Flooding stress and responses to hypoxia in plants
Juan de la Cruz Jiménez




A
B
C
D
E
F
Abstract
In recent years, research on flooding stress and hypoxic responses in plants has gathered increasing attention due to climate change and the important role of O2 in metabolism and signalling. This Collection of Functional Plant Biology on ‘Flooding stress and responses to hypoxia in plants’ presents key contributions aimed at progressing our current understanding on how plants respond to low-O2 conditions, flooding stress and a combination of stresses commonly found in flooded areas. The Collection emphasises the characterisation of diverse plant responses across different developmental stages, from seed germination to fully developed plants, and under different water stress conditions ranging from waterlogging to complete submergence, or simply low-O2 conditions resulting from limited O2 diffusivity in bulky tissues. Additionally, this Collection highlights diverse approaches, including eco-physiological characterisation of plant responses, detailed descriptions of root anatomical characteristics and their surrounding microenvironments, evaluation of the seed microbiota under flooding stress, the modification of gene expression, and evaluations of diverse germplasm collections.
Keywords: apoplastic barriers, complete submergence, flooding tolerance, low oxygen, partial submergence, radial oxygen loss, saline flooding, underwater germination.
Introduction
Plant growth in extensive agricultural and natural ecosystems is frequently hampered by flooding or a combination of diverse stress conditions, including saline flooding. Flooding encompasses two different situations: (1) waterlogging where only the roots are affected; and (2) partial or complete submergence also affecting the shoots (Fig. 1). During flooding, the gas-filled porous spaces in the soil are replaced by water, creating a hypoxic environment that limits root respiration and plant growth. In addition to the stress resulting from a lack of O2, an excess of salts in saline floods can further impair plant growth in such conditions. The adverse consequences of flooding and low-O2 stress increase in severity, from soil waterlogging to partial or complete submerge of plants (Fig. 1). These conditions can affect all stages of the plant cycle, from seed germination underwater to vegetative growth or grain production. Floods may be temporary, resulting from heavy rains, or cyclic, occurring due to tidal movements in coastal areas. Additionally, extreme conditions can also occur where heavy rains are followed by prolonged droughts, or vice versa. The temporal variability or simultaneous occurrence of multiple stresses during specific plant growth stages can significantly influence their damage and shape their adaptive responses.
