organ growth directly (Stitt and Zeeman 2012). Research in mango has focused on starch, partly due to the relative ease of quantification (Smith and Zeeman 2006). Methods quantifying individual sugars are usually more sophisticated (Ma et al. 2014; Georgelis et al. 2018).
Conceptual distribution of photosynthates and remobilisation of root starch reserves during fruit development. The bottom right wagon wheels show root transverse sections with dark brown starch deposits in xylem parenchyma cells. Left: in the absence of fruit, sugars are translocated from leaves to roots, forming elevated root starch reserves. Centre: with a balanced canopy, leaves provide sugars for fruit growth, and root starch reserves support peak sink demands. Optimal allocation of resources ensures both fruit development and leaf function. Leaf chlorophyll content remains stable as fruits mature. Right: overcropped trees rely more on root starch for fruit growth, depleting reserves. Increased competition for resources may lead to smaller fruit and reduced leaf chlorophyll, resulting in pale green leaves.
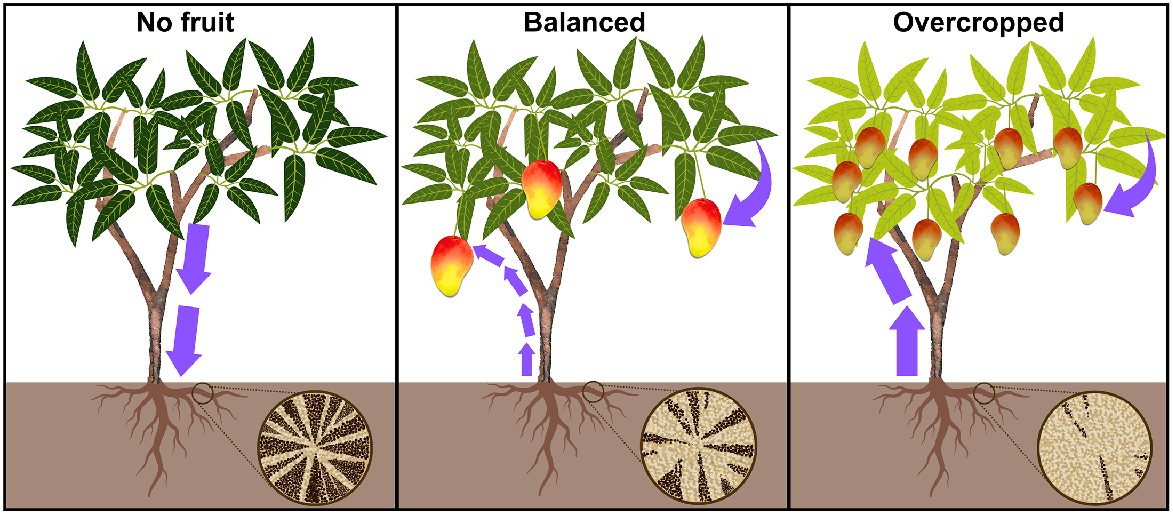
Sugar concentrations are typically less than starch (Smith and Stitt 2007; Smeekens et al. 2010). Sugars supply structural units for organic compounds, including proteins and amino acids, and secondary metabolites such as colour and flavour compounds (Priestly 1962). Sucrose, glucose and fructose concentrations differ between cultivars, tissues and developmental stages (Li et al. 2020). Sucrose, as the predominant transported carbon form from sources to sinks, is integral for resource allocation (Chauhan and Pandey 1984). Sucrose is the principal sugar found in mango fruit, whilst fructose, followed by glucose, are usually the most abundant reducing sugars (Medlicott and Thompson 1985; Malundo et al. 2001).
Source–sink organ relations dictate sugar flow. In mango, fruit is the predominant sink, importing sugars irrespective of the number of supporting leaves (Chacko et al. 1982). Source organs, like photosynthesising leaves or storage tissues, should be considered in conjunction with fruit biomass when assessing carbohydrate source and sink organ relations (Roper and Loescher 1987; Kliewer and Dokoozlian 2005; Wünsche and Ferguson 2010). Crop load, the number and size of the fruit produced per tree or branch unit (Wünsche and Ferguson 2010), influence NSC use by sinks. Excessive yield depletes NSC reserves beyond the tree’s recharge capacity, diminishing reproductive development the following season (Capelli et al. 2016) (Fig. 1). An excessive crop load delays fruit maturation, and lead to smaller and inferior quality fruit (Simmons et al. 1998). Low yield promotes NSC allocation towards reserve storage and may stimulate vegetative growth (Erf and Proctor 1987; Blanco et al. 1995; Berman and DeJong 2003).
Starch concentrations in mango roots, wood, bark, shoots and leaves tend to decrease in winter, during flowering, fruit set and the spring vegetative flush (Stassen and Janse van Vuuren 1997; Davie et al. 1999). Root and wood starch negatively correlates with fruit growth (Davie and Stassen 1997a), with greater crop loads reducing starch reserves as fruit draws carbohydrates from alternative sinks (Davie and Stassen 1997b) (Fig. 1).
This review examines NSC dynamics in fruit trees, emphasising the roles of NSC in fruit development and productivity. It explores NSC types and their roles in reproductive development, the importance of leaf photosynthesis for fruiting including crop load effects, and NSC allocation between sources and sinks. Proposing future research areas, this review uses mango as a case study, incorporating resources from fundamental plant studies and other fruiting species for comprehensiveness.
Types of NSC and their roles in fruiting
NSC exist in plants in two primary forms: (1) starch; and (2) sugars (Table 1). Starch molecules are not osmotically active, meaning they cannot be translocated and are used for carbon storage (Lalonde et al. 1999). Starch is converted to sugars to allow mass flow along the sieve tubes of the phloem. At the sink, sugars and water are unloaded into expanding or storage cells where they are metabolised for growth, storage, or as signals for biochemical processes. Partitioning of carbon between starch and sugars is regulated by carbon supply and demand signalling (Smith and Stitt 2007; Eveland and Jackson 2012; Stitt and Zeeman 2012).
Starch | Sucrose | Hexoses (glucose and fructose) | Low abundance sugars and polyols | ||
---|---|---|---|---|---|
Phloem mobility | ImmobileA,B | Mobile (primary transport sugar)A,B | MobileA,B | MobileA,B | |
Structural classification | Polysaccharide, composed of glucose monomersB,C | Disaccharide, composed of a glucose and fructose monomerB,C | MonosaccharidesB,C | Monosaccharides (e.g. galactose), disaccharides (e.g. trehalose), oligosaccharides (e.g. raffinose), polyols (e.g. myo-inositol), phosphorylated intermediates (e.g. trehalose-6-phosphate)B,C | |
Leaf origin and utilisation | Synthesised and stored at day, degraded and exported at nightB,D | End-product of photosynthesis or synthesised from starchB,D. Metabolised, converted to starch or exportedB,D | End-products of photosynthesis or synthesised from starchB. Metabolised or exportedB | Products of photosynthesis (e.g. maltose) or synthesised (e.g. myo-inositol)D,E,F,G. Metabolised or exportedE,F | |
Fruit origin and utilisation | Synthesised during fruit growth, degraded during late maturation and ripeningH,I | Imported or synthesised from starchB,J,K | Imported or synthesised during ripeningB,K | Imported or synthesised and metabolisedB,L,M | |
Root and wood origin and utilisation | Synthesised when leaf assimilate supply outweighs sink demand, remobilised when carbon demand outweighs leaf supplyN,O,P | Imported or synthesised from starch and metabolisedB,O,P,Q | Imported or synthesised from starch and metabolisedB,O,Q,R | Imported or synthesised and metabolisedM,O,Q | |
Key roles in reproductive development | Degradation and remobilisation regulate carbon availability for floral induction, flowering, fruit set, retention and growth.S,T,U | Carbon transport to buds, flowers and fruit for growth, energy generation and signallingB,S,T | Supply to buds, flowers and fruit, used for growth, energy generation and signallingB,E,S,T | Provides signalling to regulate reproductive processes including flowering, fruit growth and ripeningE,F,G,T | |
Other roles | Reserve storage and remobilisation for respiration and vegetative growthV,W | Long distance carbon transport.A,B Signalling for gene expression, cellular growth, vegetative developmental processes, carbon metabolism and abiotic stress responses.E,X,Y,Z Cellular osmotic regulation.A,G | Resources for cellular respiration.B,C Utilised for synthesis of structural carbohydrates and carbon translocation.B,X,Y Facilitate cellular and osmotic regulation.B,Y | Carbon translocation, cellular osmotic protection and regulation, stabilisation of enzymes and cell membranes.E,X,Y Signalling for gene expression, abiotic stress mitigation, carbon metabolism and vegetative development.E,X,Y |
Starch
Starch is a storage polyglucan that accumulates as granules of amylose or amylopectin in the plastids of cells. Starch acts as a resource for reserve mobilisation to sinks when demand is greater than production (Davie et al. 1999; Normand et al. 2009). In mango, starch is mainly found in xylem parenchyma of roots, the trunk, branches and shoots, and accounts for more than 80% of the NSC (Davie et al. 1999; Normand et al. 2006; Normand et al. 2009).
Starch synthesis and degradation regulate distribution of NSC between sources and sinks. Synthesis is conserved amongst plant species suggesting a common evolutionary origin. For an overview of the biochemical process of starch formation in plant cells, see the review by Pfister and Zeeman (2016). In contrast, the degradation of starch varies across species and between organs (Smith et al. 2005). In leaves, the primary NSC source, starch synthesis and degradation are regulated by enzymes, diurnally by circadian fluctuations (Kötting et al. 2010). Starch in the leaf accumulates in the chloroplasts during the day and is converted to sucrose at night to allow continued export to sinks, and to provide carbon skeletons and energy within the leaf cells (Smith et al. 2005; Stitt and Zeeman 2012). In perennial storage organs, such as roots and wood, starch synthesis and degradation are dependent on developmental processes and source-sink relations (Kötting et al. 2010; MacNeill et al. 2017; Rossouw et al. 2017a) (Fig. 1). In leaves and the perennial structures, starch is catalysed by amylases and debranching enzymes (Smith et al. 2005; Stitt and Zeeman 2012).
Soluble sugars
Sugars are involved in carbon translocation and act as structural components, cell nutrients and as signals for growth and development (Eveland and Jackson 2012). In mango and most other higher plants, sucrose is the main long-distance transport sugar. In developing fruit, sucrose is degraded to the hexoses, glucose and fructose, or their derivatives (Ruan 2012; Durán-Soria et al. 2020; Li et al. 2020). Numerous minor sugars, and polyols (sugar alcohols), including raffinose, trehalose, myo-inositol, mannitol, xylose and maltose also contribute to developmental or other physiological processes such as abiotic stress mitigation in plants (Pandey 1974; Iturriaga et al. 2009; Valluru and Van den Ende 2011).
The availability of sugars controls growth, both as immediate substrates for metabolism and as signalling molecules (Smeekens et al. 2010). Sugar signals allow plants to modulate site-specific and whole-plant growth, thereby coordinating development (Smeekens et al. 2010; Eveland and Jackson 2012). Sucrose signalling occurs directly through binding to sugar sensors or indirectly as hexose signals, a product of sucrose cleavage (Rolland et al. 2006; Eveland and Jackson 2012). Hexoses exhibit a greater signalling potential for promoting organ growth and cell proliferation, whereas sucrose signalling is associated with cell differentiation and maturation (Eveland and Jackson 2012). Minor sugars and polyols, notably myo-inositol, trehalose and raffinose also play roles in sugar signalling (Iturriaga et al. 2009; Valluru and Van den Ende 2011).
As a resource for energy and carbon, sugars are essential for all developmental processes in plants (Rolland et al. 2006; Eveland and Jackson 2012; Sami et al. 2016; Ciereszko 2018; Aluko et al. 2021). As such, vegetative growth, flowering, fruit set, fruit growth and retention, and plant adaption to challenging environmental conditions, are dependent on the plant’s sugar status. Sugar accumulation contributes to the maintenance of cell turgor potential, generating a gradient for water uptake into the cells and cell expansion (Boyer 1988; Rhodes and Samaras 1994).
In vegetative tissues, sugars trigger the proliferation of vegetative organs and produce larger and thicker leaves (Gibson 2005). Additionally, sugars are involved in leaf senescence, (Rolland et al. 2006; Kim 2019). The initiation of leaf senescence is marked by appropriate sugar signals, followed by tightly regulated temporal changes in sugars to supply carbon-mediated energy needed for the senescence processes (Kim 2019). Leaf senescence compensates for energy starvation, the breakdown of organelles such as chloroplast, and loss of photosynthetic activity. As primary cellular energy metabolites, sugar signalling and metabolism govern the induction and progression of leaf senescence.
The availability of NSC regulates canopy development and the balance between leaf and fruit biomass. Leaf-to-fruit ratio, in turn, determines mango fruit growth, regulating NSC concentrations in the tree, completing the feedback loop (Lechaudel et al. 2002; Léchaudel and Joas 2007).
As osmotically active solutes, sugars including sucrose, glucose and fructose, play important roles during abiotic stress conditions including drought, salinity and cold stress (Sami et al. 2016). During stress, these sugars stimulate the osmotic movement of water into cells, raising osmotic pressure. Certain sugars are also osmo-protective, including the raffinose family of oligosaccharides, mainly raffinose, stachyose and verbascose, which accumulates in leaves when plants are exposed to abiotic stresses (Sami et al. 2016). They stabilise proteins and interact with cell membranes to prevent denaturation of proteins under drought and other environmental constraints (Rontein et al. 2002). Fructans are indirectly involved in the osmotic adjustment of plant cells, while trehalose functions as an osmolyte (Sami et al. 2016). Both these sugar types also stabilise membranes, and their accumulation consequently provides osmo-protection for cells.
Sucrose metabolism in fruit and other sinks is self-regulating via starch biosynthesis and sucrose degradation metabolic pathways (Ruan et al. 2012; Durán-Soria et al. 2020). High sucrose concentrations in phloem, resulting from decreased sink demand or an oversupply from the leaves, reduce subsequent sugar loading to the phloem, and NSC accumulate in the surrounding leaf mesophyll cells (Chiou and Bush 1998). Carbohydrate accumulation in the mesophyll, in turn, downregulates photosynthesis. Strong sinks drain sucrose from the phloem creating osmotic pull through phloem loading and decrease sucrose in mesophyll cells to stimulate photosynthesis.
