Melatonin improves drought stress tolerance of pepper (Capsicum annuum) plants via upregulating nitrogen metabolism
Cengiz Kaya

A Soil Science and Plant Nutrition Department, Agriculture Faculty, Harran University, Sanliurfa, Turkey.
B Tasmanian Institute of Agriculture, University of Tasmania, Hobart, Tas., Australia.
C School of Biological Science, University of Western Australia, Crawley, WA, Australia.
D International Research Centre for Environmental Membrane Biology, Foshan University, Foshan, China.
Handling Editor: Muhammad Waseem
Abstract
While ameliorating effects of melatonin (MT) on abiotic stress tolerance in plants are widely reported, the mechanism that underlies this process remains elusive. This work investigated mechanisms by which MT improved drought tolerance in pepper (Capsicum annuum) plants. A foliar spray of 0.1 mM MT treatment was applied to plants grown at 80% and 40% of full field capacity for 3 days. Drought stress caused a significant decrease in plant dry weight, relative water content, leaf water potential, PSII efficiency (Fv/Fm ratio), chlorophyll, soluble protein, leaf and root nitrogen content. Drought increased hydrogen peroxide, malondialdehyde (MDA), nitrate, ammonium, free amino acids, soluble sugars, proline and glycine betaine. Drought also increased peroxidase (POD), glutathione S-transferase (GST) and catalase (CAT) activities, electrolyte leakage (EL) and methylglyoxal (MG). MT pre-treatment reduced oxidative stress and improved nitrogen metabolism by activating various enzymes such as nitrate reductase (NR), nitrite reductase (NiR), glutamine synthetase (GS), glutamate synthetase (GOGAT) and glutamine dehydrogenase (GDH) activities. It also activated enzymes related to the glyoxalase system (Gly I and Gly II) and decreased NO3−, NH4+ and free amino acid content. Our study suggests a cost-effective and sustainable solution to improve crop productivity in water-limited conditions, by enhancing plant growth, photosynthesis and nitrogen content.
Keywords: antioxidants, glyoxalase system, melatonin, nitrogen, nitrogen metabolism, pepper, ROS, water stress.
Introduction
Sweet pepper (Capsicum annuum) is among the crops most commonly produced in the Mediterranean region (Rodríguez et al. 2020). Drought is among the most critical environmental concerns in agriculture today, especially in dry and semi-dry climates including the Mediterranean area (Huang et al. 2021). Plant biologists have focused their efforts on studying the deleterious effects of drought on plants as well as techniques for mitigating those effects (Ahluwalia et al. 2021; Ahmad et al. 2022). Water supplies used for irrigation in agricultural output are progressively depleted due to climate change-driven increase in drought frequency and severity (Liu et al. 2020; Rawat et al. 2022) and population growth (Rocha et al. 2020). Drought stress inhibits plant growth and yield by reducing its photosynthetic capacity and results in an imbalance in the cellular redox modules, with antioxidant defences failing to counterbalance the increased creation of reactive oxygen species (ROS) (Sun et al. 2018). This leads a cascade of oxidative damage, impairing development and growth (Kerchev and Van Breusegem 2022). Drought stress can result in the accumulation of methylglyoxal (MG), a compound generated during the glycolysis process that can harm the membranes of cells by destroying lipids and proteins at high concentrations (Dorion et al. 2021). Therefore, it is essential for plants to eliminate MG accumulation by upregulating enzymes associated with the glyoxalase system (Talaat and Todorova 2022).
The major enzymes, glutamate synthetase (GOGAT), nitrate reductase (NR), glutamine synthetase (GS) and glutamine dehydrogenase (GDH), are involved in the metabolism of nitrogen in crop plants (He et al. 2022; Rizwan et al. 2022) and their activities accurately represent the degree of crop N absorption (Xia et al. 2020).
Drought stress dramatically lowers the soil water potential, which prevents the delivery of nitrogen (N) to the root surface, slows down root growth and significantly limits the available surface area of the root for nutrient absorption (Wang et al. 2019; Cao et al. 2022). Also, metabolic abnormalities brought about by a water shortage in the plant influence the synthesis, breakdown and metabolism of nitrogenous substances in plants (Ozturk et al. 2021). Among the most significant ways that plants have evolved to deal with drought is through regulation of nitrogen metabolism (Du et al. 2020a).
The NR and nitrite reductase (NiR) work together to change the nitrate absorbed by the plants itself to ammonium (Yoneyama and Suzuki 2019). The amino acids synthesised by a sequence of N assimilation enzymes, such as GDH, GS and GOGAT, can then be used for the production of biomacromolecular compounds (Cañas et al. 2020). Therefore, N absorption and transport can be promoted in plants by raising N metabolic enzymes’ activity (Zhang et al. 2020).
The use of bio-stimulators can reduce the negative impact of abiotic stresses (including drought) on plant performance (Altaf et al. 2022a). Among the several defensive substances naturally produced inside plants, melatonin (MT) seems to be a promoting bio-stimulator against water stress (Nawaz et al. 2021), and it is crucial in a variety of biological activities, including photosynthesis (Yan et al. 2021), nitrogen metabolism regulation (Ren et al. 2022) and ROS scavenging (Gao et al. 2022) in challenging conditions. The mitigating impact of MT has been reported for a diverse range of plant species grown under drought conditions, such as tomato (Solanum lycopersicum) (Ibrahim et al. 2020; Altaf et al. 2022a), cotton (Gossypium hirsutum) (Hu et al. 2021), tobacco (Nicotiana tabacum) (Liu et al. 2021) and Phoebe sheareri (Li et al. 2023). However, the mechanistic basis of such mitigation remains to be explored in pepper. While previous studies have briefly mentioned the mitigating effects of melatonin on drought stress in other crops, our study provides novel insights into the mechanistic basis of this mitigation through the modulation of nitrogen metabolism.
In this work, we have hypothesised that exogenous melatonin application may increase plant resilience to water scarcity by improving N metabolism. MT was found to activate a range of enzymes related to nitrogen metabolism, such as NR, NiR, GS, GOGAT and GDH, resulting in improved nitrogen content, photosynthetic traits and, ultimately, plant growth. It is therefore concluded that exogenous application of MT could provide an affordable and straightforward solution to the production of crops under conditions of limited water availability.
Materials and methods
Plant cultivation and treatments
Sweet pepper (Capsicum annuum L.) plants of the variety ‘Semerkand F1’, supplied by Nunhems Seeds Company, were grown in a greenhouse at the research station of Harran University, Sanliurfa, Turkey (37°10′13″N, 39°00′07″E), from the beginning of May 2022 to the beginning of June 2022. After being surface sterilised in a solution of 1% NaOCl (v/v), pepper seeds were sown in a plate with washed sand and germinated under controlled plant growth conditions, with day time and night time temperatures fixed at 25 ± 2°C and 15 ± 2°C, respectively. One week after germination, seedlings were planted into a 5-L container holding dry soil and sand in an equal volume (two plants per container). A 0.10 mM MT containing Tween-20 (0.01%) was sprayed to half seedlings once a day for 3 days before starting the drought treatment. The concentration of 0.10 mM melatonin content used in the experiment was based on prior research with pepper plants (Kaya et al. 2022). The spraying solution was calibrated to 25 mL per pot and the foliar application was administered until the entire plant was thoroughly wetted. A pre-treatment period of three consecutive days with MT solutions was implemented before the onset of drought stress to ensure that the plants had taken up MT within their cells, following a methodology similar to a previous study conducted with pepper (Kaya et al. 2022). All seedlings were then divided into two groups (control and drought-affected; see Supplementary Fig. S1 for details). The control plants were treated with a solution containing only Tween-20 (0.01%). For control and drought treatment, the availability of soil water was kept at 80% and 40% of total water holding potential, respectively. The type of soil was clay loam and its parameters included 25.2% CaCO3, a pH of 7.8, 1.2% organic matter, 3.2 mg kg−1 of available P and 1.4 dS m−1 of electrical conductivity. The exchangeable cation concentrations of Ca2+, K+, Mg2+ and Na+ were 25.7, 1.38, 12.9 and 0.69 cmol kg−1, respectively. The pH of irrigation water was 7.3 and the electrical conductivity was 0.54 dS m−1. Nitrogen, P2O5 and K2O were applied to the soil in amounts of 100, 50 and 120 mg kg−1 respectively, in the form of granular urea, triple superphosphate and potassium sulfate. Day time and night time temperatures were fixed at 25 ± 2°C and 15 ± 2°C, respectively. During the trial, the duration of illuminance remained around 11 h.