Flooding of plants leading to low O2 responses can occur with varying intensity, both in agriculture and in natural environments. Examples include (a) flooded Rumex crispus, (b) partially submerged Meionectes brownii, (c) Tecticornia pergranulata flooded with saline water (note the dead Melaleuca trees in the background), (d) flooded maize (Zea mays), (e) partially submerged sugar beet (Beta vulgaris), and (f) almost completely submerged wheat (Triticum aestivum). Flooding and low O2 responses of plants constitute a compound stress and manifest at multiple levels. Therefore, a transdisciplinary research approach (g) is required to understand stress responses and to utilise this knowledge in breeding attempts aimed at enhancing the flood resilience of our crops. Photos by Ole Pedersen and partly created with BioRender.com.
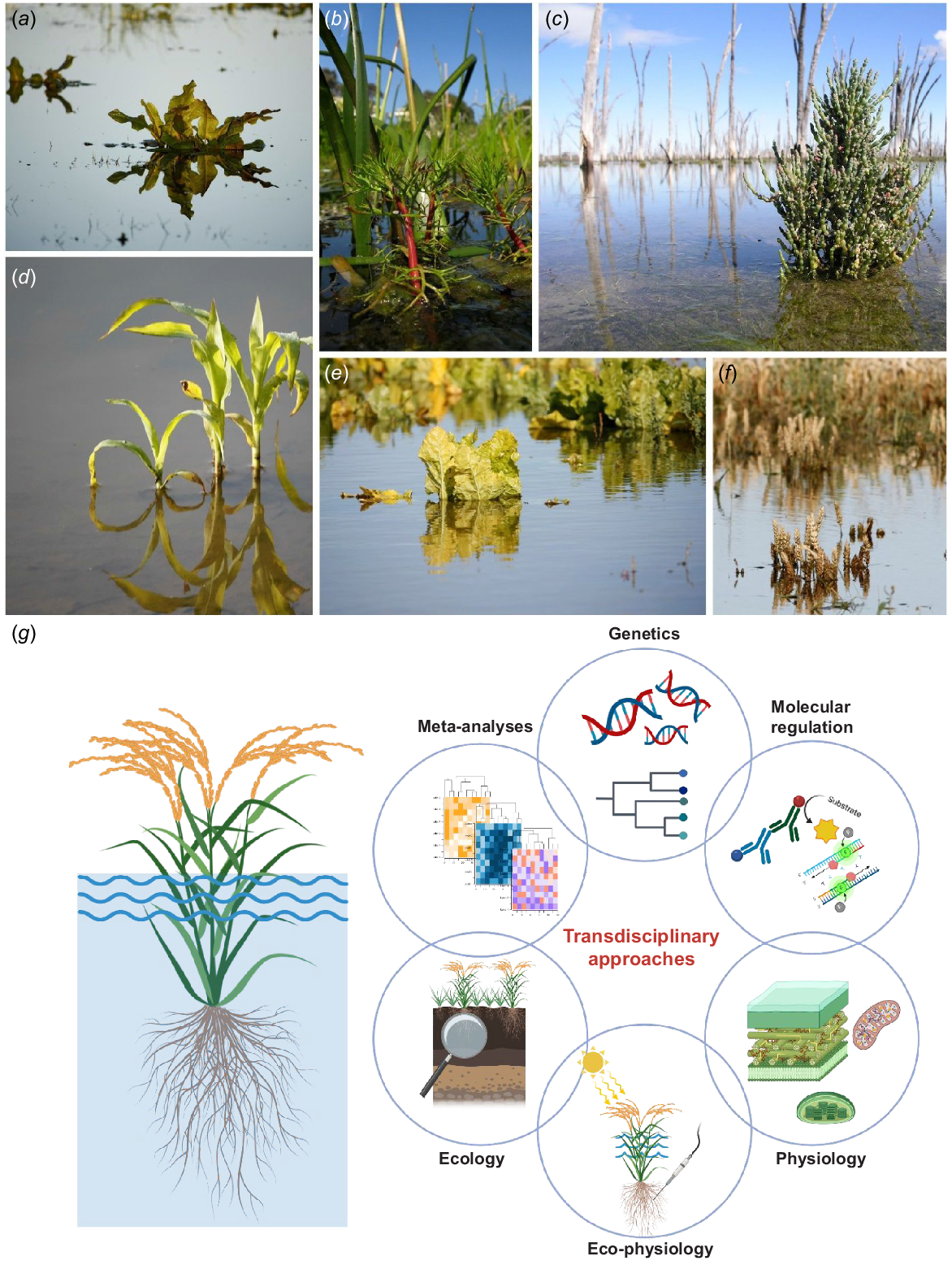
The pertinent importance of studying multiple stresses
Large areas of flooded agricultural land or those in proximity to coastal zones are often saline, containing phytotoxic concentrations of Na2+ and Cl− (Barrett-Lennard 2003). Common strategies of salt tolerance in plants include salt exclusion from sensitive tissues, vacuolar storage, or the restriction of salt entry into roots (Flowers and Colmer 2008). However, such responses can be hampered when plants are exposed to soil waterlogging with saline water, as O2 deficiency in roots leads to decline in ion (i.e. K, Na2+, Cl−) transport capacity and regulation (Kotula et al. 2015). Therefore, the combination of flooding and salinity is potentially more deleterious than either of these stresses individually (Fig. 1c). In practice, the characterisation of plant responses to combined stress conditions and the identification of associated traits of tolerance, as well as the molecular signalling related with those mechanisms of adaptation, may appear complicated. In this regard, evaluations of plants exhibiting high tolerance to both flooding and salinity, such as Salicornia europea (Jordine et al. 2024), or the evaluation of large populations of Festuca arundinacea (Menon-Martínez et al. 2024), appear to be excellent resources for traits discovery and selection of outstanding genotypes.
Temporal changes in the water load within soils (for changes in the water table above soil, see section below) can also shape plant responses. In this Collection, Jordine and collaborators found that regular tidal flooding for 5 weeks did not affect growth of S. europea, whereas only 10 days of continuous flooding stopped plant growth (Jordine et al. 2024). However, the anatomical plasticity of plant species might play an important role in their acclimation ability. Yamauchi and collaborators found that a larger cortex and aerenchyma formation in roots facilitate internal O2 diffusion from tissues above water down to the roots of Phragmites australis when grown in waterlogged soils (Yamauchi et al. 2024). This is in stark contrast to a larger stele tissue with more xylem vessels facilitating water movement in Phragmites japonica growing under water deficit conditions. Increased root porosity not only facilitates greater O2 diffusion in flooded soils but can also improve waterlogging and drought tolerance by reducing metabolic costs in the root resulting from a reduced number of root cells (Chimungu et al. 2014). On the contrary, a bulky tissue geometry of low porosity can generate hypoxic conditions due to both increased resistance to O2 diffusion and cellular O2 consumption, even under aerated environments (Brunello et al. 2024; Jiménez et al. 2024).