Sucrose cleavage is crucial to allocate carbon between sources and sinks, as well as in the initiation of hexose-based sugar signals (Koch 2004). The glucose and fructose signals modulate the expression of various genes, including those involved in the biosynthesis and perception of hormones such as abscisic acid (ABA). Invertases, enzymes responsible for sucrose cleavage, trigger the initiation and expansion of sinks by modifying the nature of sugar signals from sucrose to hexoses (Sturm and Tang 1999; Koch 2004). During the expansion of organs, changes in the hexose-to-sucrose ratio impact the transition of sinks to storage and maturation. Accordingly, the hexose-to-sucrose ratio regulates the growth and ripening of fruit (Lee et al. 2021).
T6P is a minor sugar that acts as a potent signal in plants, playing a key role in regulating sucrose levels (Figueroa and Lunn 2016; Durán-Soria et al. 2020; Fichtner and Lunn 2021). T6P regulates the production of sucrose in source organs against sink demand. Additionally, T6P regulates sucrose utilisation in sink organs for growth or storage. In Arabidopsis thaliana L., there is a reduction in T6P concentration when carbon starvation occurs, signalling low sucrose availability (Lunn et al. 2014). In contrast, increased T6P concentration in leaves inhibits the remobilisation of transitionary starch to sucrose (Fichtner and Lunn 2021). Yadav et al. (2014) indicated that T6P has a dual function as a signal and negative feedback regulator of sucrose. In addition, T6P metabolism in guard cells influences stomatal conductance, meaning T6P may affect photosynthesis (Figueroa and Lunn 2016).
T6P may regulate development by signalling the plant’s capacity to supply sucrose for upcoming development (Fichtner and Lunn 2021). In reproductive development, there is a connection between T6P and the timing of flowering (Figueroa and Lunn 2016). In the buds of mandarin (Citrus reticulata L.), trehalose metabolism associated genes were upregulated in years when cropping was limited by flowering (Shalom et al. 2012). In some species such as cucumber (Cucumis sativus L.), pre-existing fruit inhibit the growth of subsequent fruit (Smith and Samach 2013; Zhang et al. 2015). High concentrations of T6P in the first fruit increase sink strength to the detriment of the others.
Phytohormone-sugar crosstalk
Phytohormones control growth and development in plants (Weyers and Paterson 2001). Sugars interact with phytohormones to form a network for regulating development, including fruiting (León and Sheen 2003; Durán-Soria et al. 2020) (Fig. 2). Interactions between sugars and phytohormones ensure that hormone-driven processes are compatible with plant carbon status (Ljung et al. 2015). Responses that promote growth can be depressed under limited availability of NSC.
Interaction between sugar and phytohormones during fruit growth. Gibberellins and auxins promote fruit growth through cell division and expansion. Auxins inhibit sucrose accumulation and stimulate the degradation of sucrose to fructose and glucose via invertase, enhancing growth. Auxins also delay ripening. Sucrose promotes ripening and stimulates abscisic acid (ABA) and ethylene biosynthesis, while ABA and ethylene enhance sucrose accumulation. ABA also regulates ethylene biosynthesis. Adapted from Durán-Soria et al. (2020).
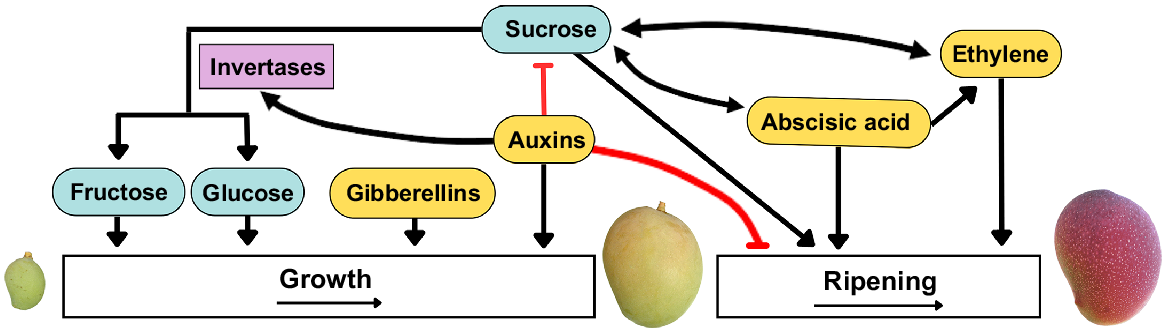
Phytohormones, such as ABA and ethylene, have crucial roles in fruit growth, ripening and senescence (Lin et al. 2009; Gupta et al. 2022). During ripening in climacteric and non-climacteric fruit, ABA regulates ethylene biosynthesis and signalling (Gupta et al. 2022).
Sugar signalling is a key regulator of ripening in climacteric and non-climacteric fruit (Cherian et al. 2014). In strawberry (Fragaria × ananassa L.), sucrose stimulates ABA production and facilitates ripening (Jia et al. 2013; Luo et al. 2020). In apple (Malus domestica L.), ABA signals sugar transport and starch degradation to promote sugar accumulation in fruit (Ma et al. 2017). The interaction between ABA and sugar signalling for mango ripening has received little attention. However, ABA–sugar crosstalk possibly affects flower induction (Upreti et al. 2013).
In mango and other climacteric fruit, ethylene is the dominant regulator of ripening (Tharanathan et al. 2006; Chen et al. 2018). Ethylene promotes sucrose accumulation in fruit, and exogenous ethylene advances sugar accumulation (Chidley et al. 2017). Similarly, exogenous sucrose stimulates ethylene biosynthesis (Durán-Soria et al. 2020). Finally, exogenous ABA accelerates ripening and ethylene biosynthesis (Zaharah et al. 2013). ABA, ethylene and sugar all interact to affect ripening in mango (Wu et al. 2022).
During early fruit development, gibberellins and auxins promote cell division and expansion, determining fruit size (Devoghalaere et al. 2012; El-Sharkawy et al. 2017; He and Yamamuro 2022). Other hormones, such as cytokinins, brassinosteroids, jasmonic acids and strigolactones are also involved (Kumar et al. 2014). Cytokinins regulate fruit growth by promoting cell division, while brassinosteroids and jasmonic acids affect ripening.
Decreases in the concentration of gibberellins are important for NSC accumulation in mango buds and for flowering (Das et al. 2019). The growth regulator paclobutrazol, a gibberellin inhibitor, is applied to broad-acre and tree crops, including mango, to reduce vegetative growth and to induce flowering. Paclobutrazol inhibits gibberellin synthesis by blocking the oxidation of ent-kaurene (Sponsel 1995), prompting an increase in sucrose concentrations in mango shoots (Rahim et al. 2011). By inhibiting vegetative growth, paclobutrazol increases the concentrations of stored NSC in trees, favouring fruit development (Yeshitela et al. 2004; Menzel and Le Lagadec 2017).
Auxins and jasmonic acids are two additional phytohormones that interact with sugars to influence reproductive development in fruiting crops, as discussed by Durán-Soria et al. (2020). Auxins stimulate invertase activity, which catalyses the hydrolysis of sucrose into glucose and fructose (Morris and Arthur 1984). However, specific roles of auxins and jasmonic acids in reproductive development remain largely unknown. Their involvement in NSC metabolism may be more indirect, possibly mediated through interactions with ABA and/or ethylene.
Pigments
Anthocyanins and carotenoids contribute to the colour of fruit (Ranganath et al. 2018). Anthocyanins are flavonoids, and accumulate in mango peel, providing a red colour, while carotenoids are responsible for yellow or orange colours and accumulate in the peel and flesh (Ni et al. 2022). The shikimate pathway, the foundation for flavonoid biosynthesis, consumes 20–50% of the carbon fixed by plants, making it a substantial carbon sink (Tohge et al. 2013). Crosstalk between sugars and phytohormones modulates anthocyanin biosynthesis in the presence of light (Das et al. 2012).
In Arabidopsis, exogenous sucrose upregulates genes in the phenylpropanoid pathway inducing anthocyanin synthesis (Das et al. 2012; Durán-Soria et al. 2020). In kiwifruit (Actinidia deliciosa L.), Nardozza et al. (2020) showed that carbon starvation following girdling, reduced the concentration of sucrose and T6P, and caused a transcriptionally induced cessation of anthocyanin synthesis in fruit. It was suggested that T6P availability may affect anthocyanin biosynthesis. Lueangprasert et al. (2010) found that applying fructose to mango peel stimulated anthocyanin, especially on the sun exposed sections of the fruit.
Carotenoids are involved in the absorption of energy for photosynthesis and the protection of chlorophyll from excessive light (Armstrong and Hearst 1996). In mango peel, carotenoid accumulation occurs when chloroplasts develop into chromoplasts (Medlicott et al. 1986; Cheung et al. 1993). Carotenoid and sugar accumulation in fruit is concomitant (Li and Yuan 2013). In mandarin, exogenous sucrose to peels accelerates the breakdown of chloroplasts to chromoplasts (Iglesias et al. 2001). Sucrose regulates carotenoid metabolism through the regulation of carotenoid biosynthesis genes (Sadali et al. 2019). For example, starch conversion into sugars during tomato (Solanum lycopersicum L.) fruit ripening is correlated with carotenoid accumulation (Li and Yuan 2013). Sucrose depletion in tomato influences expression of PSY1, a gene involved in carotenoid accretion, thereby delaying carotenoid accumulation (Télef et al. 2006). Sugar starvation, for example, as induced by defoliation inhibits the accumulation of carotenoid in the fruit (Li and Yuan 2013).
Leaves: the main source
Fully expanded mature leaves are the primary source of NSC in trees. For NSC to be used for growth, they must be generated in the leaves and transported to a sink (Kozlowski 1992; Blanke 2009). Photosynthesis and sugar export are upregulated under low sugar levels, under strong fruiting or vegetative growth (Rolland et al. 2006).
Carbon assimilation
Synthesis of NSC in plants starts with photosynthesis. Light is converted to chemical energy in the form of adenosine triphosphate (ATP) and nicotinamide adenine dinucleotide phosphate (NADPH) by chlorophyll (Avenson et al. 2005). The chemical energy is used to convert CO2 and water into sugars. Mango peel contains chlorophyll, particularly early in development. However, the rate of photosynthesis is low compared with that occurring in the leaves (Chauhan and Pandey 1984). The carbon that is fixed during photosynthesis can be metabolised to provide energy and carbon skeletons for respiration and growth or is stored as reserves.
The greater the rate of photosynthesis, the more sucrose is exported and is available for sinks (Ho 1976; Lanoue et al. 2018). Starch is produced when sucrose formation exceeds the capacity for export or the demand for NSC from sinks (Zeeman et al. 2007; Weise et al. 2011). Starch can be degraded and converted back to sucrose when the demand for sugar outweighs supply, for example, during the night (Zeeman et al. 2007).
The rate of photosynthesis is affected by phenology and the temperature, humidity and light (Pessarakli 1996; Kaiser et al. 2015). Net photosynthesis (Anet) in C3 species such as mango is a balance between the CO2 fixation and respiration (Tcherkez and Limami 2019). Stomatal conductance (gs) controls Anet in mango with a high gs corresponding with high Anet (Urban et al. 2002, 2006; Lu et al. 2012).
Photosynthetic capacity (Amax) refers to the maximum rate at which a leaf can fix carbon (Smith et al. 2019). The leaf nitrogen concentration, although not strictly determining Anet, may be rate limiting and regulates Amax of mango (Urban et al. 2003). Amax is related to leaf nitrogen because the proteins of the Calvin cycle and thylakoids represent the majority of leaf nitrogen (Evans 1989). Leaf nitrogen concentration per unit area exhibits a positive non-linear relationship with Amax regarding maximum carboxylation rate (Vcmax), electron transport capacity (Jmax), rate of phosphate release in triose phosphate utilisation (TPU), and dark respiration (Urban et al. 2003).
The regulation of photosynthesis is linked to leaf NSC concentration and composition (Goldschmidt and Huber 1992; Paul and Pellny 2003; Lloyd and Zakhleniuk 2004). Accumulation of end-products such as starch, sucrose, and hexose sugars introduces negative feedback, downregulating Amax and disrupting gene expression (Goldschmidt and Huber 1992). Inorganic phosphate (Pi) further complicates this regulation, with the rate of end-product synthesis determining the recycling of Pi back into the photosynthetic reaction, potentially leading to feedback inhibition (Paul and Pellny 2003). Pi recycling is closely intertwined with leaf carbon metabolism. Although the hexose-to-sucrose ratio may influence photosynthesis, the specific relationships with expression of photosynthetic genes remain elusive (Lloyd and Zakhleniuk 2004). The ambiguity extends to the relative impact of hexose or sucrose accumulation on photosynthetic inhibition (Lobo et al. 2015).
Respiration occurs where sugars are converted to energy for cell division, expansion, senescence, translocation and the storage of metabolites (Jackson 2003). For mango and other C3 plants, 30–60% of photosynthates are consumed by respiration (Kozlowski 1992; Fischer et al. 2013; Amthor et al. 2019). Respiration can be divided by function as tissue maintenance or growth (Fischer et al. 2013), or by light dependence as photorespiration or dark respiration. Maintenance respiration occurs in all tissues and affects the dynamics of NSC in fruiting crops. Maintenance respiration is a priority over growth when photosynthates are limited (Génard et al. 2008).
The effect of fruit on dark respiration in leaves is inconclusive. Wünsche and Ferguson (2010) found that fruiting increases dark respiration in apple, whereas Watson et al. (1978) found the opposite. In grapevine (Vitis vinifera L.), respiration in fine roots was higher in plants without fruit likely because root activity was downregulated under higher crop load (Morinaga et al. 2003).
Prior to harvest, respiration in mango fruit increases along with an increase in soluble solids (Léchaudel and Joas 2006). There is a negative relationship between respiration and postharvest shelf life (Ravindra and Goswami 2008).
Leaf source activity
Although leaves are the primary source for NSC, the contribution of individual mango leaves vary (Chacko et al. 1982). Young expanding leaves are net sinks compared with older leaves which are sources (Zude and Luedders 1997; Fischer et al. 2013). Photosynthesis eventually starts to decline in old senescent leaves (Karim et al. 1999).
In mango, the leaves export sucrose in both acropetal and basipetal directions, with acropetal transport dominant during flowering and fruit development, and basipetal transport dominant during flower bud differentiation (Chauhan and Pandey 1984). The translocation of sugars to fruit peaks when fruit are half-grown to fully-grown.
Fruit: the predominant sink
Sink strength indicates the ability to import sugars compared with other sinks and is the product of sink size and activity (Marcelis 1996; Bihmidine et al. 2013). Sink strength represents the maximum rate a tissue can accumulate dry matter (Génard et al. 2008). Sink activity is governed by phloem unloading and transport and absorption of sugars into sink cells (Génard et al. 2008). Ultimately, sinks utilise NSC for respiration, growth and storage (Pawar and Rana 2019).