The total soil water holding capacity was calculated before starting the drought treatment by examining three pots with drilled holes in each, which is a one-time measurement to determine the soil’s baseline water holding capacity. Air-dried soil and washed sand were placed in equal parts in each 5-L container, which was then filled completely with distilled water and left to drain for a day. After reweighing the pots, the equation of Bonfim-Silva et al. (2015) was employed to calculate the soil’s total capacity for holding water.
Similarly, the amount of water lost during the experiment was measured daily using the gravimetric method of da Silva Leite et al. (2019), which involves weighing the pots twice daily and adding back the water consumed by plants and evaporated water. These measurements were used to monitor the water status of the plants and ensure consistent drought stress treatment.
Each replicate consisted of three pots, with two plants per pot, and the experiment was repeated three times, resulting in a total of 18 plants per treatment. For determination of shoot and root dry weights, six plants were randomly selected from each treatment. After measuring the plant fresh weight, samples were dried in an oven set to 75°C for a period of 3 days. The remaining 12 plants from each treatment were used for physiological and biochemical assays, with the youngest fully developed leaves selected for analysis.
Chlorophyll content and operation of PSII
The content of leaf chlorophyll was measured as per the procedure of Strain and Svec (1966) using 90% acetone extract. The absorbance values for chlorophyll a and chlorophyll b were determined at 663.5 nm and 645 nm, respectively. The measurement of chlorophyll fluorescence was carried out with a Mini-PAM chlorophyll fluorometer (Walz, Germany) using dark-adapted samples. Parameters such as maximal, minimal and variable fluorescence (Fm, Fo and Fv, respectively) were recorded, and the maximum quantum efficiency of PSII (Fv/Fm ratio) was evaluated.
Leaf water relations
A Pressure Chamber Instrument (PMS model 600, USA) was used to measure the leaf water potential. To calculate leaf relative water content (RWC), the method of Yamasaki and Dillenburg (1999) was used. After weighting leaf samples, they were put inside the vials with distilled water for incubation for a day, after which time the leaf was cleared of any surface water and the leaf turgid weight (TW) was appraised. To determine the leaf dry weight (DW), these samples were finally oven-dried to a consistent weight at 65°C. RWC was computed using the following equation: RWC = [(FW – DW)/(TW – DW)] × 100.
Thermal image processing and analysis
Infra-red imaging using a thermal camera (FLIR T540) was used to measure canopy temperatures during the daytime when the canopy was at its warmest. The camera was mounted on a tripod, adjusted for emissivity, and captured thermal images of the entire canopy. The images were processed using FLIR Tools software to measure temperatures, and the mean temperature values were calculated. To obtain a representative sample of the canopy, six readings were taken in total, with two readings taken from each side of the plant, selected from various directions.
Leaf free amino acid and proline content
The leaf free amino acids and proline content were measured using the procedures described by Rosen (1957) and Bates et al. (1973), respectively. A sulfosalicylic acid (3%, 10 mL) solution was used to extract 0.5 g of leaf tissue. Each filtrate sample received 2 mL of glacial acetic acid (60% v/v) and acid-ninhydrin (2.5% w/v) solutions. The solution was then heated in a 100°C water bath for 1 h. The quantities of free amino acids and proline were measured using a spectrophotometer at A520 nm and A580 nm, respectively.
Glycine betaine (GB), soluble sugar and soluble proteins
To assess the GB content, the procedure of Grieve and Grattan (1983) was employed. First, the samples of dried leaves were powdered. After adding 20 mL of deionised water to the dry sample, it was shaken at 25°C for 24 h. A 2N H2SO4 at a 1:1 ratio was then treated with the prepared sample. To initiate the reaction, a 0.5 mL of the extract was treated with cool potassium iodide (0.2 mL). The sample mixture underwent a complete 15-min 10 000g centrifugation. A measurement of absorbance at 365 nm was taken. The GB standards ranged from 50 to 200 μg mL−1 and were prepared with 2N H2SO4.
Soluble sugars content was quantified as described by Fales (1951) method. Excised leaves were placed in 15 mL of distilled water and then permitted to boil in a water bath for 20 min before cooling naturally. A 3 mL of anthrone was added to the boiling sample. The absorbance at 620 nm was measured
The spectrophotometric technique described by Smith et al. (1985) was adopted to estimate the soluble proteins concentration. In a nutshell, 0.1 M phosphate buffer (pH 6.75) was used to pulverise 1.0 g of freshly obtained leaf tissue. Each tube had 1.5 mL of the bicinchoninic acid (BCA) reagent and 5 μL of the sample supernatant was mixed after being centrifuged at 15 000g for 15 min. After 5 min of heating in boiling water, the samples were then cooled to the ambient temperature. At 562 nm, the absorbance readings were recorded.
Hydrogen peroxide (H2O2) and malondialdehyde (MDA) content
The protocol of Velikova et al. (2000) was used to measure the leaf H2O2. A 3 mL solution of 1% TCA was used to extract 500 mg of fresh leaf material. The OD at 390 nm was measured. Leaf MDA was quantified as per Weisany et al. (2012). Following the homogenisation of 200 mg of fresh leaf tissue in trichloroacetic acid (TCA, 5 mL), the mixture was centrifuged at 12 000g for 5 min. Then, 1 mL of the sample solution was mixed with 4 mL of 0.5% thiobarbituric acid (processed in 20% TCA). The mixture solution was incubated to in a 90°C water bath for 30 min. After the mixture had cooled, absorbances at 532 nm were recorded.
Electrolyte leakage (EL)
The measurement of EL was conducted using the method described by Dionisio-Sese and Tobita (1998). Leaf samples were stored in deionised water-filled vials at ambient temperature for 24 h on a rotating shaker for measurement of the first electrolyte conductivity (EC1). After 20 min of autoclaving at 120°C, the second electrolyte conductivity (EC2) was measured. The EL was computed as per the equation below:
Antioxidant enzymes
Fresh leaf tissue weighing 0.5 g was extracted using 1% soluble polyvinyl pyrrolidine and Na-P buffer (50 mM). The extracted solution was then centrifuged at 20 000g for 15 min. All enzyme activities were tested using the extract solution. Catalase (CAT) and peroxide (POD) activity were quantified as per the protocol of Chance and Maehly (1955). In order to measure glutathione S-transferase (GST) activity, the procedure of Hossain et al. (2006) was employed. Gly I and Gly II activities were quantified as per the methods of Chakravarty and Sopory (1998) and Principato et al. (1987), respectively.
Methylglyoxal (MG) levels
After being homogenised in 5% perchloric acid with a 500 mg leaf material, the extract was centrifuged at 11 000g for 10 min at 4°C. A concentrated solution of potassium carbonate was also employed to neutralise the colour at 25°C after removing the colour from the supernatant by adding charcoal. Absorbance values were recorded at 288 nm (Wild et al. 2012).
Key enzymes of N metabolism
In order to assay the NR and NiR activities, fresh leaf tissue (1:5 w/v) was extracted in a chilled pestle and mortar with 100 mM potassium phosphate buffer. The sample solution was centrifuged and the filtrate was obtained for NR and NiR activity measurement.
The Debouba et al. (2006) method was used to measure NR activity. The extract was treated with NADH to kick off the reaction and then was incubated at 27°C for 30 min, combined with 500 mM zinc acetate (0.1 mL). The production of nitrite was established following the generation of diazotation. The absorbances at 540 nm were measured once it had cooled to a room temperature. A NaNO2 calibration graph was used to determine the amount of nitrite. A decrease in the NO2 quantity was employed to assess the NiR activity (Debouba et al. 2006). The reaction was started by adding a 0.1 M NaHCO3 solution containing 4.3 mM sodium dithionite to the reaction mixture. The reaction was halted by stirring and boiling for 1 min at 27°C for 30 min. At 540 nm, the remaining NO2 ions in the solution were recorded.
Glutamine synthetase, glutamate dehydrogenase and glutamate synthase
Fresh leaf tissue was triturated in 50 mM Tris-HCl buffer (pH 7.6) containing 1 mM EDTA, 1 mM MgCl2, 10 mM β-mercaptoethanol, 1 mM dithiothreitol and 0.5% polyvinylpyrrolidone (PVP) to quantify the activities of GS and GDH. The GS activity was measured as per the protocol of Agbaria et al. (1998). A sample cocktail (2.0 mL) included 1 mM ADP, the enzyme extract, 50 mM glutamine, MgCl2 (20 mM), Tris-HCl buffer (50 mM) and sodium arsenate (20 mM). The OD was read at 540 nm after centrifuging at 3000g for 10 min. The activity GDH was quantified by recording NADH oxidation at 340 nm as per Groat and Vance (1981).