In addition to changes in root porosity, a barrier to restrict radial O2 loss (ROL) is formed in roots of flood-tolerant plants when these grow in waterlogged soils. Such barriers are characterised by depositions of lignin and/or suberin in cell walls of the sclerenchyma, exodermis, or epidermis and appear as a key root trait for tolerance to multiple stresses (Peralta Ogorek et al. 2024). The formation of apoplastic barriers in the outer part of the root facilitates internal O2 diffusion by impeding ROL from roots to the rhizosphere. This barrier also restricts water loss from roots (Peralta Ogorek et al. 2023) and the entry of soil phytotoxins in the opposite direction (from soils to the roots; i.e. NaCl, Ranathunge et al. 2011; reduced iron, Jiménez et al. 2021; hydrogen sulfide, Peralta Ogorek et al. 2023). Consequently, the outer apoplastic barriers play an important role in scenarios involving multiple stress conditions, as the barriers can impede O2 and water loss from roots, while also preventing the entry of salts or other phytotoxins from rhizosphere to roots. However, more research is needed to evaluate the potential drawbacks of such barriers especially for nutrient and/or water uptake.
Type of stress – intensity of the stress condition
In lowland regions or during periods of heavy rainfall, water levels can rise in poorly drained soils, causing partial or even complete submergence of plants, thereby further hampering plant growth. The longer the diffusional path between the atmosphere and the submerged tissues; i.e. the deeper the flood, the lower the net O2 flux; therefore, deeper water tables above the plant imply less O2 availability for plant growth. Complete submergence not only affects O2 diffusion for respiration but also CO2 diffusion for photosynthesis. As such, plant responses to hypoxic conditions, including molecular signalling, upregulation of hypoxic genes, anatomical changes and overall plant responses to such conditions vary widely between different water levels. These responses are detailed by Lin and colleagues in this issue. In waterlogged soils, aerenchyma formation and the development of barriers to impede ROL facilitate internal O2 diffusion from shoots above water down to submerged roots (Lin et al. 2024; Peralta Ogorek et al. 2024). In situations of partial submergence, the plant may escape submergence by elongating the shoots to enhance contact with air or, alternatively, may become quiescent by stopping growth to conserve resources (Hattori et al. 2009). Additionally, an increased formation of aquatic adventitious roots developed from submerged stem internodes can enhance O2 uptake from O2 dissolved in the floodwater (Lin et al. 2021). In the extreme case of complete submergence, the formation of gas films in leaves allow diffusion of molecular O2 and CO2 dissolved in water into the leaf (Colmer and Pedersen 2008) and therefore these gas films might play an important role in internal aeration under such conditions.
Evaluations of plant responses during continuous periods of waterlogging, partial or complete submergence, compared to non-stress controls, represent the basis of our current understanding of how plants respond to such conditions. However, plant responses during the recovery phase after the flood has receded have often been overlooked (Striker 2012). Stunted growth during flooding does not necessarily indicate poor performance under stress, nor does enhanced growth during stress indicate tolerance. In fact, the latter can lead to depletion of resources, hampering further growth in the recovery phase. In this Collection, Buraschi et al. (2024) found that some genotypes of the forage legume Lotus spp. exhibited better growth under partial or complete submergence, whereas other Lotus genotypes grew better during the recovery phase. Furthermore, the same study revealed that there was no apparent correlation between the intrinsic growth ability under non-stress conditions and growth under submergence stress. In contrast, Menon-Martínez and colleagues (this Collection 2024) evaluated 39 tall fescue (Festuca spp.) genotypes and indicated that more productive accessions under non-stress conditions also showed greater stress tolerance (Menon-Martínez et al. 2024). These differences in response to stress compared to non-stress conditions are highly relevant, as breeding efforts should prioritise high productivity under both stressful environments and optimal growth conditions.