Mango fruit have different sink strength during development (Fig. 3). The fruit exhibits a sigmoid pattern of growth, with slower initial growth followed by several months of rapid growth before the fruit stabilise (Simmons et al. 1998). Carbon isotope tracing showed that the sink strength peaks when fruit are half-grown to fully-grown (Chauhan and Pandey 1984). Flower buds and marble sized fruit are weak sinks, whereas flowers and pea-sized fruit are intermediate.
A model depicting the evolution of reproductive sink size, activity, and strength from budswell to fruit ripening in mango. Based on insights from Chauhan and Pandey (1984) and Simmons et al. (1998).
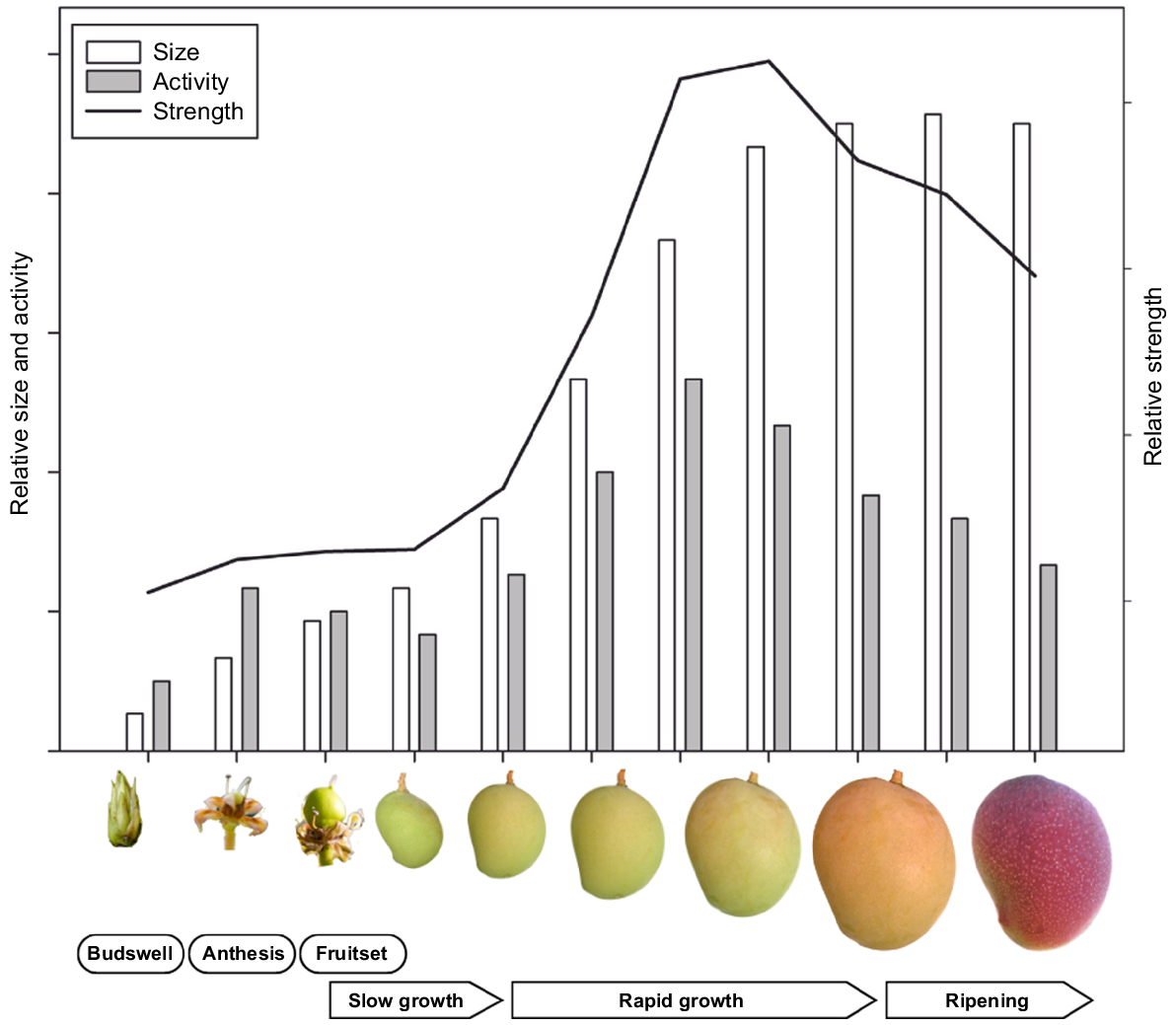
In mature mangoes, NSC represent 90–94% of the dry mass, with the rest being organic acids, lipids and proteins (Bello-Pérez et al. 2007). NSC levels are stable in the initial fruit growth, however, accumulation of NSC primarily occurs as starch during rapid growth (Tandon and Kalra 1983). The rate of starch accumulation and amylase activity matches the growth rate, slow initially, rapid intermediately and plateauing before maturity. As the fruit ripen, sugars, primarily sucrose, accumulate as a result of the hydrolysis of starch (Malundo et al. 2001). Fruit should be harvested at the right stage when starch is converting to sugar to maintain quality and shelf life (Simão et al. 2008; Yu et al. 2022). Fruit starch maintain shelf life by providing carbon for respiration. Starch also contributes to fruit firmness, with the fruit softening when the starch is converted to sugars, improving sweetness and flavour.
Individual fruit compete for NSC, along with growing shoots, roots and leaves (Pawar and Rana 2019). The proximity of the sink to the source is important in determining a sink’s competitive ability (Patrick 1988). Active sinks on a branch are fed by the nearest sources provided sources are sufficient to meet sink activity (Sprugel et al. 1991). Fruit closer to the main stem are larger than those further away because they have access to current assimilates and NSC remobilised from the roots and wood (Singh et al. 2017).
Contribution of sugars to reproductive development
Sugars play a key role in plant reproductive development by serving as fuel for cellular carbon and energy metabolism, while also acting as key signalling molecules (Rolland et al. 2006). The interplay between photosynthesis and NSC metabolism in source and sink organs generates diverse sugar signals that modulate reproductive processes. Starch mobilisation and the availability of sucrose emerge as crucial factors promoting flower induction and flowering. Additionally, the activity of invertases and the ensuing sugar metabolism contribute to reproductive processes, with hexoses playing a stimulatory role in the progression of both flower and fruit development (Ruan 2012).
Flower induction is associated with the mobilisation of starch and an increase in leaf sugar export, which may also transport flowering-related hormones to the flowering bud (Rolland et al. 2006; Davenport 2009; Lauri and Normand 2017). Greater NSC content in terminal shoots corresponds with heavier flowering (Pongsomboon et al. 1997). Flower abscission is also correlated with lower concentrations of sugars (Sawicki et al. 2015).
In mango, sugars produced in leaves are a potential driving force for phloem-based transport of a flowering promoter (Davenport 2007). The accumulation of NSC in the shoot apex is crucial for floral induction (Davenport 2009). The concentration of sucrose is highest in terminal and sub-terminal shoots at flowering, with roots, the trunk, stems and leaves exhibiting relatively lower concentrations (Normand et al. 2006). Higher concentrations of NSC or higher carbon-to-nitrogen ratio in a shoot increases the probability of flowering (Corbesier et al. 2002; Prasad et al. 2014).
Sucrose metabolism regulates flowering, along with T6P signals and gibberellins (Rolland et al. 2006; Sharma et al. 2019). Sucrose and its metabolising enzymes, notably sucrose phosphate synthase, appear to increase in leaves and terminal buds to trigger mango flowering, highlighting the important role of sucrose signalling to induce flowering (Prasad et al. 2014; Kaur et al. 2022). Increased supply of sucrose to the flowering shoot is also important for the emergence of panicles, while sucrose transporters are involved in determining flowering time (Liang et al. 2022). Moreover, the sucrose synthase signal associated with flowering may be mediated through T6P, as evidenced by its upregulation in the inflorescence (Kaur et al. 2022). TPS1 transcripts, involved in T6P synthesis, are expressed leading up to mango flowering, which could trigger T6P induced flower induction signals (Liang et al. 2022). Sucrose seems to function primarily in the leaf phloem to enhance the generation of florigens such as FLOWERING LOCUS T (FT), while T6P functions in the shoot apical meristem to promote the flowering signal pathway downstream of those florigens (Liang et al. 2022).
Horticultural practices aimed at manipulating flowering in mango trees, such as trunk or branch girdling and the application of plant growth regulators like ethephon and paclobutrazol, are closely linked to changes in NSC dynamics (Goren et al. 2003; Davenport 2007; Desta and Amare 2021). Girdling disrupts the phloem transport of sugars between the canopy and roots, offering a means to manipulate sugar distribution (Goren et al. 2003). In mango and other fruit trees, this manipulation, in turn, has the potential to reduce vegetative growth, induce flowering, and enhance fruit set and growth. Above the girdle, NSC accumulates, particularly in shoot terminals, leading to an improved carbon-to-nitrogen ratio and, consequently, enhanced flowering. Greater allocation of sugars to fruit following girdling also improves fruit size, dry matter and peel anthocyanin accumulation.
In lychee (Litchi chinensis L.), foliar application of the ethylene precursor, ethephon (2-chloroethylphosphonic acid), can suppress new vegetative growth before flower induction, promoting flowering (Cronje et al. 2022). Although less studied in mango, ethephon has shown promising flowering-inducing properties for the crop (Davenport 2009). In lychee, ethephon induces bud dormancy, delays panicle emergence, and enhances flower development (Cronje et al. 2022). By inhibiting new growth and extending bud dormancy, ethephon prolongs the period for NSC reserve accumulation in terminal shoots, resulting in higher levels of starch and sugars at floral initiation, thereby contributing to improved flowering.
The triazole plant growth retardants, paclobutrazol and uniconazole, inhibitors of gibberellin biosynthesis, stimulate floral induction in mango (Davenport 2007; Rahim et al. 2011). By suppressing vegetative growth, they redirect sugars, originally available for vegetative growth, towards enhancing flowering, particularly through increased allocation to reproductive shoots (Yeshitela et al. 2004; Desta and Amare 2021).
Fruit set in tree crops such as mango is regulated by the availability of sugars (Jannoyer and Lauri 2006). If flowering is heavy, sugar may limit the fruit set and growth causing the tree to rely more on NSC reserves (Chacko et al. 1982; Jannoyer and Lauri 2006).
Fruit abscission generally occurs during three stages: (1) shortly after fruit set; (2) mid-season; and (3) before harvest (Singh et al. 2004). Abscission after fruit set is due to poor fertilisation, hormonal signals and NSC related crosstalk, under high ethylene and low auxins (Singh et al. 2004; Xie et al. 2013; Sawicki et al. 2015). ABA also plays a key role in the initiation of an abscission layer. In contrast, high concentrations of gibberellins, cytokinins and auxins, are positively correlate with fruit retention (Singh et al. 2004; Xie et al. 2013). The availability of NSC is critical for fruit retention during later stages of development. Fruit and shoots may compete for NSC and accelerate abscission (Singh et al. 2004; Xie et al. 2013).
Hormones and sugars regulate abscission in plants (Sawicki et al. 2015). For example, in mandarin (C. unshiu), defoliation increases fruitlet abscission due to low sugar supply, an increase in ABA and ethylene, and a decrease in gibberellin (Gómez-Cadenas et al. 2000). Conversely, increased NSC supply decreases ethylene production and fruit abscission. Limited NSC supply to lychee fruitlets decreases auxin concentrations causing abscission (Kuang et al. 2012). In Arabidopsis, an overexpression of the T6P synthase gene AtTPS1, which regulates ABA signalling, occurs in abscising fruitlets (Avonce et al. 2004; Sawicki et al. 2015). Hence, T6P signalling may regulate ABA’s stimulatory role in fruitlet abscission. Taken together, low sugar levels appear to reduce hormonal growth stimulants and increase stress signals, triggering abscission.
Research on the link between sugar, signalling and hormones in mango fruit retention is scarce. Sakhidin et al. (2011) found low sugar and high starch content predicted fruit abscission. This might be due to a reduction in starch metabolism in the pre-abscised fruit, allowing an accumulation of starch. Ethephon induces fruitlet abscission, associated with an upregulation of ethylene receptors and a decrease in sucrose (Hagemann et al. 2015).
Relationship between fruit and leaves
Increased sink demand by developing fruit improves photosynthetic efficiency in crops (Aluko et al. 2021). For example, in peach (Prunus persica L.), DeJong (1986) showed Anet during early fruit development was similar in fruited and defruited trees. However, closer to fruit maturity, Anet was 11–15% higher in fruiting trees. Fruit increases Amax in mango and the concentration of nitrogen per leaf area (Urban et al. 2003).
Leaf Anet is affected by the leaf-to-fruit ratio (Urban et al. 2003, 2004a; Urban and Léchaudel 2005). A lower leaf-to-fruit ratio increases Anet, probably by exhausting NSC stored in the leaves (Urban et al. 2002). Partial defoliation of fruit trees increases photosynthesis in remaining leaves (Fischer et al. 2013; Pawar and Rana 2019). In contrast, a high leaf-to-fruit ratio increases leaf starch and inhibits photosynthesis (Urban et al. 2003, 2004a; Léchaudel et al. 2005a; Urban and Léchaudel 2005). Under low crop load, the accumulation of starch in leaves during the day reduces gs and leaf nitrogen (Simmons et al. 1998; Urban et al. 2004a; Urban and Léchaudel 2005).
Accumulation of NSC in leaves under sink limitation, such as low crop load, may result in reduced TPU (Fabre et al. 2020), representing a limitation for Amax in mango (Urban et al. 2003). TPU signifies the rate at which triose phosphates are utilised for synthesising sugars and starch within leaves (Sharkey 2019; Zhou et al. 2023). Under sink limitation, NSC accumulate in leaves, and feedback inhibition on further sugar synthesis can inhibit TPU (Zhou et al. 2023), meaning TPU potentially modulates the influence of the leaf-to-fruit ratio on leaf source activity and sugar synthesis in mango.
In terms of the relationships between reproductive development and photosynthesis, the proximity of fruit to leaves enhances or inhibits Anet, depending on the stage of fruit development (Shivashankara and Mathai 2000; Urban et al. 2004b). Flowering is associated with a decrease in Anet in leaves near inflorescences (Urban et al. 2004b, 2008). The reduction in photosynthesis is due to a decrease in electron flow in PSII, gs, mesophyll conductance and Jmax. A decrease in photorespiration may also contribute to lower Anet for leaves near flowers. Leaf nitrogen concentration is lower in leaves near inflorescences compared with leaves on vegetative shoots. Shivashankara and Mathai (2000) indicated that low Anet in flowering branches was due to lower carboxylation efficiency. After fruit set, Amax is positively related to the proximity of the leaves to fruit, independent of light and leaf age (Urban et al. 2003). This response is associated with higher nitrogen concentration in leaves and a greater mass-to-area ratio rather than to NSC.