Fresh leaf material (1:5, w/v) was extracted in potassium phosphate buffer (50 mM) containing 1 mM phenylmethylsulfonyl fluoride (PMSF), 10 mM KCl, 2 mM EDTA, ethylene glycol (3.58 M) and 14 mM β-mercaptoethanol for the measurement of GOGAT activity. After centrifuging the sample solution at 20 000g for 20 min, an aliquot was taken and used to quantify the NADH-GOGAT activity. The protocol of Groat and Vance (1981) was employed to measure the NADH-GOGAT activity by screening for NADH oxidation at 340 nm.
Determination of total N, NO3 and NH4
The determination of total nitrogen content in dried plant leaf and root samples was performed using the Kjeldahl method. To measure the concentrations of NO3 and NH4, fresh leaf and root tissues were extracted in distilled water and then thoroughly warmed. To determine nitrate levels, the sample solution was prepared according to the procedure outlined in Cataldo et al. (1975). Specifically, the solution contained 0.1 mL of the filtrate, 0.2 mL of concentrated H2SO4 and 0.2 mL of 5% salicylic acid. Next, the extracted material was treated with 1 mL of 4 M NaOH. The absorbance of the resulting extract was measured at 410 nm after appropriate cooling.
The Nessler reagent was used to assess the concentration of ammonium according to Molins-Legua et al. (2006). To measure the concentration of ammonia in the sample, the reaction solution was prepared using 0.1 mL of the filtrate, the Nessler reagent, 0.01 mL of 10% K-Na tartrate and 2.4 mL of redistilled water. The optical density was then measured at 425 nm.
Results
Exogenous MT treatment enhanced drought tolerance, leaf water relations and transpiration cooling in pepper
To investigate the impact of exogenous melatonin on drought tolerance, various plant growth and photosynthesis-related parameters were evaluated. Under drought stress, the shoot, root and total dry mass of plants were significantly reduced (P ≤ 0.05) by 30%, 55% and 34%, respectively, compared to control plants (Fig. 1a–c). However, externally applied melatonin showed significant increases in plant growth-related attributes, including the shoot, root and total dry mass, by approximately 26%, 80% and 32%, respectively (Fig. 1a–c). No significant (P < 0.05) effects of melatonin treatment were observed under normal conditions. Additionally, under drought stress, pepper seedlings’ chlorophyll a and b concentrations, as well as PSII efficiency (Fv/Fm), were significantly decreased (P ≤ 0.05) compared to control plants, with reductions of 53%, 30% and 23%, respectively. However, when melatonin was externally applied under drought conditions, there were significant increases in these photosynthesis-related traits by 56%, 30% and 29%, respectively, compared to plants that were only exposed to drought stress (Fig. 1d–f). The melatonin treatment did not have a significant (P ≤ 0.05) effect on the traits of control plants.
Effect of melatonin (MT) on agronomical and photosynthetic characteristics of pepper plants exposed to drought conditions. (a) Shoot dry matter (DM), (b) root DM, (c) total plant DM, (d, e) chlorophyll a and b content and (f) chlorophyll fluorescence Fv/Fm ratio. Plants were sprayed either with 0.10 mM melatonin (+MT) or water (mock control; −MT). Data is mean ± s.e. (n = 6–8). Tukey’s test was performed to determine the significant differences between groups and bars labelled with different lower case letters are statistically different at P ≤ 0.05.
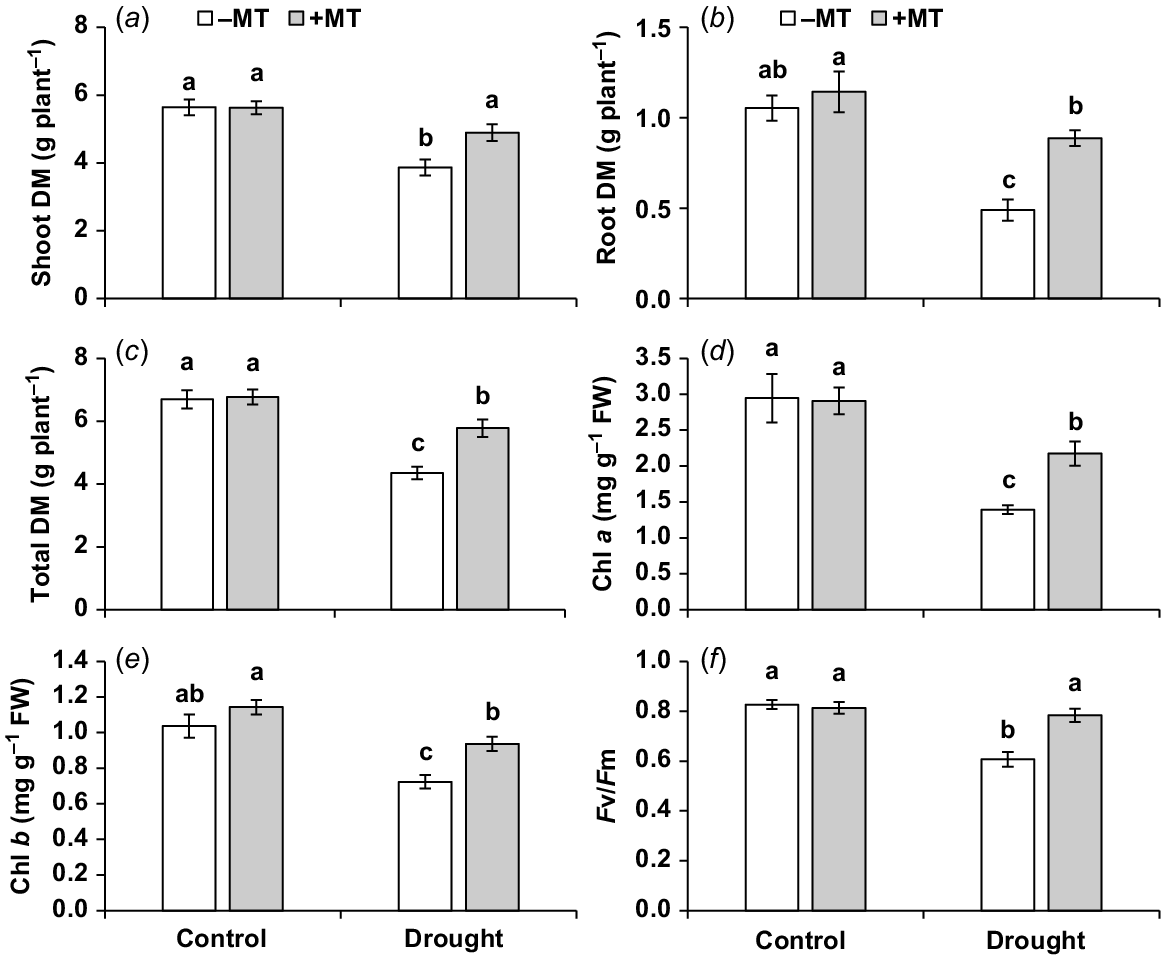
Drought stress significantly lowered leaf RWC and water potential (ΨI). MT significantly (P ≤ 0.05) enhanced leaf RWC and leaf ΨI by 33% and 80%, respectively (Fig. 2a, b). The control plants did not exhibit significant changes after pre-treatment with MT. Drought-affected plants shown wilting symptoms (Fig. 2c) that were not present in MT-treated plants.
Effect of melatonin (MT) on water-related characteristics of pepper plants exposed to drought conditions. (a) Leaf relative water content (RWC), (b) leaf water potential (Ψl) and (c) plant phenotype and leaf temperature. Plants were sprayed either with 0.10 mM melatonin (+MT) or water (mock control; −MT). Data is mean ± s.e. (n = 6–8). Tukey’s test was performed to determine the significant differences between groups and bars labelled with different lower case letters are statistically different at P ≤ 0.05. Digital and infra-red photographs were taken at harvest stage.