Flooding can also affect plant growth at different stages. The impact on productivity differs among crops; field peas are severely affected at any stage, early flooding greatly impacts wheat production, whereas barley (Hordeum vulgare) and rapeseed (Brassica napus) can still produce significant yield if transient flooding occurs during early rather than at late stages (Ploschuk et al. 2018). Flooding during seed germination stages can hamper crop seed germination while favouring the germination of better adapted weed species (Echeverry Holguín et al. 2024). Flood-adapted plants like rice can germinate under water, outcompeting weeds, but flooding during germination stages can be deleterious for other non-adapted crops, and underwater germination can benefit weed survival. After successful germination, constitutive aerenchyma formation in populations of black-grass (Alopecurus myosuroides) weeds, but not in wheat genotypes, facilitated rapid establishment of the former when challenged by waterlogging (Harrison et al. 2024). Several environmental and intrinsic factors including temperature and nutrient conditions (Echeverry Holguín et al. 2024), transcriptomic and metabolomic responses (Harrison et al. 2024), and the composition of bacterial microbiota inhabiting the seeds (Gómez-Álvarez et al. 2024) play important roles in determining the ability of a seed to germinate and/or grow under water or under hypoxic conditions.
Transdisciplinary research
It requires transdisciplinary research efforts in order to identify mechanisms of adaptation, as complex stress condition can vary in intensity and affect different stages of plant development and/or the normal functioning of different plant tissues (Fig. 1g). A set of genes, including some required for fermentative metabolism, redox management or simply those involved in O2 sensing, have been widely identified in the model plant Arabidopsis thaliana in response to hypoxia (Mustroph et al. 2009; Weits et al. 2014). Some of these hypoxia-responsive genes were found to be strongly induced in the aerial, yet hypoxic roots of Phalaenopsis orchids (Brunello et al. 2024), or in the shoots of the halophyte S. europea in response to flooding (Jordine et al. 2024). This indicates that similar responses to hypoxia stress are conserved across different plant species or tissues, and that the vast molecular information generated in the model plant Arabidopsis could be used to integrate the molecular responses of crops to flooding conditions. Interestingly, the upregulation of metabolism-based herbicide resistance genes in shoots in response to root waterlogging (Harrison et al. 2024), or the higher expression of hypoxia-related genes followed by salinity treatments (Jordine et al. 2024), suggest a parallel stress response to different stress conditions that deserves further investigation.
Root anatomical traits, including aerenchyma development and the formation of barriers to ROL are common adaptive responses of plants to waterlogging, droughts, and saline conditions (Peralta Ogorek et al. 2023; Yamauchi et al. 2024). The known effect of ethylene in inducing aerenchyma but not a barrier to ROL (Colmer et al. 2006), and conversely, the induction of the barrier to ROL following applications of ABA but with no concomitant effect on aerenchyma formation (Shiono et al. 2022), indicate that these acclimations are differentially regulated. While the molecular mechanisms of O2 sensing in hypoxic conditions are well-described in Arabidopsis (e.g. reviewed in León et al. 2021; Loreti and Perata 2023; Renziehausen et al. 2024), further research is needed on the specific signalling pathways and the molecular regulation of traits conferring hypoxia tolerance, as well as their functional characterisation and translation of such resources into flooding-sensitive crops. Excitingly, excellent examples of the use of molecular tools characterising genes responsive to hypoxia, tissue metabolic responses to hypoxia, tissue O2 status, anatomical changes facilitating effective O2 diffusion, and whole-plant responses to waterlogging, partial or complete submergence, or a combination of flooding with other stress conditions are well described in this Collection on ‘Flooding stress and responses to hypoxia in plants’. These examples represent the diverse efforts of the research community to understand how plants respond to low-O2 conditions. The evaluation of undomesticated target genotypes or large populations of crops are key strategies to develop genotypes with outstanding adaptation to such stressful conditions, and we believe that transdisciplinary approaches are essential to tackle the deleterious effect of flooding in crops.
Conflicts of interest
The authors are the Guest Editors of the ‘Flooding Stress and Responses to Hypoxia in Plants’ Collection but were blinded from the peer review process for this paper.