The contrasting effects of flowering and fruit growth on leaf nitrogen may be attributed to the availability of nitrogen at different stages of tree development. Total tree nitrogen content is lowest at flowering and increases after fruit set, especially in the leaves (Stassen et al. 2000). Flowering decreases leaf nitrogen due to the diversion of nitrogenous compounds from leaves to sinks such as the inflorescences (Durán-Zuazo et al. 2005). During fruit growth, the leaves and fruit accumulate nitrogen, indicating increased soil nitrogen uptake during this period (Stassen et al. 2000), suggesting that nitrogen is primarily directed towards the fruit and nearby leaves.
Effect of crop load on reproductive development
High yields are important for profitability in mango cultivation. However, a heavy crop that draws on NSC reserves may reduce yield the following year. Depleted NSC reserves can lead to lower vegetative growth, resulting in less NSC accumulation to support production in the following year, thus, causing irregular bearing (Monselise and Goldschmidt 1982; Génard et al. 2008; Davenport 2009; Das et al. 2019). High yields are commonly achieved by improving harvest index, the ratio of yield-to-plant dry matter, rather than by improving photosynthesis (Génard et al. 2008). However, yield per land area is increased by enhancing leaf area and light interception (Menzel and Le Lagadec 2017).
The effect of leaf-to-fruit ratio on mango fruit development is well investigated (Chacko et al. 1982; Reddy and Singh 1991; Simmons et al. 1998; Lechaudel et al. 2002; Léchaudel et al. 2005b; Urban and Léchaudel 2005; Léchaudel and Joas 2006; Léchaudel and Joas 2007; Grechi and Normand 2019). Typically, a shoot or branch with a set number of leaves and fruit was girdled to inhibit phloem translocation of sugars from external NSC sources. The results of these studies demonstrate that a low leaf-to-fruit ratio decreases the supply of NSC supply to the fruit. Fruit size and dry matter accumulation generally increase with the number of leaves per fruit (Fig. 4). It has been suggested that more than 30 to 50 leaves are required to support the growth of a single mango without support from reserves (Chacko et al. 1982; Reddy and Singh 1991).
A logarithmic model for the meta-analysis of leaf-to-fruit ratio on fruit size (top) and fruit dry matter content (bottom) across regions, cultivars and tree ages. There was a significant exponential relationship for both fruit size (adj. R2 = 44.2) and dry matter content (adj. R2 = 29.1). Branches were girdled and either fruit or leaf removed. Data has been centred and scaled within each study and cultivar. Data for both trends has been drawn from Chacko et al. (1982); Simmons et al. (1998); Lechaudel et al. (2002); Léchaudel and Joas (2006) and Grechi and Normand (2019); and for fruit size from the additional studies: Reddy and Singh (1991); Urban and Léchaudel (2005) and Singh et al. (2007).
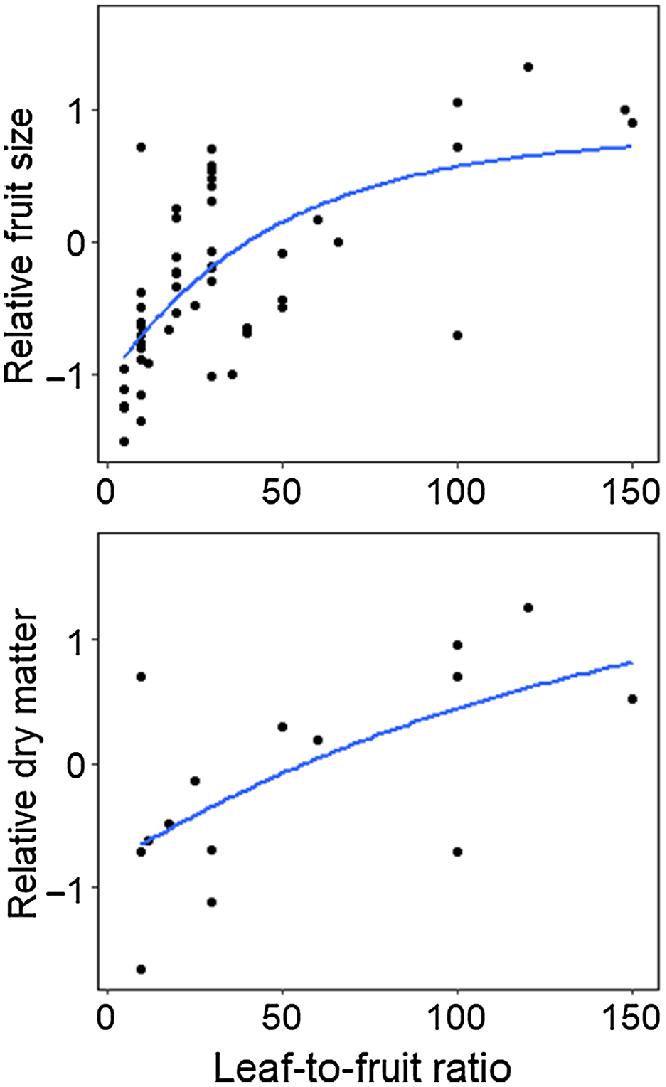
In high cropping seasons or cultivars, there are often fewer than 30 leaves to support each fruit. Therefore, the balance between sources and sinks is maintained by NSC reserves (Fig. 1), which in turn is affected by the activities of the sources and sinks (Léchaudel et al. 2005a). Fruit set competes for limited NSC resources, and when flowering is heavy, the NSC may be inadequate for fruit set and growth (Jannoyer and Lauri 2006). Fruit retention is also affected, where ten leaves per fruit is inadequate (Grechi and Normand 2019).
Pruning or fruit thinning can be used in orchards to manipulate source or sink size and alter fruit growth. Increasing the leaf-to-fruit ratio by fruit thinning results in larger fruit. In contrast, increasing the leaf-to-fruit ratio above 50 does not result in further increases in fruit size (Léchaudel et al. 2005a). Thus, when NSC limitations are removed, fruit growth is limited by the sink strength. When fruit size is not limited by the availability of NSC, excess carbohydrates are stored.
When the fruit are mature, sucrose concentration and starch hydrolysis correlate positively with an increasing leaf-to-fruit ratio. During ripening, a greater leaf-to-fruit ratio increases fruit respiration and the synthesis of the ethylene precursor, 1-aminocyclopropane-1-carboxylic acid (ACC) (Léchaudel and Joas 2006). A higher leaf-to-fruit ratio increases the accumulation of dry matter (Fig. 4) (Génard et al. 2008). In contrast, a low leaf-to-fruit ratio delays the degradation of starch to sucrose in mature fruit and increases glucose and fructose content on a dry mass basis (Léchaudel et al. 2005b; Léchaudel and Joas 2007).
NSC dynamics also contribute to physiological disorders in mango, particularly the development of spongy tissue disorder in mature fruit (Shivashankar and Sumathi 2019). This disorder, characterised by internal breakdown of mango flesh, is often observed in specific cultivars, including ‘Alphonso’ and ‘Tommy Atkins’. Fruit affected by spongy tissue disorder exhibit lower amylase activity and sugars during ripening, whilst having higher starch content compared to healthy fruit. Increased competition for resources, such as NSC, among developing fruits for seed development is believed to play a crucial role in the occurrence of spongy tissue disorder (Shivashankar and Sumathi 2019). Consequently, amylase activity is reduced, and starch degradation is impeded in affected fruit.
Reserves and storage organs
Roots, trunks, branches and shoots predominantly function as sinks. Nevertheless, some organs undergo transitions from sinks to sources, such as roots and stems, which can transiently shift from storage sinks to sources (Sonnewald and Fernie 2018). The storage organs serve as energy reserves for future growth and development.
In mango, the fruit represents the principle sink and hence, secondary NSC sinks accumulate starch only when the fruit sink strength declines or is absent (Stassen and Janse van Vuuren 1997; Davie et al. 1999) (Fig. 5). The concentration of starch in vegetative tissues increases through bud development and peaks prior to flowering (Davie et al. 1999; Normand et al. 2006). The majority of the starch is stored in thick roots and woody tissues, mainly roots larger than 10 mm in diameter and branches (Stassen and Janse van Vuuren 1997; Normand et al. 2009). Smaller contributions arise from bark, thin roots, scaffolding branches, shoots and terminal growth units.
Theoretical changes in distribution of starch from floral bud initiation to harvest in roots, bark, wood, shoots, leaves and fruit in mango. Inspired by Stassen and Janse van Vuuren (1997); Davie et al. (1999) and Normand et al. (2006).
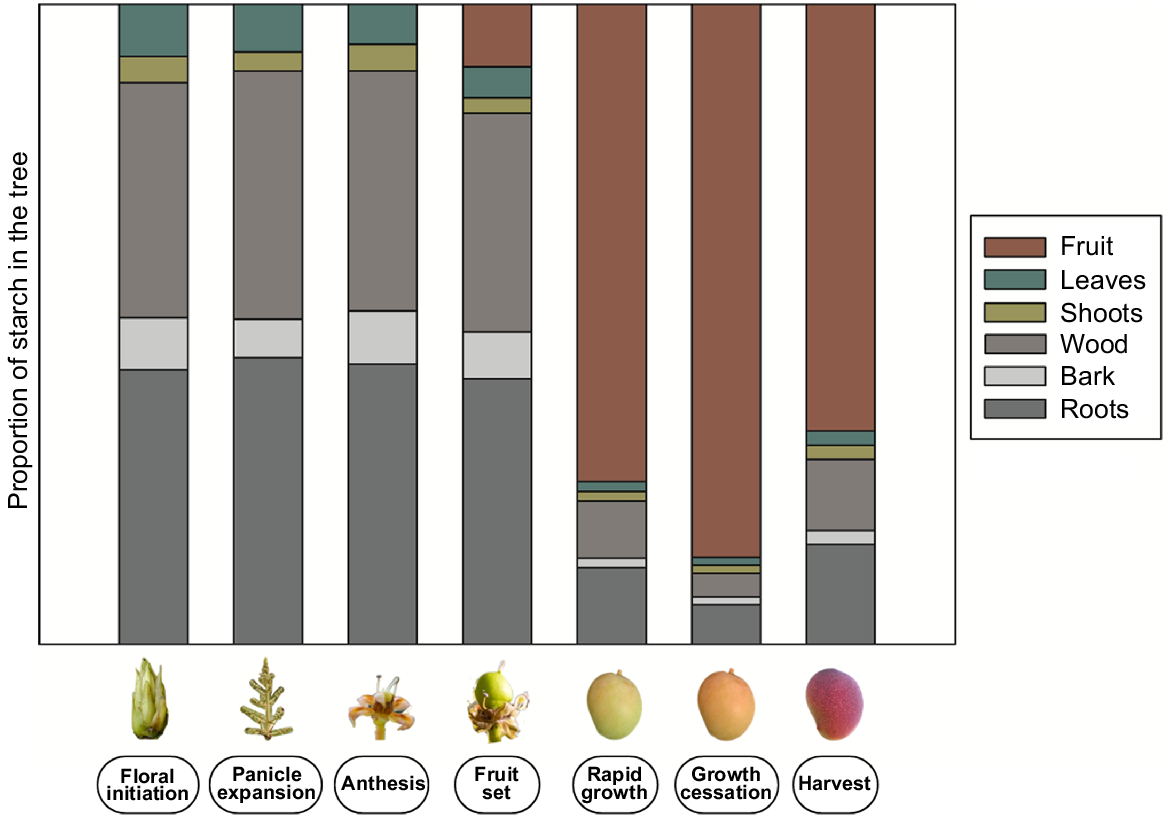
Remobilisation
The development of evergreen trees relies on current photoassimilates as the primary source of NSC (Olesen et al. 2008). However, reserves can buffer against fluctuations in the supply of current photosynthates (Fig. 1). During elevated photosynthate production, branches are primarily self-supporting (Sprugel et al. 1991). However, when the leaf area is reduced or sink demand is amplified, strong sinks can draw NSC from outside the branch. Root starch reserves are the first utilised for fruit development prior to the remobilisation of starch stored in woody tissues (Davie and Stassen 1997b). In fruiting species, remobilisation from storage tissues occurs when sink requirements exceed source production (Loescher et al. 1990; Candolfi-Vasconcelos et al. 1994; Han et al. 2016; Rossouw et al. 2017b; Breen et al. 2020).
Roots and woody tissues such as the trunk, branches, bark and shoots act as sources rather than sinks during flowering, spring vegetative growth flush, fruit set and fruit growth when their starch is depleted (Stassen and Janse van Vuuren 1997; Davie et al. 1999). The demand for NSC exceeds the current supply from photosynthesis, resulting in the mobilisation of reserves. At flowering, roots contain higher concentrations of starch than other vegetative tissues, particularly coarse roots, the taproot and stump (Normand et al. 2006). Medium roots (2–10 mm in diameter), the trunk, branches and older leaves have intermediate starch levels, whereas fine roots (<2 mm in diameter) and terminal and subterminal growth units of shoots have the lowest starch levels.
Effects of fruiting on reserves
The impact of fruiting on NSC and on vegetative and reproductive development have been extensively studied in deciduous fruit crops (Holzapfel et al. 2010; Fischer et al. 2013; Rossouw et al. 2017b). However, research in this area is scarce for evergreen species such as mango. Intra-annual variation in NSC concentration might not vary to the same extent as in deciduous species. However, there is still cycles due to seasonal and phenological changes in evergreen species (Normand et al. 2006).
In grapevine, a high yield leads to a depletion of starch reserves at harvest (Rossouw et al. 2017a). This results in lower yields in the following season (Smith and Holzapfel 2009). In contrast, when apple trees were defoliated causing NSC reserves to deplete, the yield for the next season was unaffected (Breen et al. 2020). The role of NSC reserves in inter-annual yields varies amongst deciduous species and between deciduous and evergreen species.
The importance of reserves for reproductive growth in deciduous crops depends on the timing of flowering and fruit development (Loescher et al. 1990). If flowering occurs before the canopy is formed, there is greater reliance on reserves for fruiting. However, yield also influences the role of NSC reserves as they are necessary to balance source production and sink demand.