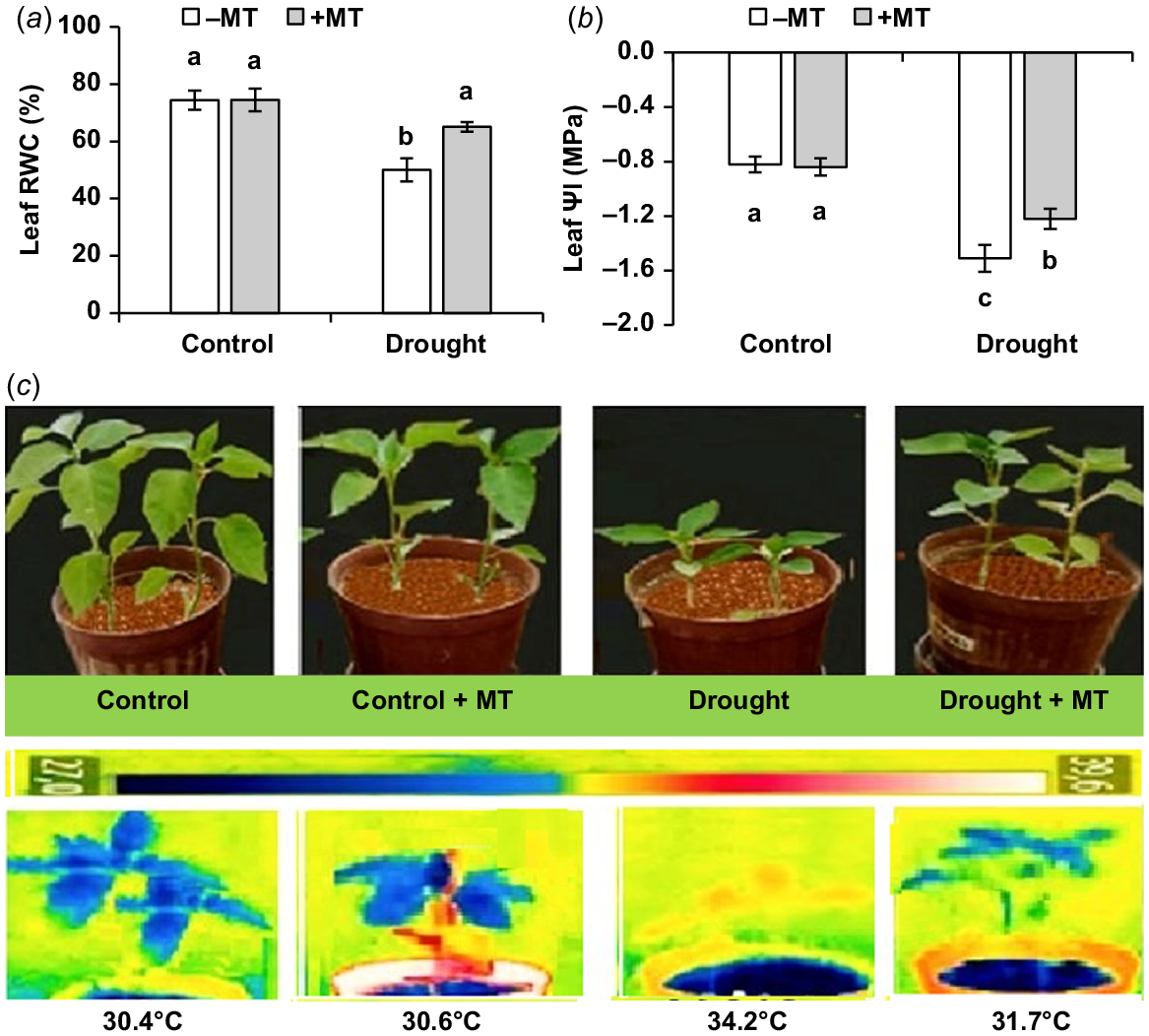
An infra-red image was used to measure the canopy temperatures of pepper plants receiving various treatments. The foliage temperatures were significantly raised by drought in comparison to the control treatment (30.4°C vs 34.2°C, respectively). MT application significantly lowered canopy temperature to 31.7°C if drought-stressed plants (Fig. 2c), suggesting better transpiration cooling.
Exogenous MT treatment improved synthesis of compatible solutes under drought stress
Proline, soluble sugar, glycine betaine and free amino acid contents were increased by 94%, 29%, 30% and 46%, respectively (Fig. 3a–d), but soluble protein content decreased by 45% compared to control plants when subjected to drought stress (Fig. 3e). Pre-treatment of MT further increased proline, glycine betaine, soluble sugar and soluble protein content by 22%, 16%, 9% and 39%, respectively. Thus, beneficial effects of exogenous MT application could be attributed to better osmotic adjustment by means of compatible solutions.
Effect of melatonin (MT) on biochemical characteristics of pepper plants exposed to drought condition. (a) Proline, (b) soluble sugar (SS), (c) glycine betaine (GB), (d) free amino acid (FAA) and (e) soluble protein (SP). Plants were sprayed either with 0.10 mM melatonin (+MT) or water (mock control; −MT). Data is mean ± s.e. (n = 6–8). Tukey’s test was performed to determine the significant differences between groups and bars labelled with different lower case letters are statistically different at P ≤ 0.05. FW, fresh weight; DW, dry weight.
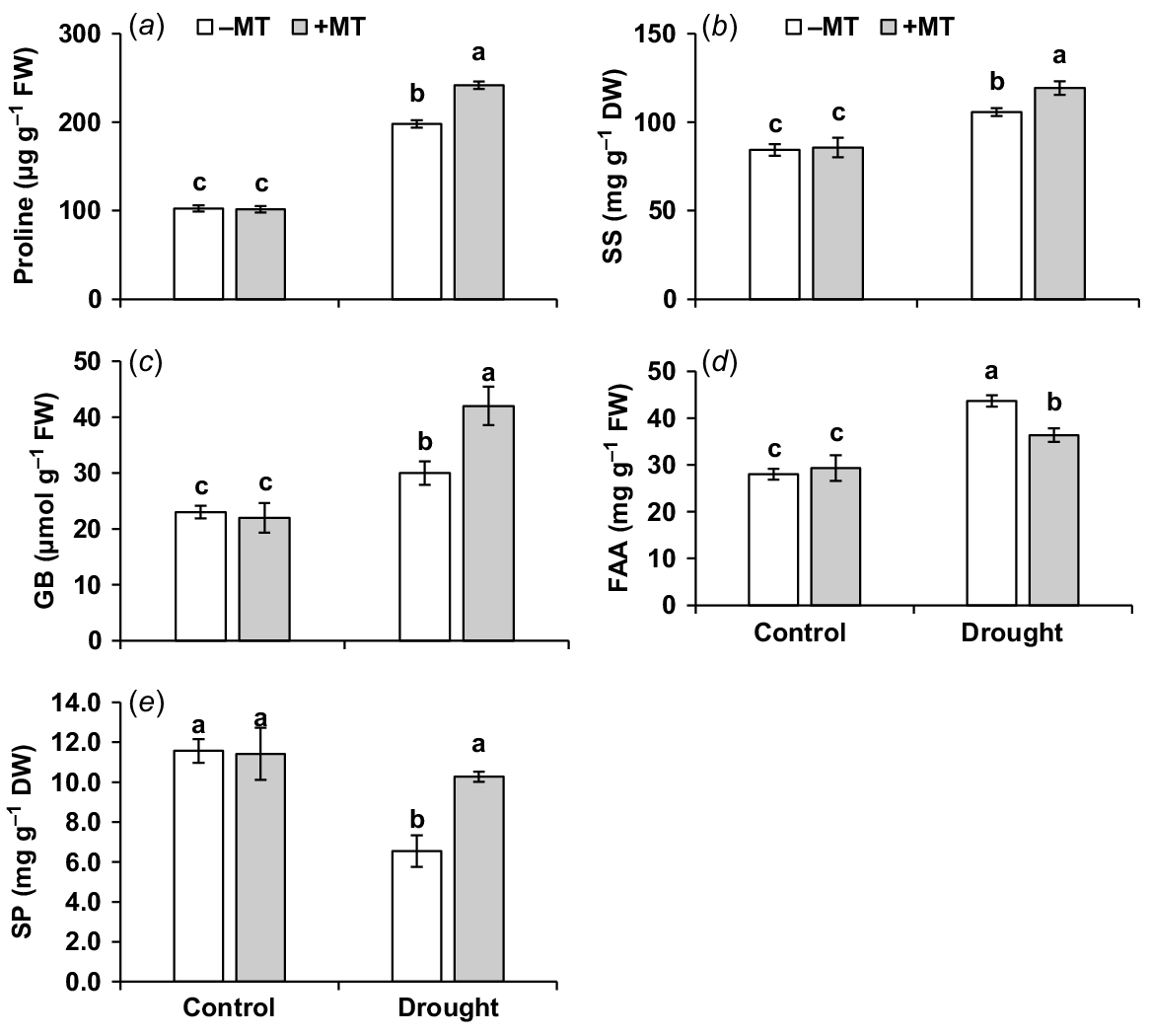
MT decreased oxidative stress and boosts antioxidant enzyme activity under drought stress
The oxidative stress experienced by plants under drought stress conditions (Bernardo et al. 2019) was assessed in this study, with a focus on the extent of oxidative damage and the possible mitigating effects of MT treatment. When compared to control plants, drought-stressed plants had higher levels of the oxidative stress markers, H2O2, MDA and EL ratio, which were 2.4, 3.4 and 1.7-fold higher, respectively. Priming with MT decreased these oxidative stress indicators by 32%, 25% and 30%, respectively (Fig. 4a–c). Exogenous application of MT was found to be beneficial in reducing drought-induced oxidative damage, lowering EL, improving membrane stability and promoting pepper plant growth under drought.