Acknowledgements
The authors thank all authors and reviewers that contributed to this Collection. We acknowledge funding from European Union under a Marie Skłodowska-Curie Postdoctoral Fellowship (SmartRoots, grant No. 101061962 to JdlCJ), Danish International Development Agency (Climate-smart African rice, grant No. 19-03-KU to OP), the Netherlands Organisation for Scientific Research grant (VIDI.213.055) and ERC-Stg (LOKI, grant No. 101077812). The authors thank the Deutsche Forschungsgesellschaft (DFG) for grant number SCHM3345/2-1.
References
Barrett-Lennard EG (2003) The interaction between waterlogging and salinity in higher plants: causes, consequences and implications. Plant and Soil 253(1), 35-54.
| Crossref | Google Scholar |
Brunello L, Polverini E, Lauria G, Landi M, Guidi L, Loreti E, Perata P (2024) Root photosynthesis prevents hypoxia in the epiphytic orchid Phalaenopsis. Functional Plant Biology 51, FP23227.
| Crossref | Google Scholar |
Buraschi FB, Mollard FPO, Di Bella CE, Grimoldi AA, Striker GG (2024) Shaking off the blow: plant adjustments during submergence and post-stress growth in Lotus forage species. Functional Plant Biology 51(1), FP23172.
| Crossref | Google Scholar |
Chimungu JG, Brown KM, Lynch JP (2014) Reduced root cortical cell file number improves drought tolerance in maize. Plant Physiology 166(4), 1943-1955.
| Crossref | Google Scholar | PubMed |
Colmer TD, Pedersen O (2008) Oxygen dynamics in submerged rice (Oryza sativa). New Phytologist 178(2), 326-334.
| Crossref | Google Scholar | PubMed |
Colmer TD, Cox MC, Voesenek LA (2006) Root aeration in rice (Oryza sativa): evaluation of oxygen, carbon dioxide, and ethylene as possible regulators of root acclimatizations. New Phytologist 170(4), 767-777.
| Crossref | Google Scholar |
Echeverry Holguín J, Crepy M, Striker GG, Mollard FPO (2024) Boosting underwater germination in Echinochloa colona seeds: the impact of high amplitude alternating temperatures and potassium nitrate osmopriming. Functional Plant Biology 51(1), FP23184.
| Crossref | Google Scholar |
Flowers TJ, Colmer TD (2008) Salinity tolerance in halophytes. New Phytologist 179(4), 945-963.
| Crossref | Google Scholar | PubMed |
Gómez-Álvarez EM, Salardi-Jost M, Ahumada GD, Perata P, Dell’Acqua M, Pucciariello C (2024) Seed bacterial microbiota in post-submergence tolerant and sensitive barley genotypes. Functional Plant Biology 51(1), FP23166.
| Crossref | Google Scholar |
Harrison C, Noleto-Dias C, Ruvo G, Hughes DJ, Smith DP, Mead A, Ward JL, Heuer S, MacGregor DR (2024) The mechanisms behind the contrasting responses to waterlogging in black-grass (Alopecurus myosuroides) and wheat (Triticum aestivum). Functional Plant Biology 51, FP23193.
| Crossref | Google Scholar |
Hattori Y, Nagai K, Furukawa S, Song X-J, Kawano R, Sakakibara H, Wu J, Matsumoto T, Yoshimura A, Kitano H, Matsuoka M, Mori H, Ashikari M (2009) The ethylene response factors SNORKEL1 and SNORKEL2 allow rice to adapt to deep water. Nature 460(7258), 1026-1030.
| Crossref | Google Scholar | PubMed |
Jiménez JdlC, Clode PL, Signorelli S, Veneklaas EJ, Colmer TD, Kotula L (2021) The barrier to radial oxygen loss impedes the apoplastic entry of iron into the roots of Urochloa humidicola. Journal of Experimental Botany 72(8), 3279-3293.
| Crossref | Google Scholar | PubMed |
Jiménez JdlC, Armstrong W, Colmer TD, Pedersen O (2024) Overcoming constraints to measuring O2 diffusivity and consumption of intact roots. Plant Physiology kiae046.
| Crossref | Google Scholar |
Jordine A, Retzlaff J, Gens L, Ehrt B, Fürtauer L, van Dongen JT (2024) Introducing the halophyte Salicornia europaea to investigate combined impact of salt and tidal submergence conditions. Functional Plant Biology 51, FP23228.
| Crossref | Google Scholar |
Kotula L, Clode PL, Striker GG, Pedersen O, Lauchli A, Shabala S, Colmer TD (2015) Oxygen deficiency and salinity affect cell-specific ion concentrations in adventitious roots of barley (Hordeum vulgare). New Phytologist 208(4), 1114-1125.