Bearing pattern
Biennial or alternate, and irregular bearing occurs in mango and are influenced by the cultivar, agronomic practices, and the environment (Shivashankara and Mathai 2000; Sharma et al. 2020). These phenomena all contribute to low productivity (Pandey 1989). Irregular bearing is characterised by an irregular pattern of fruiting across different seasons (Smith and Samach 2013; Sharma et al. 2019) (Fig. 6). In biennial bearing, a heavy yield is followed by a light yield in the following year (Sharma et al. 2019). ‘Off’ and ‘on’ years are often associated with cycles of carbohydrate reserves (Fig. 6).
Starch regulation in fruiting and non-fruiting terminal shoots contributes to the distinctive flowering patterns in regular (top left), biennial (top right), and irregular bearing (bottom) mango cultivars. Larger box size corresponds with a greater proportion. Regular bearers maintain high starch concentrations in both previously fruiting and non-fruiting terminals, ensuring consistent flowering and fruiting cycles. Biennial bearers, experiencing seasons of abundant flowering, exhibit reduced terminal starch levels in subsequent cycles, resulting in poor flowering before recovery. Irregular bearers display a less predictable pattern, with previously fruiting terminals having lower starch and previously non-fruiting terminals having higher starch in the next cycle. The balance between these terminal types, along with factors like abiotic conditions, influences the irregular bearing pattern.
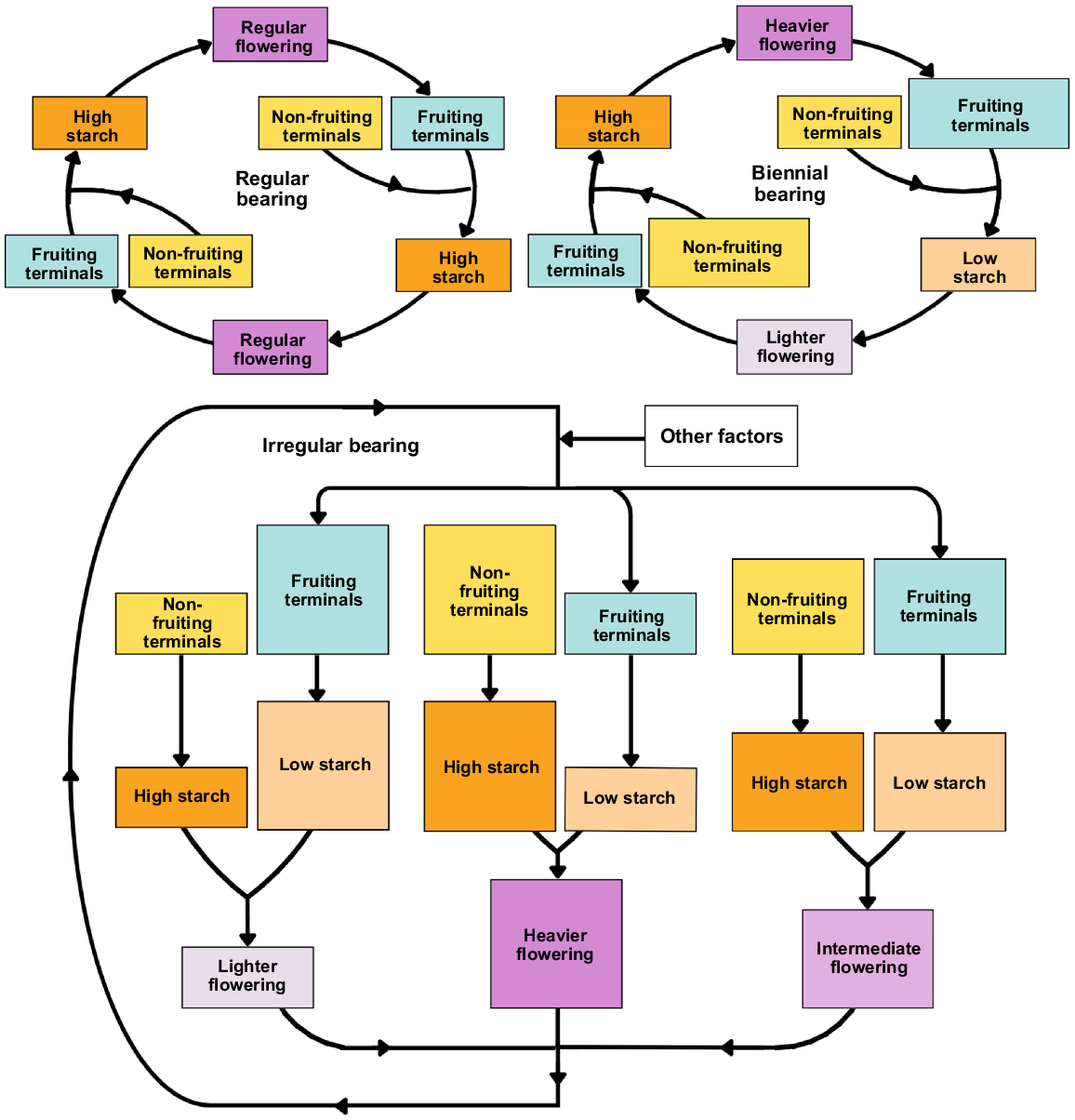
Links between bearing pattern and NSC
Reserve NSC concentrations can limit flower and fruit development (Whiley et al. 1989; Normand et al. 2006), as high NSC at fruit bud differentiation is positively correlated with yield in mango. A high yield provides a strong sink for NSC, limiting flowering the following season (Chacko et al. 1982; Fischer et al. 2013; Sharma et al. 2019). Limited vegetative growth also results from low NSC and reduces the number of terminal growth units that can flower (Normand et al. 2017). Low NSC limits fruit set and development resulting in biennial or irregular bearing (Davie and Stassen 1997b).
Yields across different seasons are related to the availability and replenishment of starch reserves (Reddy and Singh 1991; Capelli et al. 2021). High starch levels in the leaves and stems are correlated with better flowering and yields (Suryanarayana 1978; Monselise and Goldschmidt 1982). Branch terminals have low concentrations of starch after harvest, and starch accumulation is slower in fruiting terminals than quiescent and flowering terminals (Normand et al. 2017). Vegetative flushing after harvest further depletes starch accumulation (Capelli et al. 2021). In contrast, in non-fruiting growth units, starch accumulates after harvest. This is why previously fruiting growth units are less likely to have timely vegetative growth. Decreased vegetative growth, in turn, limits flowering and fruiting in the following cycle.
Differences amongst cultivars
Irregular bearing cultivars have high fluctuations in yield over different seasons and are highly influenced by abiotic factors such as temperature and water supply (Sharma et al. 2019) (Fig. 6). In contrast, biennial bearing cultivars have an on-and-off yields (Fig. 6). Das et al. (2019) and Capelli et al. (2021) found that irregular and biennial bearing cultivars differ in their storage and use of starch. Differences in starch between fruiting and non-fruiting terminals are greater in irregular ‘Cogshall’ than in biennial ‘José’ after harvest (Capelli et al. 2021). In regular ‘Amrapali’, buds and leaves had higher NSC than biennial ‘Dashehari’ (Das et al. 2019). In previously fruiting growth units on irregular bearing cultivars, low starch reduced vegetative growth compared with previously non-fruiting growth units. The proportion of non-fruiting growth units across the canopy dictates growth and potential flowering. This information can help guide canopy management in mango orchards. The key is to achieve a balance of fruiting and non-fruiting growth. Selective pruning can remove some of the fruiting terminals, providing a better balance between fruiting and non-fruiting terminals.
For biennial bearers, low starch in fruiting and non-fruiting terminals decrease vegetative growth. All terminals behave similarly leading to poor vegetative growth and flowering. Regular bearers maintain a balanced concentration of NSC each season, whereas biennial bearers have wide fluctuations in NSC concentration. In ‘on’ seasons, NSC levels drop for the biennial bearer, and in the ‘off’ season the biennial bearer exhibits lower reserves (Sharma et al. 2020).
Additionally, the number of leaves required to support a single fruit is higher in the biennial ‘Dashehari’ and ‘Langra’ compared with the consistent ‘Neelum’ and ‘Kalahandi’ (Reddy and Singh 1991). This response could be due to differences in fruit size, leaf area and photosynthesis. Lastly, rootstock selection could alter the bearing habit of scion cultivars by influencing the carbon-to-nitrogen ratio in buds (Vittal et al. 2023). For biennial ‘Dashehari’, the rootstock Kurukkan was able to improve the carbon-to-nitrogen ratio in buds as compared to another rootstock, Olour. A higher carbon-to-nitrogen ratio is conducive to flowering and may help reduce erratic bearing.
Conclusions
Starch–sugar metabolism regulates carbon allocation in fruit trees. Starch reserves in roots and wood are synthesised when sugars are abundant or degraded upon sugar starvation. The availability of starch reserves influences flowering, fruit set, and growth, with the inability to recover starch post-harvest leading to irregular bearing.
Sugars function as a mobile energy source and in signalling with phytohormones to stimulate floral induction, and fruit set, retention and growth. Sucrose signals modulate fruit development by regulating starch metabolism, maintaining cellular carbon homeostasis, while T6P is a potent signalling molecule to balance NSC supply with demand. In later fruit development, sugar-phytohormone cues regulate the accumulation of pigments such as anthocyanins and carotenoids in peels.
Fruit is the major NSC sink whereas leaves are the major source. When yields are low, leaf starch accumulates, leading to reduced photosynthesis. Conversely, high yields deplete leaf starch, and stimulates photosynthesis. Photosynthesis negatively correlates with the proximity of inflorescences to leaves during flowering, and positively correlates with the proximity of leaves to growing fruit.
Leaf activity peaks when fruit are approximately half to fully grown, corresponding to fruit sink demand. However, leaf NSC supply often falls short, necessitating the remobilisation of reserves. Larger roots, trunk, and branches store starch reserves, crucial for reproductive development, including flowering, fruit set, growth, and dry matter accumulation. These reserves are replenished when fruit activity declines. Insufficient photosynthate and starch storage lead to reduced fruit size and dry matter accumulation, impacting long-term productivity when crop load is excessive. Crop load management techniques, for instance, crop thinning, commonly used in temperate crops, could be considered for mango in high crop load years. This approach could help prevent the depletion of NSC reserves, potentially leading to more consistent bearing across seasons.
This review identifies various gaps in understanding the effects of NSC on fruiting and mango productivity. Exploring sugar signalling under a range of NSC availabilities, particularly T6P signalling, and investigating interactions between sugars, phytohormones and secondary metabolites during flowering and fruit development are crucial. Developing a carbon balance, considering the effects of crop load on NSC reserves and carbon assimilation in mango leaves, is essential. An improved understanding of NSC autonomy in terms of proximal and distal sources and their recharge during and after fruiting will contribute to managing irregular bearing effectively.
Declaration of funding
This work has been supported by the National Tree Crop Intensification in Horticulture Program (AS18000), funded by the Hort Frontiers strategic partnership initiative developed by Hort Innovation, with co-investment from Queensland’s Department of Agriculture and Fisheries, Queensland Alliance for Agriculture and Food Innovation – The University of Queensland, Plant and Food Research and the Western Australian Department of Primary Industries and Regional Development, and contributions from the Australian Government.
Acknowledgements
The authors thank Dr. Christopher Menzel for providing valuable feedback on the manuscript.
References
Aluko OO, Li C, Wang Q, Liu H (2021) Sucrose utilization for improved crop yields: a review article. International Journal of Molecular Sciences 22, 4704.
| Crossref | Google Scholar | PubMed |
Amthor JS, Bar-Even A, Hanson AD, Millar AH, Stitt M, Sweetlove JL, Tyerman SD (2019) Engineering strategies to boost crop productivity by cutting respiratory carbon loss. The Plant Cell 31, 297-314.
| Crossref | Google Scholar | PubMed |
Armstrong GA, Hearst JE (1996) Carotenoids 2: genetics and molecular biology of carotenoid pigment biosynthesis. FASEB Journal 10, 228-237.
| Crossref | Google Scholar | PubMed |
Avenson TJ, Cruz JA, Kanazawa A, Kramer DM (2005) Regulating the proton budget of higher plant photosynthesis. Proceedings of the National Academy of Sciences 102, 9709-9713.
| Crossref | Google Scholar |
Avonce N, Leyman B, Mascorro-Gallardo JO, Van Dijck P, Thevelein JM, Iturriaga G (2004) The Arabidopsis trehalose-6-P synthase AtTPS1 gene is a regulator of glucose, abscisic acid, and stress signaling. Plant Physiology 136, 3649-3659.
| Crossref | Google Scholar | PubMed |
Bello-Pérez LA, García-Suárez FJL, Agama-Acevedo E (2007) Mango carbohydrates. Food 1, 36-40.
| Google Scholar |
Berman ME, DeJong TM (2003) Seasonal patterns of vegetative growth and competition with reproductive sinks in peach (Prunus persica). The Journal of Horticultural Science and Biotechnology 78, 303-309.
| Crossref | Google Scholar |
Bihmidine S, Hunter CT, III, Johns CE, Koch KE, Braun DM (2013) Regulation of assimilate import into sink organs: update on molecular drivers of sink strength. Frontiers in Plant Science 4, 177.
| Crossref | Google Scholar | PubMed |
Blanco A, Pequerul A, Val J, Monge E, Gomez Aparisi J (1995) Crop-load effects on vegetative growth, mineral nutrient concentration and leaf water potential in ‘Catherine’ peach. Journal of Horticultural Science 70, 623-629.
| Crossref | Google Scholar |
Blanke MM (2009) Regulatory mechanisms in source-sink relationships in plants – a review. Acta Horticulturae 835, 13-20.
| Crossref | Google Scholar |
Boldingh H, Smith GS, Klages K (2000) Seasonal concentrations of non-structural carbohydrates of five Actinidia species in fruit, leaf, and fine root tissue. Annals of Botany 85, 469-476.
| Crossref | Google Scholar |
Boyer JS (1988) Cell enlargement and growth-induced water potentials. Physiologia Plantarum 73, 311-316.
| Crossref | Google Scholar |
Breen K, Tustin S, Palmer J, Boldingh H, Close D (2020) Revisiting the role of carbohydrate reserves in fruit set and early-season growth of apple. Scientia Horticulturae 261, 109034.
| Crossref | Google Scholar |
Candolfi-Vasconcelos MC, Candolfi MP, Kohlet W (1994) Retranslocation of carbon reserves from the woody storage tissues into the fruit as a response to defoliation stress during the ripening period in Vitis vinifera L. Planta 192, 567-573.