Effect of melatonin (MT) on redox characteristics of pepper plants exposed to drought conditions. (a) Hydrogen peroxide (H2O2), (b) malondialdehyde (MDA) and (c) electrolyte leakage (EL). Plants were sprayed either with 0.10 mM melatonin (+MT) or water (mock control; −MT). Data is mean ± s.e. (n = 6–8). Tukey’s test was performed to determine the significant differences between groups and bars labelled with different lower case letters are statistically different at P ≤ 0.05. FW, fresh weight.
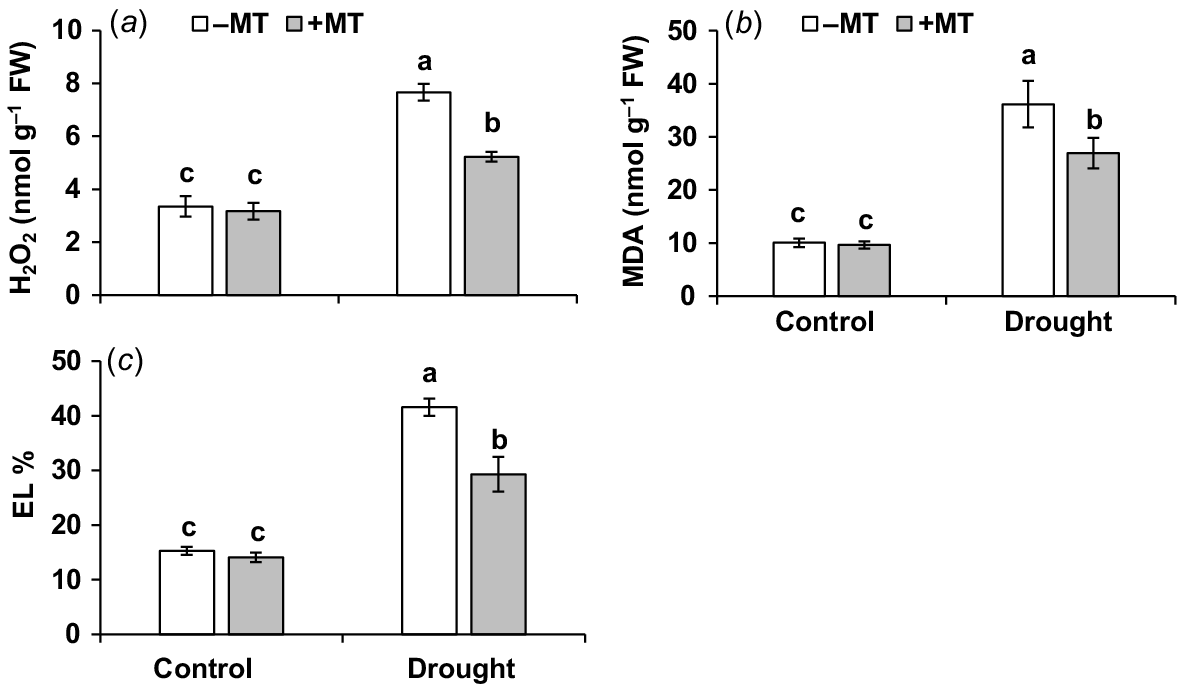
The activities of GST, CAT and POD were measured to confirm the alleviating effect of MT on oxidative detoxification in drought-stressed pepper plants. In drought-affected plants, activities of GST, CAT and POD was elevated by 71%, 75% and 131%, respectively, when compared to those in control plants (Fig. 5a–c). The activities of these enzymes were enhanced further after treatment with MT in drought-stressed plants. These findings suggested that the use of MT might strengthen antioxidant defence in plants subjected to drought treatment.
Effect of melatonin (MT) on antioxidant activity of pepper plants exposed to drought conditions. (a) Glutathione S-transferase (GST), (b) catalase (CAT), (c) peroxidase (POD), (d) methylglyoxal (MG), (e) glyoxalase I (Gly I) and (f) glyoxalase II (Gly II). Plants were sprayed either with 0.10 mM melatonin (+MT) or water (mock control; −MT). Data is mean ± s.e. (n = 6–8). Tukey’s test was performed to determine the significant differences between groups and bars labelled with different lower case letters are statistically different at P ≤ 0.05.
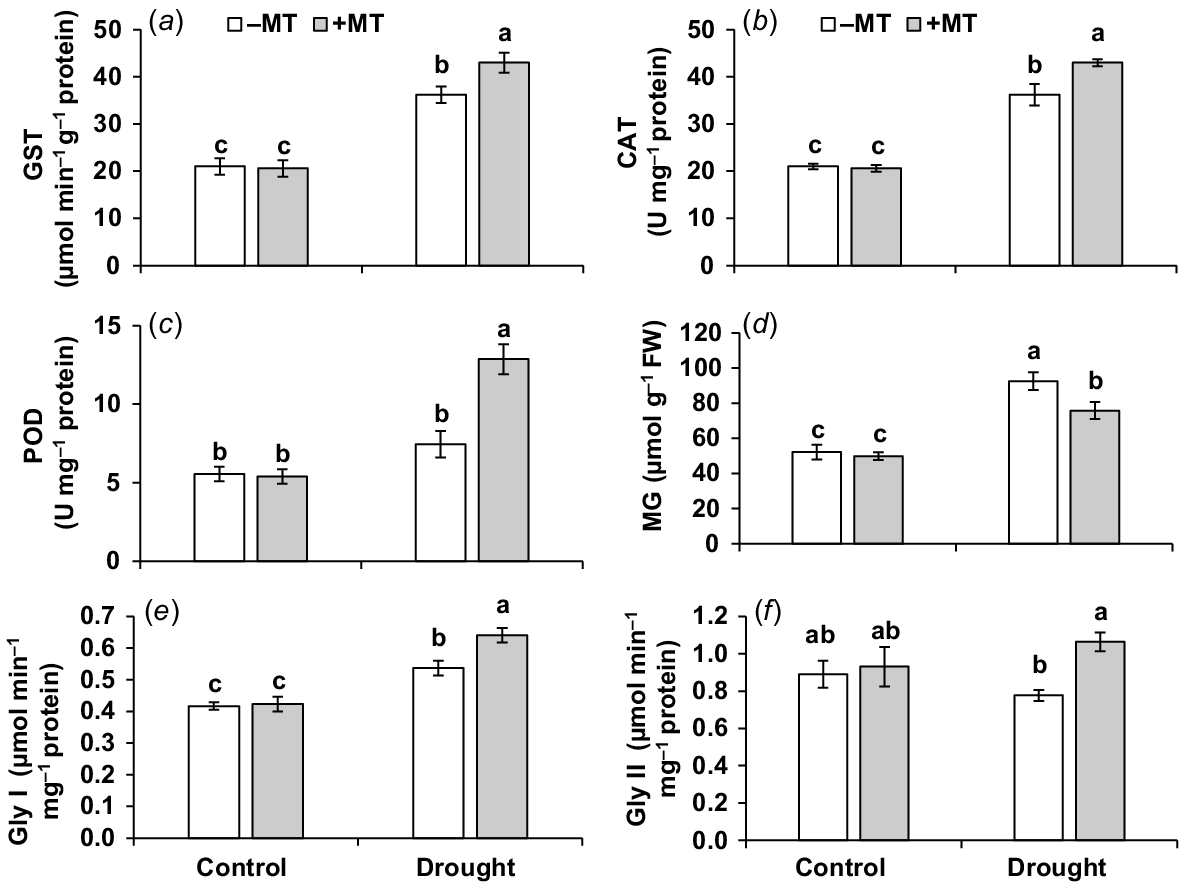
MT increased the glyoxalase system enzyme activities and decreases the amount of methylglyoxal under drought
In response to drought, the content of methylglyoxal (MG) increased by 80% (Fig. 5d). When drought-stressed plants were compared to control plants, there was a substantial increase (29%) in Gly I but a slight decrease in Gly II (12%) (Fig. 5e, f). The activities of Gly I and Gly II in drought-stressed plants pre-treated with MT were considerably higher, but MG levels were lower than in plants treated with MT alone under drought. This shows that MT effectively increases drought tolerance by activating the glyoxalase system and reducing MG concentration.