| Crossref | Google Scholar | PubMed |
León J, Castillo MC, Gayubas B (2021) The hypoxia-reoxygenation stress in plants. Journal of Experimental Botany 72(16), 5841-5856.
| Crossref | Google Scholar | PubMed |
Lin C, Ogorek LLP, Pedersen O, Sauter M (2021) Oxygen in the air and oxygen dissolved in the floodwater both sustain growth of aquatic adventitious roots in rice. Journal of Experimental Botany 72(5), 1879-1890.
| Crossref | Google Scholar | PubMed |
Lin C, Zhang Z, Shen X, Liu D, Pedersen O (2024) Flooding-adaptive root and shoot traits in rice. Functional Plant Biology 51, FP23226.
| Crossref | Google Scholar |
Loreti E, Perata P (2023) ERFVII transcription factors and their role in the adaptation to hypoxia in Arabidopsis and crops. Frontiers in Genetics 14, 1213839.
| Crossref | Google Scholar | PubMed |
Menon-Martínez FE, Grimoldi AA, Striker GG, Di Bella CE (2024) Changes in morphological traits associated with waterlogging, salinity and saline waterlogging in Festuca arundinacea. Functional Plant Biology 51(1), FP23140.
| Crossref | Google Scholar |
Mustroph A, Zanetti ME, Jang CJH, Holtan HE, Repetti PP, Galbraith DW, Girke T, Bailey-Serres J (2009) Profiling translatomes of discrete cell populations resolves altered cellular priorities during hypoxia in Arabidopsis. Proceedings of the National Academy of Sciences of the United States of America 106(44), 18843-18848.
| Crossref | Google Scholar | PubMed |
Peralta Ogorek LL, Takahashi H, Nakazono M, Pedersen O (2023) The barrier to radial oxygen loss protects roots against hydrogen sulphide intrusion and its toxic effect. New Phytologist 238(5), 1825-1837.
| Crossref | Google Scholar | PubMed |
Peralta Ogorek LL, Jiménez JdlC, Visser EJW, Takahashi H, Nakazono M, Shabala S, Pedersen O (2024) Outer apoplastic barriers in roots: prospects for abiotic stress tolerance. Functional Plant Biology 51(1), FP23133.
| Crossref | Google Scholar |
Ploschuk RA, Miralles DJ, Colmer TD, Ploschuk EL, Striker GG (2018) Waterlogging of winter crops at early and late stages: impacts on leaf physiology, growth and yield. Frontiers in Plant Science 9, 1863.
| Crossref | Google Scholar | PubMed |
Ranathunge K, Lin J, Steudle E, Schreiber L (2011) Stagnant deoxygenated growth enhances root suberization and lignifications, but differentially affects water and NaCl permeabilities in rice (Oryza sativa L.) roots. Plant, Cell & Environment 34(8), 1223-1240.
| Crossref | Google Scholar | PubMed |
Renziehausen T, Frings S, Schmidt-Schippers R (2024) ‘Against all floods’: plant adaptation to flooding stress and combined abiotic stresses. The Plant Journal 117(6), 183 6-1855.
| Crossref | Google Scholar |
Shiono K, Yoshikawa M, Kreszies T, Yamada S, Hojo Y, Matsuura T, Mori IC, Schreiber L, Yoshioka T (2022) Abscisic acid is required for exodermal suberization to form a barrier to radial oxygen loss in the adventitious roots of rice (Oryza sativa). New Phytologist 233(2), 655-669.
| Crossref | Google Scholar |
Striker GG (2012) Time is on our side: the importance of considering a recovery period when assessing flooding tolerance in plants. Ecological Research 27(5), 983-987.
| Crossref | Google Scholar |
Weits DA, Giuntoli B, Kosmacz M, Parlanti S, Hubberten H-M, Riegler H, Hoefgen R, Perata P, van Dongen JT, Licausi F (2014) Plant cysteine oxidases control the oxygen-dependent branch of the N-end-rule pathway. Nature Communications 5(1), 3425.
| Crossref | Google Scholar |
Yamauchi T, Sumi K, Morishita H, Nomura Y (2024) Root anatomical plasticity contributes to the different adaptive responses of two Phragmites species to water-deficit and low-oxygen conditions. Functional Plant Biology 51, FP23231.
| Crossref | Google Scholar |