| Crossref | Google Scholar |
Capelli M, Lauri PÉ, Normand F (2016) Deciphering the costs of reproduction in mango – vegetative growth matters. Frontiers in Plant Science 7, 1531.
| Crossref | Google Scholar | PubMed |
Capelli M, Lauri PÉ, Léchaudel M, Normand F (2021) Hormones and carbohydrates are both involved in the negative effects of reproduction on vegetative bud outgrowth in the mango tree: consequences for irregular bearing. Tree Physiology 41, 2293-2307.
| Crossref | Google Scholar | PubMed |
Chacko EK, Reddy YTN, Ananthanarayanan TV (1982) Studies on the relationship between leaf number and area and fruit development in mango (Mangifera indica L.). Journal of Horticultural Science 57, 483-492.
| Crossref | Google Scholar |
Chauhan PS, Pandey RM (1984) Relative 14CO2 fixation by leaves and fruits, and translocation of 14C-sucrose in mango. Scientia Horticulturae 22, 121-128.
| Crossref | Google Scholar |
Chen Y, Grimplet J, David K, Castellarin SD, Terol J, Wong DCJ, Luo Z, Schaffer R, Celton JM, Talon M, Gambetta GA, Chervin C (2018) Ethylene receptors and related proteins in climacteric and non-climacteric fruits. Plant Science 276, 63-72.
| Crossref | Google Scholar | PubMed |
Cherian S, Figueroa CR, Nair H (2014) ‘Movers and shakers’ in the regulation of fruit ripening: a cross-dissection of climacteric versus non-climacteric fruit. Journal of Experimental Botany 65, 4705-4722.
| Crossref | Google Scholar | PubMed |
Cheung AY, McNellis T, Piekos B (1993) Maintenance of chloroplast components during chromoplast differentiation in the tomato mutant green flesh. Plant Physiology 101, 1223-1229.
| Crossref | Google Scholar | PubMed |
Chidley HG, Deshpande AB, Oak PS, Pujari KH, Giri AP, Gupta VS (2017) Effect of postharvest ethylene treatment on sugar content, glycosidase activity and its gene expression in mango fruit. Journal of the Science of Food and Agriculture 97, 1624-1633.
| Crossref | Google Scholar | PubMed |
Chiou T-J, Bush DR (1998) Sucrose is a signal molecule in assimilate partitioning. Proceedings of the National Academy of Sciences 95, 4784-4788.
| Crossref | Google Scholar |
Ciereszko I (2018) Regulatory roles of sugars in plant growth and development. Acta Societatis Botanicorum Poloniae 87, 3583.
| Crossref | Google Scholar |
Corbesier L, Bernier G, Périlleux C (2002) C:N ratio increases in the phloem sap during floral transition of the long-day plants Sinapis alba and Arabidopsis thaliana. Plant and Cell Physiology 43, 684-688.
| Crossref | Google Scholar | PubMed |
Cronje RB, Hajari E, Jonker A, Ratlapane IM, Huang X, Theron KI, Hoffman EW (2022) Foliar application of ethephon induces bud dormancy and affects gene expression of dormancy- and flowering-related genes in ‘Mauritius’ litchi (Litchi chinensis Sonn.). Journal of Plant Physiology 276, 153768.
| Crossref | Google Scholar | PubMed |
Das PK, Shin DH, Choi SB, Park YI (2012) Sugar-hormone cross-talk in anthocyanin biosynthesis. Molecules and Cells 34, 501-508.
| Crossref | Google Scholar | PubMed |
Das A, Geetha GA, Ravishankar KV, Shivashankara KS, Roy TK, Dinesh MR (2019) Interrelations of growth regulators, carbohydrates and expression of flowering genes (FT, LFY, AP1) in leaf and shoot apex of regular and alternate bearing mango (Mangifera indica L.) cultivars during flowering. Scientia Horticulturae 253, 263-269.
| Crossref | Google Scholar |
Davenport TL (2007) Reproductive physiology of mango. Brazilian Journal of Plant Physiology 19, 363-376.
| Crossref | Google Scholar |
Davie SJ, Stassen PJC (1997a) The effect of fruit thinning and tree pruning on tree starch reserves and on fruit retention of “Sensation” mango trees. Acta Horticulturae 455, 160-166.
| Crossref | Google Scholar |
Davie SJ, Stassen PJC (1997b) Mango model: Starch distribution in different tissues of “Sensation” mango trees of varying ages. Acta Horticulturae 455, 143-150.
| Crossref | Google Scholar |
Davie SJ, Stassen PJC, Grove HG (1999) Starch reserves in the mango tree. Acta Horticulturae 509, 335-346.
| Google Scholar |
DeJong TM (1986) Fruit effects on photosynthesis in Prunus persica. Physiologia Plantarum 66, 149-153.
| Crossref | Google Scholar |
Desta B, Amare G (2021) Paclobutrazol as a plant growth regulator. Chemical and Biological Technologies in Agriculture 8, 1.
| Crossref | Google Scholar |
Devoghalaere F, Doucen T, Guitton B, Keeling J, Payne W, Ling TJ, Ross JJ, Hallett IC, Gunaseelan K, Dayatilake GA, Diak R, Breen KC, Tustin DS, Costes E, Chagné D, Schaffer RJ, David KM (2012) A genomics approach to understanding the role of auxin in apple (Malus x domestica) fruit size control. BMC Plant Biology 12, 7.
| Crossref | Google Scholar | PubMed |
Dominguez PG, Niittylä T (2022) Mobile forms of carbon in trees: metabolism and transport. Tree Physiology 42, 458-487.
| Crossref | Google Scholar |
Durán-Soria S, Pott DM, Osorio S, Vallarino JG (2020) Sugar signaling during fruit ripening. Frontiers in Plant Science 11, 564917.
| Crossref | Google Scholar | PubMed |
Durán-Zuazo V, Aguilar-Ruiz J, Martínez-Raya A (2005) Fruit yield, plant growth and nutrient status in mango: effect of rootstocks. International Journal of Fruit Science 5, 3-21.
| Crossref | Google Scholar |
El-Sharkawy I, Sherif S, Abdulla M, Jayasankar S (2017) Plum fruit development occurs via gibberellin–sensitive and –insensitive DELLA repressors. PLoS ONE 12, e0169440.
| Crossref | Google Scholar | PubMed |
Erf JA, Proctor JTA (1987) Changes in apple leaf water status and vegetative growth influenced by crop load. Journal of the American Society for Horticultural Science 112, 617-620.
| Crossref | Google Scholar |
Evans JR (1989) Photosynthesis and nitrogen relationships in leaves of C₃ plants. Oecologia 78, 9-19.
| Crossref | Google Scholar | PubMed |
Eveland AL, Jackson DP (2012) Sugars, signalling, and plant development. Journal of Experimental Botany 63, 3367-3377.
| Crossref | Google Scholar | PubMed |
Fabre D, Dingkuhn M, Yin X, Clément-Vidal A, Roques S, Soutiras A, Luquet D (2020) Genotypic variation in source and sink traits affects the response of photosynthesis and growth to elevated atmospheric CO2. Plant, Cell & Environment 43, 579-593.
| Crossref | Google Scholar | PubMed |
Fichtner F, Lunn JE (2021) The role of trehalose 6-phosphate (Tre6P) in plant metabolism and development. Annual Review of Plant Biology 72, 737-760.
| Crossref | Google Scholar | PubMed |
Figueroa CM, Lunn JE (2016) A tale of two sugars: trehalose 6-phosphate and sucrose. Plant Physiology 172, 7-27.
| Crossref | Google Scholar | PubMed |
Fischer G, Almanza-Merchán PJ, Ramírez F (2013) Source-sink relationships in fruit species: a review. Revista Colombiana de Ciencias Hortícolas 6, 238-253.
| Google Scholar |
Génard M, Dauzat J, Franck N, Lescourret F, Moitrier N, Vaast P, Vercambre G (2008) Carbon allocation in fruit trees: from theory to modelling. Trees 22, 269-282.
| Crossref | Google Scholar |
Georgelis N, Fencil K, Richael CM (2018) Validation of a rapid and sensitive HPLC/MS method for measuring sucrose, fructose and glucose in plant tissues. Food Chemistry 262, 191-198.
| Crossref | Google Scholar | PubMed |
Gibson SI (2005) Control of plant development and gene expression by sugar signaling. Current Opinion in Plant Biology 8, 93-102.
| Crossref | Google Scholar | PubMed |
Goldschmidt EE, Huber SC (1992) Regulation of photosynthesis by end-product accumulation in leaves of plants storing starch, sucrose, and hexose sugars. Plant Physiology 99, 1443-1448.
| Crossref | Google Scholar | PubMed |
Gómez-Cadenas A, Mehouachi J, Tadeo FR, Primo-Millo E, Talon M (2000) Hormonal regulation of fruitlet abscission induced by carbohydrate shortage in citrus. Planta 210, 636-643.
| Crossref | Google Scholar | PubMed |
Grechi I, Normand F (2019) Effect of source-sink relationships from the branch to the tree scale on mango fruit size and quality at harvest. Acta Horticulturae 1244, 93-100.
| Crossref | Google Scholar |
Gupta K, Wani SH, Razzaq A, Skalicky M, Samantara K, Gupta S, Pandita D, Goel S, Grewal S, Hejnak V, Shiv A, El-Sabrout AM, Elansary HO, Alaklabi A, Brestic M (2022) Abscisic acid: role in fruit development and ripening. Frontiers in Plant Science 13, 817500.
| Crossref | Google Scholar | PubMed |
Hagemann MH, Winterhagen P, Hegele M, Wünsche JN (2015) Ethephon induced abscission in mango: physiological fruitlet responses. Frontiers in Plant Science 6, 706.
| Crossref | Google Scholar | PubMed |
Han Q, Kagawa A, Kabeya D, Inagaki Y (2016) Reproduction-related variation in carbon allocation to woody tissues in Fagus crenata using a natural 13C approach. Tree Physiology 36, 1343-1352.
| Google Scholar | PubMed |
Hartmann H, Trumbore S (2016) Understanding the roles of nonstructural carbohydrates in forest trees – from what we can measure to what we want to know. New Phytologist 211, 386-403.
| Crossref | Google Scholar | PubMed |
He H, Yamamuro C (2022) Interplays between auxin and GA signaling coordinate early fruit development. Horticulture Research 9, uhab078.
| Crossref | Google Scholar |
Ho LC (1976) The relationship between the rates of carbon transport and of photosynthesis in tomato leaves. Journal of Experimental Botany 27, 87-97.
| Crossref | Google Scholar |
Iglesias DJ, Tadeo FR, Legaz F, Primo-Millo E, Talon M (2001) In vivo sucrose stimulation of colour change in citrus fruit epicarps: interactions between nutritional and hormonal signals. Physiologia Plantarum 112, 244-250.
| Crossref | Google Scholar | PubMed |
Iturriaga G, Suárez R, Nova-Franco B (2009) Trehalose metabolism: from osmoprotection to signaling. International Journal of Molecular Sciences 10, 3793-3810.
| Crossref | Google Scholar | PubMed |
Jannoyer M, Lauri P-E (2006) Removal of mango inflorescence increases fruit set and does not affect yield (Mangifera indica cv. Cogshall). Acta Horticulturae 820, 433-436.
| Google Scholar |
Jia H, Wang Y, Sun M, Li B, Han Y, Zhao Y, Li X, Ding N, Li C, Ji W, Jia W (2013) Sucrose functions as a signal involved in the regulation of strawberry fruit development and ripening. New Phytologist 198, 453-465.
| Crossref | Google Scholar | PubMed |
Kaiser E, Morales A, Harbinson J, Kromdijk J, Heuvelink E, Marcelis LFM (2015) Dynamic photosynthesis in different environmental conditions. Journal of Experimental Botany 66, 2415-2426.
| Crossref | Google Scholar | PubMed |
Karim A, Koeda K, Nii N (1999) Changes in anatomical features, pigment content and photosynthetic activity related to age of ‘Irwin’ mango leaves. Journal of the Japanese Society for Horticultural Science 68, 1090-1098.
| Crossref | Google Scholar |
Kaur H, Sidhu GS, Mittal A, Yadav IS, Mittal M, Singla D, Singh N, Chhuneja P (2022) Comparative transcriptomics in alternate bearing cultivar Dashehari reveals the genetic model of flowering in mango. Fontiers in Genetics 13, 1061168.
| Crossref | Google Scholar |
Kim J (2019) Sugar metabolism as input signals and fuel for leaf senescence. Genes & Genomics 41, 737-746.
| Crossref | Google Scholar | PubMed |
Kliewer WM, Dokoozlian NK (2005) Leaf area/crop weight ratios of grapevines: influence on fruit composition and wine quality. American Journal of Enology and Viticulture 56, 170-181.
| Crossref | Google Scholar |
Koch K (2004) Sucrose metabolism: regulatory mechanisms and pivotal roles in sugar sensing and plant development. Current Opinion in Plant Biology 7, 235-246.
| Crossref | Google Scholar | PubMed |
Kötting O, Kossmann J, Zeeman SC, Lloyd JR (2010) Regulation of starch metabolism: the age of enlightenment? Current Opinion in Plant Biology 13, 320-328.
| Crossref | Google Scholar |
Kozlowski TT (1992) Carbohydrate sources and sinks in woody plants. The Botanical Review 58, 107-222.
| Crossref | Google Scholar |
Kuang J-F, Wu J-Y, Zhong H-Y, Li C-Q, Chen J-Y, Lu W-J, Li J-G (2012) Carbohydrate stress affecting fruitlet abscission and expression of genes related to auxin signal transduction pathway in litchi. International Journal of Molecular Sciences 13, 16084-16103.
| Crossref | Google Scholar | PubMed |
Kumar R, Khurana A, Sharma AK (2014) Role of plant hormones and their interplay in development and ripening of fleshy fruits. Journal of Experimental Botany 65, 4561-4575.
| Crossref | Google Scholar | PubMed |
Lalonde S, Boles E, Hellmann H, Barker L, Patrick JW, Frommer WB, Ward JM (1999) The dual function of sugar carriers: transport and sugar sensing. The Plant Cell 11, 707-726.
| Crossref | Google Scholar | PubMed |
Lanoue J, Leonardos ED, Grodzinski B (2018) Effects of light quality and intensity on diurnal patterns and rates of photo-assimilate translocation and transpiration in tomato leaves. Frontiers in Plant Science 9, 756.
| Crossref | Google Scholar | PubMed |
Lauri P-É, Normand F (2017) Are leaves only involved in flowering? Bridging the gap between structural botany and functional morphology. Tree Physiology 37, 1137-1139.