MT modulated nitrogen metabolism under drought
Nitrogen metabolism-related enzyme activities, as well as nitrite, ammonium and total nitrogen content, were measured in the plant to see if MT-upregulation of nitrogen metabolism-related parameters improves pepper plant tolerance to drought stress. During drought treatment, the activities of enzymes related to nitrogen metabolism, including NR, NiR, GS, GOGAT and GDH, were significantly reduced (Fig. 6a–e). Plants supplemented with MT had 20%, 22%, 24%, 29% and 30% higher NR, NiR, GS, GOGAT and GDH levels, respectively, than those in drought-stressed plants.
Effect of melatonin (MT) on activity of enzymes related to nitrogen metabolism in pepper plants exposed to drought conditions. (a) Nitrate reductase (NR), (b) nitrit reductase (NiR), (c) glutamine synthetase (GS), (d) glutamate synthase (GOGAT) and (e) glutamate dehydrogenase (GDH). Plants were sprayed either with 0.10 mM melatonin (+MT) or water (mock control; −MT). Data is mean ± s.e. (n = 6–8). Tukey’s test was performed to determine the significant differences between groups and bars labelled with different lower case letters are statistically different at P ≤ 0.05. FW, fresh weight.
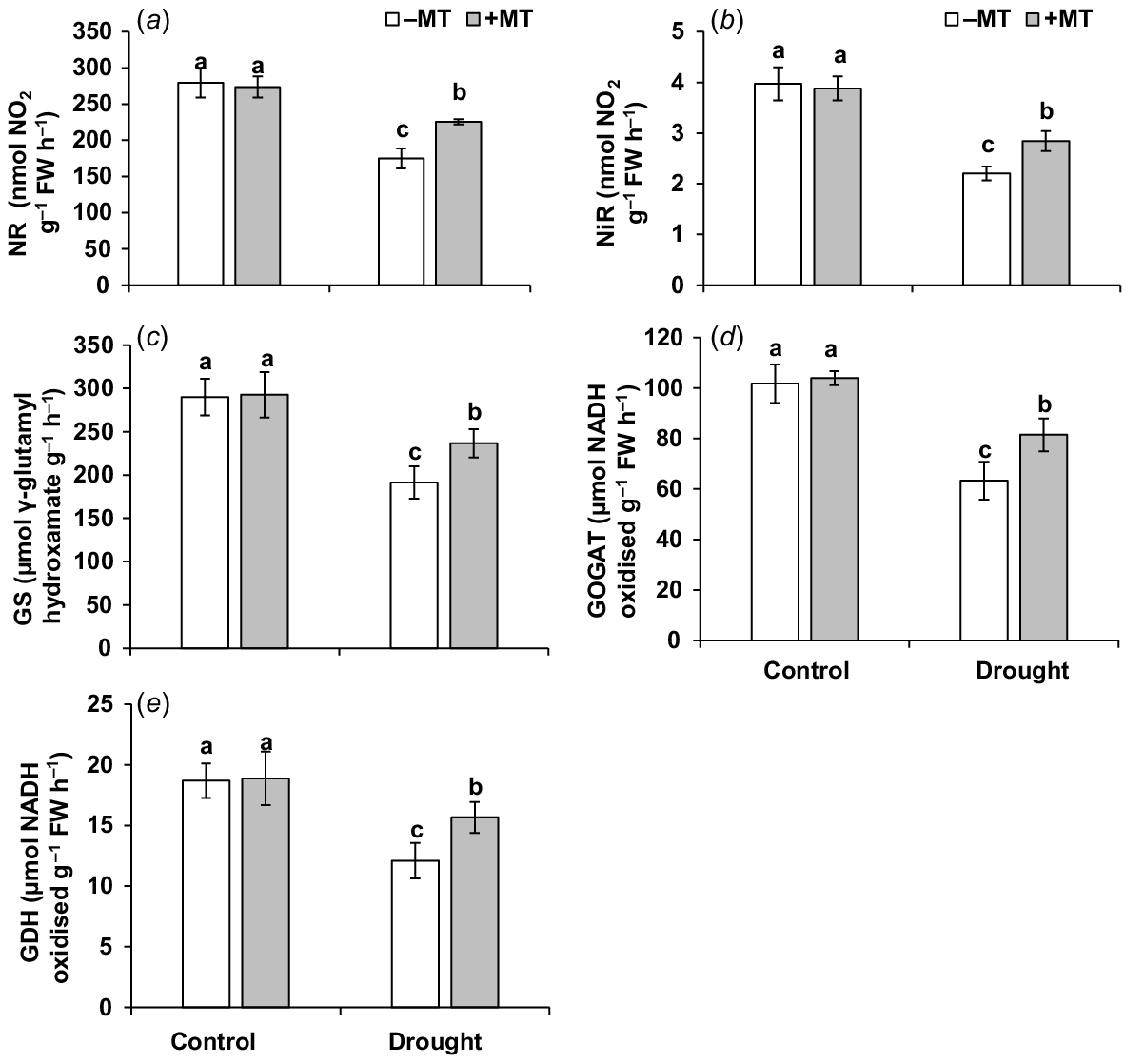
Drought stress significantly (P ≤ 0.05) elevated ammonium (NH4+)-N content by 51% and 59%, and nitrate (NO3−)-N by 39% and 31% but reduced total nitrogen (N) content by 36% and 35% in the leaves and roots, respectively, compared to those in control plants (Fig. 7a–f). MT reduced NH4+ contents by 18% and 25%, and NO3− content by 18% and 10%, but increased N content by 22% and 20% in leaves and roots, respectively, in those of drought-stressed plants alone. This demonstrates that MT in the presence of drought mitigated an increase in NO3− and NH4+ contents (thus reducing their potential toxicity to cell metabolism) while increasing total N content in plants.
Effect of melatonin (MT) on nitrogen content and profile in pepper plants exposed to drought conditions. (a) Leaf ammonium (NH4+), (b) root ammonium (NH4+), (c) leaf nitrate (NO3−), (d) root nitrate (NO3−), (e) leaf total nitrogen and (f) root total nitrogen. Plants were sprayed either with 0.10 mM melatonin (+MT) or water (mock control; −MT). Data is mean ± s.e. (n = 6–8). Tukey’s test was performed to determine the significant differences between groups and bars labelled with different lower case letters are statistically different at P ≤ 0.05. FW, fresh weight; DW, dry weight.