| Crossref | Google Scholar |
Lechaudel M, Genard M, Lescourret F, Urban L, Jannoyer M (2002) Leaf-to-fruit ratio affects water and dry-matter content of mango fruit. The Journal of Horticultural Science and Biotechnology 77, 773-777.
| Crossref | Google Scholar |
Léchaudel M, Joas J (2006) Quality and maturation of mango fruits of cv. Cogshall in relation to harvest date and carbon supply. Australian Journal of Agricultural Research 57, 419-426.
| Crossref | Google Scholar |
Léchaudel M, Joas J (2007) An overview of preharvest factors influencing mango fruit growth, quality and postharvest behaviour. Brazilian Journal of Plant Physiology 19, 287-298.
| Crossref | Google Scholar |
Léchaudel M, Génard M, Lescourret F, Urban L, Jannoyer M (2005a) Modeling effects of weather and source–sink relationships on mango fruit growth. Tree Physiology 25, 583-597.
| Google Scholar | PubMed |
Léchaudel M, Joas J, Caro Y, Genard M, Jannoyer M (2005b) Leaf:fruit ratio and irrigation supply affect seasonal changes in minerals, organic acids and sugars of mango fruit. Journal of the Science of Food and Agriculture 85, 251-260.
| Crossref | Google Scholar |
Lee B-R, Cho J-H, Wi SG, Yang U, Jung W-J, Lee S-H (2021) The sucrose-to-hexose ratio is a significant determinant for fruit maturity and is modulated by invertase and sucrose re-synthesis during fruit development and ripening in Asian pear (Pyrus pyrifolia Nakai) cultivars. Horticultural Science and Technology 39, 141-151.
| Crossref | Google Scholar |
León P, Sheen J (2003) Sugar and hormone connections. Trends in Plant Science 8, 110-116.
| Crossref | Google Scholar | PubMed |
Li L, Yuan H (2013) Chromoplast biogenesis and carotenoid accumulation. Archives of Biochemistry and Biophysics 539, 102-109.
| Crossref | Google Scholar | PubMed |
Li L, Wu H-X, Ma X-W, Xu W-T, Liang Q-Z, Zhan R-L, Wang S-B (2020) Transcriptional mechanism of differential sugar accumulation in pulp of two contrasting mango (Mangifera indica L.) cultivars. Genomics 112, 4505-4515.
| Crossref | Google Scholar | PubMed |
Liang Q, Song K, Lu M, Dai T, Yang J, Wan J, Li L, Chen J, Zhan R, Wang S (2022) Transcriptome and metabolome analyses reveal the involvement of multiple pathways in flowering intensity in mango. Frontiers in Plant Science 13, 933923.
| Crossref | Google Scholar | PubMed |
Lin Z, Zhong S, Grierson D (2009) Recent advances in ethylene research. Journal of Experimental Botany 60, 3311-3336.
| Crossref | Google Scholar | PubMed |
Ljung K, Nemhauser JL, Perata P (2015) New mechanistic links between sugar and hormone signalling networks. Current Opinion in Plant Biology 25, 130-137.
| Crossref | Google Scholar | PubMed |
Lloyd JR, Kossmann J (2019) Starch trek: the search for yield. Frontiers in Plant Science 9, 1930.
| Crossref | Google Scholar |
Lloyd JC, Zakhleniuk OV (2004) Responses of primary and secondary metabolism to sugar accumulation revealed by microarray expression analysis of the Arabidopsis mutant, pho3. Journal of Experimental Botany 55, 1221-1230.
| Crossref | Google Scholar | PubMed |
Lobo AKM, de Oliveira Martins M, Neto MCL, Machado EC, Ribeiro RV, Silveira JAG (2015) Exogenous sucrose supply changes sugar metabolism and reduces photosynthesis of sugarcane through the down-regulation of Rubisco abundance and activity. Journal of Plant Physiology 179, 113-121.
| Crossref | Google Scholar | PubMed |
Loescher WH, McCamant T, Keller JD (1990) Carbohydrate reserves, translocation, and storage in woody plant roots. HortScience 25, 274-281.
| Crossref | Google Scholar |
Lu P, Chacko EK, Bithell SL, Schaper H, Wiebel J, Cole S, Müller WJ (2012) Photosynthesis and stomatal conductance of five mango cultivars in the seasonally wet–dry tropics of northern Australia. Scientia Horticulturae 138, 108-119.
| Crossref | Google Scholar |
Lueangprasert K, Uthaibutra J, Saengnil K, Arakawa O (2010) The effects of sugar application on the concentrations of anthocyanin and flavonol of ‘Mahajanaka’ mango (Mangifera indica Linn. cv. Mahajanaka) fruit. Chiang Mai Journal of Science 37, 355-362.
| Google Scholar |
Lunn JE, Delorge I, Figueroa CM, Van Dijck P, Stitt M (2014) Trehalose metabolism in plants. The Plant Journal 79, 544-567.
| Crossref | Google Scholar | PubMed |
Luo Y, Ge C, Ling Y, Mo F, Yang M, Jiang L, Chen Q, Lin Y, Sun B, Zhang Y, Wang Y, Li M, Wang X, Tang H (2020) ABA and sucrose co-regulate strawberry fruit ripening and show inhibition of glycolysis. Molecular Genetics and Genomics 295, 421-438.
| Crossref | Google Scholar | PubMed |
Ma C, Sun Z, Chen C, Zhang L, Zhu S (2014) Simultaneous separation and determination of fructose, sorbitol, glucose and sucrose in fruits by HPLC–ELSD. Food Chemistry 145, 784-788.
| Crossref | Google Scholar | PubMed |
Ma Q-J, Sun M-H, Lu J, Liu Y-J, Hu D-G, Hao Y-J (2017) Transcription Factor AREB2 is involved in soluble sugar accumulation by activating sugar transporter and amylase genes. Plant Physiology 174, 2348-2362.
| Crossref | Google Scholar | PubMed |
MacNeill GJ, Mehrpouyan S, Minow MA, Patterson JA, Tetlow IJ, Emes MJ, Raines C (2017) Starch as a source, starch as a sink: the bifunctional role of starch in carbon allocation. Journal of Experimental Botany 68, 4433-4453.
| Crossref | Google Scholar | PubMed |
Malundo TMM, Shewfelt RL, Ware GO, Baldwin EA (2001) Sugars and acids influence flavor properties of mango (Mangifera indica). Journal of the American Society for Horticultural Science 126, 115-121.
| Crossref | Google Scholar |
Marcelis LFM (1996) Sink strength as a determinant of dry matter partitioning in the whole plant. Journal of Experimental Botany 47, 1281-1291.
| Crossref | Google Scholar | PubMed |
Medlicott AP, Thompson AK (1985) Analysis of sugars and organic acids in ripening mango fruits (Mangifera indica L. var Keitt) by high performance liquid chromatography. Journal of the Science of Food and Agriculture 36, 561-566.
| Crossref | Google Scholar |
Medlicott AP, Bhogal M, Reynolds SB (1986) Changes in peel pigmentation during ripening of mango fruit (Mangifera indica var. Tommy Atkins). Annals of Applied Biology 109, 651-656.
| Crossref | Google Scholar |
Menzel CM, Le Lagadec MD (2017) Can the productivity of mango orchards be increased by using high-density plantings? Scientia Horticulturae 219, 222-263.
| Crossref | Google Scholar |
Mesa K, Serra S, Masia A, Gagliardi F, Bucci D, Musacchi S (2019) Preliminary study on effect of early defoliation on dry matter accumulation and storage of reserves on ‘Abbé Fétel’ pear trees. HortScience 54, 2169-2177.
| Crossref | Google Scholar |
Monselise S, Goldschmidt E (1982) Alternate bearing in fruit trees. Horticultural Reviews 4, 128-173.
| Google Scholar |
Morinaga K, Imai S, Yakushiji H, Koshita Y (2003) Effects of fruit load on partitioning of 15N and 13C, respiration, and growth of grapevine roots at different fruit stages. Scientia Horticulturae 97, 239-253.
| Crossref | Google Scholar |
Morris DA, Arthur ED (1984) Invertase and auxin-induced elongation in internodal segments of Phaseolus vulgaris. Phytochemistry 23, 2163-2167.
| Crossref | Google Scholar |
Nardozza S, Boldingh HL, Kashuba MP, Feil R, Jones D, Thrimawithana AH, Ireland HS, Philippe M, Wohlers MW, McGhie TK, Montefiori M, Lunn JE, Allan AC, Richardson AC (2020) Carbon starvation reduces carbohydrate and anthocyanin accumulation in red-fleshed fruit via trehalose 6-phosphate and MYB27. Plant, Cell & Environment 43, 819-835.
| Crossref | Google Scholar | PubMed |
Ni J, Liao Y, Zhang M, Pan C, Yang Q, Bai S, Teng Y (2022) Blue light simultaneously induces peel anthocyanin biosynthesis and flesh carotenoid/sucrose biosynthesis in mango fruit. Journal of Agricultural and Food Chemistry 70, 16021-16035.
| Crossref | Google Scholar | PubMed |
Noiraud N, Maurousset L, Lemoine R (2001) Transport of polyols in higher plants. Plant Physiology and Biochemistry 39, 717-728.
| Crossref | Google Scholar |
Normand F, Jannoyer M, Barantin P, Damour G, Dechazal M, Mialet-Serra I, Clément A, Verdeil JL, Escoute J, Sonderegger N (2006) Nature, location and seasonal changes of non-structural carbohydrates in mango. In ‘Carbon storage in coconut, oil palm, rubber and mango: origins, dynamics and consequences for plantation management’. (Ed. CIRAD) pp 25–38. (CIRAD: Montpellier, France)
Normand F, Lagier S, Escoutes J, Verdeil JL, Mialet-Serra I (2009) Starch localisation in mango tree: histological observations. Acta Horticulturae 820, 245-250.
| Crossref | Google Scholar |
Normand F, Boudon F, Capelli M, Dambreville A, Lauri PÉ (2017) Linking vegetative growth, reproduction and irregular bearing in the mango tree. Acta Horticulturae 1229, 341-348.
| Crossref | Google Scholar |
Olesen T, Robertson D, Muldoon S, Meyer R (2008) The role of carbohydrate reserves in evergreen tree development, with particular reference to macadamia. Scientia Horticulturae 117, 73-77.
| Crossref | Google Scholar |
Pandey R (1974) Biochemical changes in the developing mango fruit (Mangifera indica L.) cv. Dashehari. Progressive Horticulture 5, 47-59.
| Google Scholar |
Pandey R (1989) Physiology of flowering in mango. Acta Horticulturae 231, 361-380.
| Google Scholar |
Patrick JW (1988) Assimilate partitioning in relation to crop productivity. HortScience 23, 33-40.
| Crossref | Google Scholar |
Paul MJ, Pellny TK (2003) Carbon metabolite feedback regulation of lead photosynthesis and development. Journal of Experimental Botany 54, 539-547.
| Crossref | Google Scholar | PubMed |
Pawar R, Rana VS (2019) Manipulation of source-sink relationship in pertinence to better fruit quality and yield in fruit crops: a review. Agricultural Reviews 40, 200-207.
| Crossref | Google Scholar |
Pfister B, Zeeman SC (2016) Formation of starch in plant cells. Cellular and Molecular Life Sciences 73, 2781-2807.
| Crossref | Google Scholar | PubMed |
Pongsomboon W, Subhadrabandhu S, Stephenson RA (1997) Some aspects of the ecophysiology of flowering intensity of mango (Mangifera indica L.) cv. Nam Dok Mai in a semi-tropical monsoon Asian climate. Scientia Horticulturae 70, 45-56.
| Crossref | Google Scholar |
Prasad SRS, Reddy YTN, Upreti KK, Rajeshwara AN (2014) Studies on changes in carbohydrate metabolism in regular bearing and “off” season bearing cultivars of mango (Mangifera indica L.) during flowering. International Journal of Fruit Science 14, 437-459.
| Crossref | Google Scholar |
Priestly CA (1962) Carbohydrate resources within the perennial plant. Soil Science 94, 198.
| Crossref | Google Scholar |
Rahim AA, Elaminand O, Bangerth FK (2011) Effects of paclobutrazol (PBZ) on floral induction and associated hormonal and metabolic changes of biennially bearing mango (Mangifera indica L.) cultivars during off year. Journal of Agricultural and Biological Science 6, 55-67.
| Google Scholar |
Ranganath KG, Shivashankara KS, Roy TK, Dinesh MR, Geetha GA, Pavithra KCG, Ravishankar KV (2018) Profiling of anthocyanins and carotenoids in fruit peel of different colored mango cultivars. Journal of Food Science and Technology 55, 4566-4577.
| Crossref | Google Scholar | PubMed |
Ravindra MR, Goswami TK (2008) Modelling the respiration rate of green mature mango under aerobic conditions. Biosystems Engineering 99, 239-248.
| Crossref | Google Scholar |
Reddy YTN, Singh G (1991) Further studies on the relationship between leaf number and area and fruit development in mango (Mangifera indica L.). Journal of Horticultural Science 66, 471-478.
| Crossref | Google Scholar |
Rolland F, Baena-Gonzalez E, Sheen J (2006) Sugar sensing and signaling in plants: conserved and novel mechanisms. Annual Review of Plant Biology 57, 675-709.
| Crossref | Google Scholar | PubMed |
Rontein D, Basset G, Hanson AD (2002) Metabolic engineering of osmoprotectant accumulation in plants. Metabolic Engineering 4, 49-56.
| Crossref | Google Scholar | PubMed |
Roper TR, Loescher WH (1987) Relationships between leaf area per fruit and fruit quality in ‘Bing’ sweet cherry. HortScience 22, 1273-1276.
| Crossref | Google Scholar |
Rossouw GC, Orchard BA, Šuklje K, Smith JP, Barril C, Deloire A, Holzapfel BP (2017a) Vitis vinifera root and leaf metabolic composition during fruit maturation: implications of defoliation. Physiologia Plantarum 161, 434-450.
| Crossref | Google Scholar | PubMed |
Rossouw GC, Smith JP, Barril C, Deloire A, Holzapfel BP (2017b) Carbohydrate distribution during berry ripening of potted grapevines: impact of water availability and leaf-to-fruit ratio. Scientia Horticulturae 216, 215-225.
| Crossref | Google Scholar |
Ruan Y-L (2012) Signaling role of sucrose metabolism in development. Molecular Plant 5, 763-765.
| Crossref | Google Scholar | PubMed |
Ruan Y-L, Patrick JW, Bouzayen M, Osorio S, Fernie AR (2012) Molecular regulation of seed and fruit set. Trends in Plant Science 17, 656-665.