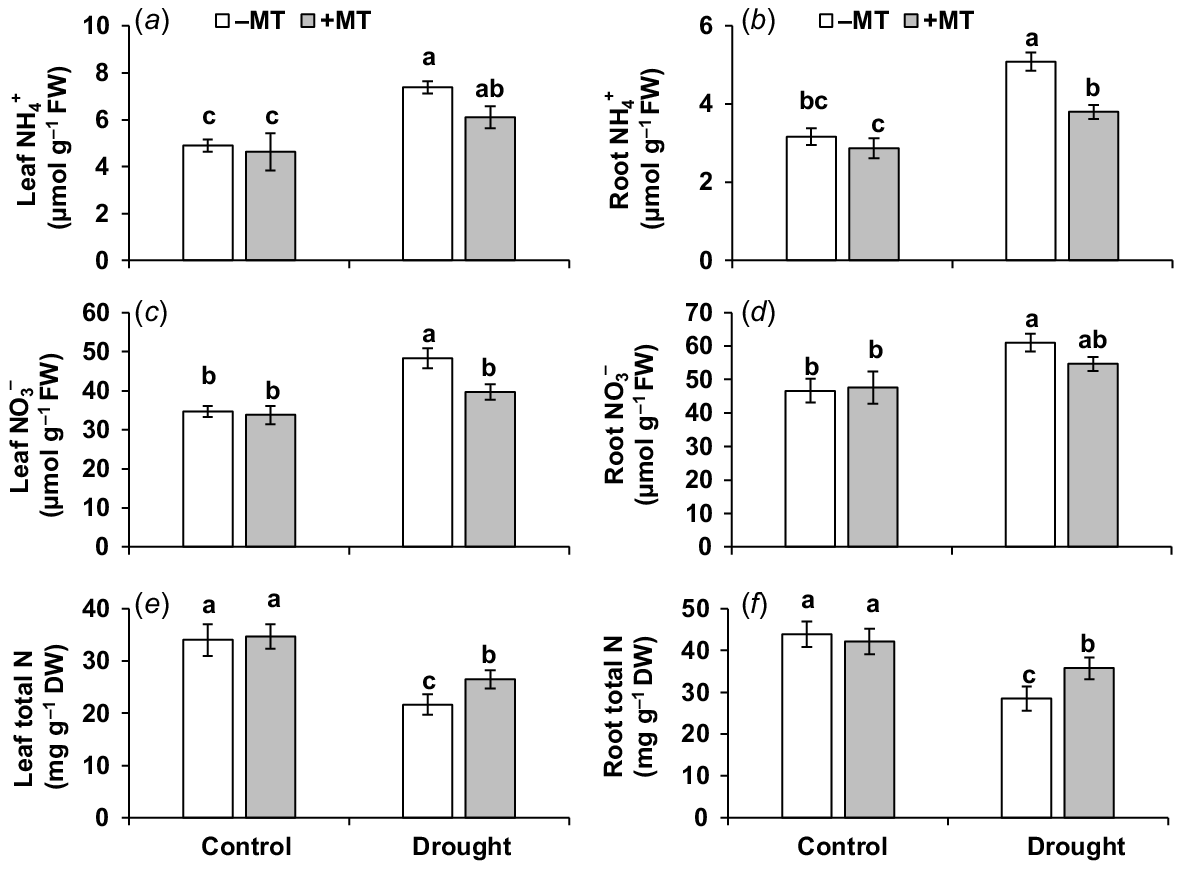
Discussion
MT improved plant growth and water relation under drought
Drought stress has been shown to have a harmful effect on plant performance (Khan et al. 2019) including plant growth (Al-Huqail et al. 2020), leaf water content (Ichsan et al. 2020) and redox characteristics (Bernardo et al. 2019). Osmolytes, such as proline, glycine betaine and soluble sugars, are produced by plants due to drought stress to maintain high osmotic pressure and water potential within the cells, maintaining the integrity of the cell membranes and enhancing drought resistance in crops (Du et al. 2020a). In the present study, plants experiencing drought stress had lower RWC and leaf water potential (Ψl). Soluble sugars, proline and glycine betaine levels were all increased to maintain the water status of plant under drought conditions. The accumulation and synthesis of soluble compounds, such as amino acids, particularly proline and soluble sugars, control osmoregulation during drought (Castañeda and González 2021). The results of current study showed that both free amino acid and proline concentrations increased in response to drought, which is in agreement with prior research conducted by Du et al. (2020b) and Cao et al. (2022). This finding supports the notion that increased accumulation of free amino acids and proline is a common response of plants to water deficit conditions. Proline also protects plant cells from oxidative damage and effectively neutralises ROS in addition to acting as an osmoprotectant (Ould said et al. 2021). Thus, MT-mediated proline synthesis could represent an additional mechanism by which plants enhance their drought tolerance.
Canopy temperature is a critical indicator of crop water potential and is used to plan irrigation for a variety of crops (Virnodkar et al. 2020). Drought treatment increased the canopy temperature from 30.4°C to 34.2°C in the present experiment. Drought stress causes stomata to close quickly, transpiration to decrease and leaf temperature to rise (Gräf et al. 2021). High canopy temperatures inhibit plant growth and can reduce biomass and yield (Sofi et al. 2019). The treatment of MT reduced the canopy temperature to the level comparable to the control treatment. This shows that MT-improved plant growth might be due to lower canopy temperatures.
MT improves traits related to photosynthesis under drought
The maximum photochemical efficiency (Fv/Fm) and chlorophyll content were both negatively impacted by drought stress; similar findings that drought-induced decreases in those attributes have also been observed in coffee (Coffea arabica) (de Souza et al. 2020) and rice (Oryza sativa) plants (Xia et al. 2022). Due to overproduction of ROS and altered cellular enzyme activity in our experiment, the amount of chlorophyll decreased under drought. Decreased chlorophyll content could be due to the lower N metabolism in drought-affected plants, given that chlorophyll levels are often used as a proxy for leaf N status (Zahoor et al. 2017; Xia et al. 2020). One more explanation for the decrease in chlorophyll concentration could be due to either a faster rate of breakdown of the chlorophyll or a drop in the enzyme’s activity required for chlorophyll synthesis (Altuntaş et al. 2020). Moreover, enhanced traits related to photosynthesis and MT-induced decreased H2O2 indicate that MT helps to lessen the negative impacts of drought on photosynthetic traits, possibly by slowing the build-up of H2O2, similar to what was reported in rice (Silalert and Pattanagul 2021). MT has also been noted to elevate the chlorophyll synthesis in coffee plants (Campos et al. 2019) and tomatoes (Ibrahim et al. 2020) under drought.
MT alleviated oxidative stress and upregulates the antioxidant defence system under drought
Drought stress induces oxidative stress by interfering with electron transport throughout the photosynthetic process (Dalal and Tripathy 2018). MDA and H2O2 are well-known oxidative damage metrics and both were elevated under drought in the current study, indicating increased oxidative stress in drought-treated plants (Iqbal et al. 2018; Sohag et al. 2020).
Antioxidant defence systems are built by plants to regulate ROS build-up within cells (Guidi and Tattini 2021). In our studies, GST was upregulated. GST has the potential to detoxify H2O2 by employing the reagent glutathione (Majeed et al. 2018). CAT and POD levels also increased (Fig 5b, c). MT pre-treatments alleviated oxidative stress in drought-treated plants, as indicated by lower H2O2 and MDA levels. Additional elevations in GST were achieved with the pre-treatment of MT under drought, showing that GST plays a positive function in detoxifying H2O2. This confirms the role of MT as an inducer of antioxidant enzymatic activity and eliminating ROS. Melatonin is a multifunctional molecule that is known as an all-purpose antioxidant per se (Yan et al. 2021; Huang et al. 2022).
MT-upregulated glyoxalase system under drought
The glyoxalase system contains enzymes Gly I and Gly II to scavenge excess methylglyoxal (MG) build-up under harsh conditions (Li 2019; Altaf et al. 2022b). MG build-up reportedly increased in rapeseed (Brassica napus) (Hasanuzzaman et al. 2017) and soybean (Glycine max) (Hasan et al. 2020) under drought stress conditions. In pepper plants, water stress increased Gly I activity while lowered Gly II activity. MT treatment decreased the buildup of MG and improved the activity of both enzymes, demonstrating the contribution of MT to drought tolerance through boosting the glyoxalase system. MT-downregulated MG synthesis has been reported in potatoes (Solanum tuberosum) under drought stress (El-Yazied et al. 2022). The process of glycolysis in cells results in the production of methylglycoxal (MG), a newly discovered signalling molecule involved in the responses and tolerance of plants to abiotic stress (Li 2019).