| Crossref | Google Scholar | PubMed |
Sadali NM, Sowden RG, Ling Q, Jarvis RP (2019) Differentiation of chromoplasts and other plastids in plants. Plant Cell Reports 38, 803-818.
| Crossref | Google Scholar | PubMed |
Sakhidin , Purwoko BS, Teixeira da Silva JA, Poerwanto R, Soesanto S (2011) Indole-3-acetic acid, 1-amino cyclopropane-1-carboxylic acid, and carbohydrate in relation to fruit drop on mango tree. Journal of Fruit and Ornamental Plant Research 19, 41-49.
| Google Scholar |
Sami F, Yusuf M, Faizan M, Faraz A, Hayat S (2016) Role of sugars under abiotic stress. Plant Physiology and Biochemistry 109, 54-61.
| Crossref | Google Scholar | PubMed |
Sawicki M, Aït Barka E, Clément C, Vaillant-Gaveau N, Jacquard C (2015) Cross-talk between environmental stresses and plant metabolism during reproductive organ abscission. Journal of Experimental Botany 66, 1707-1719.
| Crossref | Google Scholar | PubMed |
Shalom L, Samuels S, Zur N, Shlizerman L, Zemach H, Weissberg M, Ophir R, Blumwald E, Sadka A (2012) Alternate bearing in citrus: changes in the expression of flowering control genes and in global gene expression in ON- versus OFF-crop trees. PLoS One 7, e46930.
| Crossref | Google Scholar | PubMed |
Sharkey TD (2019) Is triose phosphate utilization important for understanding photosynthesis? Journal of Experimental Botany 70, 5521-5525.
| Crossref | Google Scholar | PubMed |
Sharma N, Singh SK, Mahato AK, Ravishankar H, Dubey AK, Singh NK (2019) Physiological and molecular basis of alternate bearing in perennial fruit crops. Scientia Horticulturae 243, 214-225.
| Crossref | Google Scholar |
Sharma N, Singh AK, Singh SK, Mahato AK, Srivastav M, Singh NK (2020) Comparative RNA sequencing based transcriptome profiling of regular bearing and alternate bearing mango (Mangifera indica L.) varieties reveals novel insights into the regulatory mechanisms underlying alternate bearing. Biotechnology Letters 42, 1035-1050.
| Crossref | Google Scholar | PubMed |
Shivashankar S, Sumathi M (2019) Rapid burst of ethylene evolution by premature seed: a warning sign for the onset of spongy tissue disorder in Alphonso mango fruit? Journal of Biosciences 44, 133.
| Crossref | Google Scholar | PubMed |
Shivashankara KS, Mathai CK (2000) Inhibition of photosynthesis by flowering in mango (Mangifera indica L.). A study by gas exchange methods. Scientia Horticulturae 83, 205-212.
| Crossref | Google Scholar |
Simão RA, Silva APFB, Peroni FHG, do Nascimento JRO, Louro RP, Lajolo FM, Cordenunsi BR (2008) Mango starch degradation. I. A microscopic view of the granule during ripening. Journal of Agricultural and Food Chemistry 56, 7410-7415.
| Crossref | Google Scholar | PubMed |
Simmons SL, Hofman PJ, Whiley AW, Hetherington SE (1998) Effects of leaf: fruit ratios on fruit growth, mineral concentration and quality of mango (Mangifera indica L. cv. Kensington Pride). The Journal of Horticultural Science and Biotechnology 73, 367-374.
| Crossref | Google Scholar |
Singh SK, Nath V, Marboh E, Sharma S (2017) Source-sink relationship in litchi verses mango: a concept. International Journal of Current Microbiology and Applied Sciences 6, 500-509.
| Crossref | Google Scholar |
Smeekens S, Ma J, Hanson J, Rolland F (2010) Sugar signals and molecular networks controlling plant growth. Current Opinion in Plant Biology 13, 273-278.
| Crossref | Google Scholar |
Smith JP, Holzapfel BP (2009) Cumulative responses of Semillon grapevines to late season perturbation of carbohydrate reserve status. American Journal of Enology and Viticulture 60, 461-470.
| Crossref | Google Scholar |
Smith HM, Samach A (2013) Constraints to obtaining consistent annual yields in perennial tree crops. I: heavy fruit load dominates over vegetative growth. Plant Science 207, 158-167.
| Crossref | Google Scholar | PubMed |
Smith AM, Stitt M (2007) Coordination of carbon supply and plant growth. Plant, Cell & Environment 30, 1126-1149.
| Crossref | Google Scholar | PubMed |
Smith AM, Zeeman SC (2006) Quantification of starch in plant tissues. Nature Protocols 1, 1342-1345.
| Crossref | Google Scholar | PubMed |
Smith AM, Zeeman SC, Smith SM (2005) Starch degradation. Annual Review of Plant Biology 56, 73-98.
| Crossref | Google Scholar | PubMed |
Smith NG, Keenan TF, Prentice IC, Wang H, Wright IJ, Niinemets Ü, Crous KY, Domingues TF, Guerrieri R, Ishida FY, Kattge J, Kruger EL, Maire V, Rogers A, Serbin SP, Tarvainen L, Togashi HF, Townsend PA, Wang M, Weerasinghe LK, Zhou SX (2019) Global photosynthetic capacity is optimized to the environment. Ecology Letters 22, 506-517.
| Crossref | Google Scholar | PubMed |
Sonnewald U, Fernie AR (2018) Next-generation strategies for understanding and influencing source–sink relations in crop plants. Current Opinion in Plant Biology 43, 63-70.
| Crossref | Google Scholar | PubMed |
Sprugel DG, Hinckley TM, Schaap W (1991) The theory and practice of branch autonomy. Annual Review of Ecology and Systematics 22, 309-334.
| Crossref | Google Scholar |
Stassen PJC, Janse van Vuuren BPH (1997) Storage, redistribution, and utilization of starch in young bearing “Sensation” mango trees. Acta Horticulturae 455, 151-159.
| Crossref | Google Scholar |
Stassen PJC, Grove HG, Davie SJ (2000) Uptake, distribution and requirements of macro elements in ‘Sensation’ mango trees. Acta Horticulturae 509, 365-374.
| Crossref | Google Scholar |
Stitt M, Zeeman SC (2012) Starch turnover: pathways, regulation and role in growth. Current Opinion in Plant Biology 15, 282-292.
| Crossref | Google Scholar | PubMed |
Sturm A, Tang G-Q (1999) The sucrose-cleaving enzymes of plants are crucial for development, growth and carbon partitioning. Trends in Plant Science 4, 401-407.
| Crossref | Google Scholar | PubMed |
Suryanarayana V (1978) Seasonal changes in sugars, starch, nitrogen and C:N ratio in relation to flowering in mango. Plant Biochemical Journal 5, 108-117.
| Google Scholar |
Tandon DK, Kalra SK (1983) Changes in sugars, starch and amylase activity during development of mango fruit cv. Dashehari. Journal of Horticultural Science 58, 449-453.
| Crossref | Google Scholar |
Tcherkez G, Limami AM (2019) Net photosynthetic CO2 assimilation: more than just CO2 and O2 reduction cycles. New Phytologist 223, 520-529.
| Crossref | Google Scholar | PubMed |
Télef N, Stammitti-Bert L, Mortain-Bertrand A, Maucourt M, Carde JP, Rolin D, Gallusci P (2006) Sucrose deficiency delays lycopene accumulation in tomato fruit pericarp discs. Plant Molecular Biology 62, 453-469.
| Crossref | Google Scholar | PubMed |
Tharanathan RN, Yashoda HM, Prabha TN (2006) Mango (Mangifera indica L.), “The king of fruits” – an overview. Food Reviews International 22, 95-123.
| Crossref | Google Scholar |
Tixier A, Guzmán-Delgado P, Sperling O, Amico Roxas A, Laca E, Zwieniecki MA (2020) Comparison of phenological traits, growth patterns, and seasonal dynamics of non-structural carbohydrate in Mediterranean tree crop species. Scientific Reports 10, 347.
| Crossref | Google Scholar | PubMed |
Tohge T, Watanabe M, Hoefgen R, Fernie A (2013) Shikimate and phenylalanine biosynthesis in the green lineage. Frontiers in Plant Science 4, 62.
| Crossref | Google Scholar | PubMed |
Upreti KK, Reddy YTN, Prasad SRS, Bindu GV, Jayaram HL, Rajan S (2013) Hormonal changes in response to paclobutrazol induced early flowering in mango cv. Totapuri. Scientia Horticulturae 150, 414-418.
| Crossref | Google Scholar |
Urban L, Léchaudel M (2005) Effect of leaf-to-fruit ratio on leaf nitrogen content and net photosynthesis in girdled branches of Mangifera indica L. Trees 19, 564-571.
| Crossref | Google Scholar |
Urban L, Bertheuil F, Lechaudel M (2002) A coupled photosynthesis and stomatal conductance model for mango leaves. Acta Horticulturae 584, 81-88.
| Crossref | Google Scholar |
Urban L, Le Roux X, Sinoquet H, Jaffuel S, Jannoyer M (2003) A biochemical model of photosynthesis for mango leaves: evidence for the effect of fruit on photosynthetic capacity of nearby leaves. Tree Physiology 23, 289-300.
| Crossref | Google Scholar | PubMed |
Urban L, Léchaudel M, Lu P (2004a) Effect of fruit load and girdling on leaf photosynthesis in Mangifera indica L. Journal of Experimental Botany 55, 2075-2085.
| Crossref | Google Scholar | PubMed |
Urban L, Lu P, Thibaud R (2004b) Inhibitory effect of flowering and early fruit growth on leaf photosynthesis in mango. Tree Physiology 24, 387-399.
| Crossref | Google Scholar | PubMed |
Urban L, Montpied P, Normand F (2006) Season effects on leaf nitrogen partitioning and photosynthetic water use efficiency in mango. Journal of Plant Physiology 163, 48-57.
| Crossref | Google Scholar | PubMed |
Urban L, Jegouzo L, Damour G, Vandame M, François C (2008) Interpreting the decrease in leaf photosynthesis during flowering in mango. Tree Physiology 28, 1025-1036.
| Crossref | Google Scholar | PubMed |
Valluru R, Van den Ende W (2011) Myo-inositol and beyond – emerging networks under stress. Plant Science 181, 387-400.
| Crossref | Google Scholar | PubMed |
Vittal H, Sharma N, Dubey AK, Shivran M, Singh SK, Meena MC, Kumar N, Sharma N, Singh N, Pandey R, Bollinedi H, Singh BP, Sharma RM, Gururani M (2023) Rootstock-mediated carbohydrate metabolism, nutrient contents, and physiological modifications in regular and alternate mango (Mangifera indica L.) scion varieties. PLoS ONE 18, e0284910.
| Crossref | Google Scholar | PubMed |
Watson RL, Landsberg JJ, Thorpe MR (1978) Photosynthetic characteristics of the leaves of ‘Golden Delicious’ appletrees. Plant, Cell & Environment 1, 51-58.
| Crossref | Google Scholar |
Weise SE, van Wijk KJ, Sharkey TD (2011) The role of transitory starch in C3, CAM, and C4 metabolism and opportunities for engineering leaf starch accumulation. Journal of Experimental Botany 62, 3109-3118.
| Crossref | Google Scholar | PubMed |
Weyers JDB, Paterson NW (2001) Plant hormones and the control of physiological processes. New Phytologist 152, 375-407.
| Crossref | Google Scholar | PubMed |
Whiley AW, Rasmussen TS, Saranah JB, Wolstenholme BN (1989) Effect of temperature on growth, dry matter production and starch accumulation in ten mango (Mangifera indica L.) cultivars. Journal of Horticultural Science 64, 753-765.
| Crossref | Google Scholar |
Wu S, Wu D, Song J, Zhang Y, Tan Q, Yang T, Yang J, Wang S, Xu J, Xu W, Liu A (2022) Metabolomic and transcriptomic analyses reveal new insights into the role of abscisic acid in modulating mango fruit ripening. Horticulture Research 9, uhac102.
| Crossref | Google Scholar |
Xie RJ, Deng L, Jing L, He SL, Ma YT, Yi SL, Zheng YQ, Zheng L (2013) Recent advances in molecular events of fruit abscission. Biologia Plantarum 57, 201-209.
| Crossref | Google Scholar |
Yadav UP, Ivakov A, Feil R, Duan GY, Walther D, Giavalisco P, Piques M, Carillo P, Hubberten HM, Stitt M, Lunn JE (2014) The sucrose-trehalose 6-phosphate (Tre6P) nexus: specificity and mechanisms of sucrose signalling by Tre6P. Journal of Experimental Botany 65, 1051-1068.
| Crossref | Google Scholar | PubMed |
Yeshitela T, Robbertse PJ, Stassen PJC (2004) Paclobutrazol suppressed vegetative growth and improved yield as well as fruit quality of ‘Tommy Atkins’ mango (Mangifera indica) in Ethiopia. New Zealand Journal of Crop and Horticultural Science 32, 281-293.
| Crossref | Google Scholar |
Yu J, Tseng Y, Pham K, Liu M, Beckles DM (2022) Starch and sugars as determinants of postharvest shelf life and quality: some new and surprising roles. Current Opinion in Biotechnology 78, 102844.
| Crossref | Google Scholar | PubMed |
Zaharah SS, Singh Z, Symons GM, Reid JB (2013) Mode of action of abscisic acid in triggering ethylene biosynthesis and softening during ripening in mango fruit. Postharvest Biology and Technology 75, 37-44.
| Crossref | Google Scholar |
Zeeman SC, Smith SM, Smith AM (2007) The diurnal metabolism of leaf starch. Biochemical Journal 401, 13-28.
| Crossref | Google Scholar | PubMed |
Zhang Z, Deng Y, Song X, Miao M (2015) Trehalose-6-phosphate and SNF1-related protein kinase 1 are involved in the first-fruit inhibition of cucumber. Journal of Plant Physiology 177, 110-120.
| Crossref | Google Scholar | PubMed |
Zhou Z, Zhang Z, van der Putten PEL, Fabre D, Dingkuhn M, Struik PC, Yin X (2023) Triose phosphate utilization in leaves is modulated by wholeplant sink–source ratios and nitrogen budgets in rice. Journal of Experimental Botany 74, 6692-6707.
| Crossref | Google Scholar | PubMed |
Zude M, Luedders P (1997) Vegetative growth cycles and comparison of chlorophyll and phenol contents, gas exchange and water regime from young to old leaves in mango. Angewandte Botanik 71, 10-13.
| Google Scholar |