MT improved nitrogen metabolism under drought
Drought-induced physiological abnormalities unavoidably influence the synthesis, breakdown and metabolism of nitrogenous substances (Cao et al. 2022). Overall, this may result in a shortage of the substrate needed by enzymes involved in nitrogen metabolism, which could explain why those enzyme activities declined in this study under drought conditions. The ability of a plant to assimilate nitrogen, produce proteins and engage in overall N metabolism is all dependent on the activities of nitrogen metabolism-related key enzymes (Wen et al. 2019). Consequently, the reactions of these crucial enzymes are crucial for plant growth and development (Gaudinier et al. 2018; Rizwan et al. 2022). Data obtained in this study confirmed that under drought, the NR, NiR, GS, GOGAT and GDH activities of pepper leaves considerably decreased (Fig 6a–e). Reduced activities of these enzymes due to drought may eventually lead to decreased protein synthesis, most likely due to reduced assimilation of N. Because of a lack of substrates, a chain of events occurs, resulting in a decrease in the activity of specific enzymes involved in N metabolism. Under stressful conditions, it has been shown that plant biostimulators increase the activation of essential N metabolism enzymes and increase soybean stress tolerance via modifying N metabolism to delay the rate of leaf ageing (Zhang et al. 2020). The activities of nitrogen metabolism-related enzymes significantly increased when exogenous melatonin (MT) was applied to pepper plants under drought conditions in this study. These findings are consistent with previous research, which has shown that exogenous MT can help to maintain a consistent level of nitrogen assimilation by upregulating significant enzyme activities and offsetting the negative effects of drought on these essential enzyme activities in nitrogen metabolism (Cao et al. 2022). Inorganic N forms such as NH4+ and NO3− are the primary N sources for crop plants (Gruffman et al. 2014). For crops, ammonium is a preferred source of nitrogen because it requires less energy to metabolise. However, high NH4+ concentrations can damage the plant’s cells and induce N metabolism dysfunction (Dubey et al. 2021). Limiting the overabundance of NH4+ in plant tissues is regarded as a crucial ability to tolerate drought stress (Zou et al. 2021). In our study, drought resulted in a substantial rise in both NH4+ and NO3−-N contents, but reduced nitrogen content in the roots and leaves of pepper plants, as previously reported in soybean plants (Cao et al. 2022). Decreased NR and NiR activity, as also observed in soybean (Du et al. 2020a), is most likely the cause of leaf NO3− accumulation under drought conditions. NO3− in leaves altered guard cell depolarisation, thereby causing stomatal closing, which leads to decreased photosynthesis activity and, as a result, reduced plant growth (Schäfer et al. 2018). One of possible reasons of increased canopy temperature caused by drought could be high NO3− accumulation within the leaf cell, which causes stomatal closure, reducing transpiration and increasing canopy temperature, as observed in our study.
Exogenous MT may increase the activity of critical N metabolism enzymes while decreasing NO3− and NH4+ concentrations, all of which lead to an increase in protein assimilation. Exogenous MT increased NR and NiR activity in the presence of drought, lowering NO3− concentration. The NR and NiR collaborate to convert the NO3− taken up by the plant into NH4+ (Yoneyama and Suzuki 2019). Reduced NH4+ in the plant tissues due to exogenous MT under drought could be attributed to increase in the activity of the GS and GOGAT enzymes, which convert NH4+ to glutamine and glutamate, respectively (Wang et al. 2020).
Conclusions
Our study demonstrates that the application of melatonin can stimulate the antioxidant defence system, lower ROS levels, enhance RWC and upregulate nitrogen metabolism in sweet pepper plants under water-limited conditions. These findings suggest that exogenous melatonin application can serve as a sustainable and cost-effective solution to reduce crop losses and improve potential yields in water-limited conditions. Our study also underscores the need for further investigation into the molecular processes underlying melatonin action and its impact on nutrient absorption to improve plant tolerance and adaptation to drought. The development of low cost and eco-friendly technologies like melatonin application is crucial in the context of global warming and our findings provide new insights into the potential of melatonin in crop production.
Acknowledgements
The authors are grateful to the University of Harran (Turkey) for supporting this study.
References
Agbaria H, Heuer B, Zieslin N (1998) Rootstock-imposed alterations in nitrate reductase and glutamine synthetase activities in leaves of rose plants. Biologia plantarum 41, 85-91.
| Crossref | Google Scholar |
Ahluwalia O, Singh PC, Bhatia R (2021) A review on drought stress in plants: implications, mitigation and the role of plant growth promoting rhizobacteria. Resources, Environment and Sustainability 5, 100032.
| Crossref | Google Scholar |
Ahmad S, Wang G-Y, Muhammad I, Chi Y-X, Zeeshan M, Nasar J, Zhou X-B (2022) Interactive effects of melatonin and nitrogen improve drought tolerance of maize seedlings by regulating growth and physiochemical attributes. Antioxidants 11(2), 359.
| Crossref | Google Scholar |
Al-Huqail A, El-Dakak RM, Sanad MN, Badr RH, Ibrahim MM, Soliman D, Khan F (2020) Effects of climate temperature and water stress on plant growth and accumulation of antioxidant compounds in sweet basil (Ocimum basilicum L.) leafy vegetable. Scientifica 2020, 3808909.
| Crossref | Google Scholar |
Altaf MA, Shahid R, Ren M-X, Naz S, Altaf MM, Khan LU, Tiwari RK, Lal MK, Shahid MA, Kumar R, Nawaz MA, Jahan MS, Jan BL, Ahmad P (2022a) Melatonin improves drought stress tolerance of tomato by modulating plant growth, root architecture, photosynthesis, and antioxidant defense system. Antioxidants 11(2), 309.
| Crossref | Google Scholar |
Altaf MM, Diao X-P, Wang H, Khan LU, Rehman Au, Shakoor A, Altaf MA, Farooq TH (2022b) Salicylic acid induces vanadium stress tolerance in rice by regulating the AsA-GSH cycle and glyoxalase system. Journal of Soil Science and Plant Nutrition 22, 1983-1999.
| Crossref | Google Scholar |
Altuntaş C, Demiralay M, Sezgin Muslu A, Terzi R (2020) Proline-stimulated signaling primarily targets the chlorophyll degradation pathway and photosynthesis associated processes to cope with short-term water deficit in maize. Photosynthesis Research 144(1), 35-48.
| Crossref | Google Scholar |
Bates LS, Waldren RP, Teare ID (1973) Rapid determination of free proline for water-stress studies. Plant and Soil 39, 205-207.
| Crossref | Google Scholar |
Bernardo L, Carletti P, Badeck FW, Rizza F, Morcia C, Ghizzoni R, Rouphael Y, Colla G, Terzi V, Lucini L (2019) Metabolomic responses triggered by arbuscular mycorrhiza enhance tolerance to water stress in wheat cultivars. Plant Physiology and Biochemistry 137, 203-212.
| Crossref | Google Scholar |
Bonfim-Silva EM, de Anicésio ECA, de Oliveira JR, de Freitas Sousa HH, da Silva TJA (2015) Soil water availabilities in the content and accumulation of nitrogen and chlorophyll index in the safflower. American Journal of Plant Sciences 6, 2280-2286.
| Crossref | Google Scholar |
Campos CN, Ávila RG, de Souza KRD, Azevedo LM, Alves JD (2019) Melatonin reduces oxidative stress and promotes drought tolerance in young Coffea arabica L. plants. Agricultural Water Management 211, 37-47.
| Crossref | Google Scholar |
Cao L, Qin B, Gong Z, Zhang Y (2022) Melatonin improves nitrogen metabolism during grain filling under drought stress. Physiology and Molecular Biology of Plants 28, 1477-1488.
| Crossref | Google Scholar |
Castañeda V, González EM (2021) Strategies to apply water-deficit stress: similarities and disparities at the whole plant metabolism level in Medicago truncatula. International Journal of Molecular Sciences 22(6), 2813.
| Crossref | Google Scholar |
Cataldo DA, Maroon M, Schrader LE, Youngs VL (1975) Rapid colorimetric determination of nitrate in plant tissue by nitration of salicylic acid. Communications in Soil Science and Plant Analysis 6(1), 71-80.
| Crossref | Google Scholar |
Cañas RA, Yesbergenova-Cuny Z, Belanger L, Rouster J, Brulé L, Gilard F, Quillere I, Sallaud C, Hirel B (2020) NADH-GOGAT overexpression does not improve maize (Zea mays L.) performance even when pyramiding with NAD-IDH, GDH and GS. Plants 9(2), 130.
| Crossref | Google Scholar |
Chakravarty TN, Sopory SK (1998) Blue light stimulation of cell proliferation and glyoxalase I activity in callus cultures of Amaranthus paniculatus. Plant Science 132, 63-69.
| Crossref | Google Scholar |
Chance B, Maehly AC (1955) Assay of catalase and peroxidases. Methods in Enzymology 2, 764-775.
| Crossref | Google Scholar |
Dalal VK, Tripathy BC (2018) Water-stress induced downsizing of light-harvesting antenna complex protects developing rice seedlings from photo-oxidative damage. Scientific Reports 8(1), 5955.
| Crossref | Google Scholar |
da Silva Leite R, do Nascimento MN, Tanan TT, Gonçalves Neto LP, da Silva Ramos CA, da Silva AL (2019) Alleviation of water deficit in Physalis angulata plants by nitric oxide exogenous donor. Agricultural Water Management 216, 98-104.
| Crossref | Google Scholar |
de Souza BP, Martinez HEP, de Carvalho FP, Loureiro ME, Sturião WP (2020) Gas exchanges and chlorophyll fluorescence of young coffee plants submitted to water and nitrogen stresses. Journal of Plant Nutrition 43(16), 2455-2465.
| Crossref | Google Scholar |
Debouba M, Gouia H, Suzuki A, Ghorbel MH (2006) NaCl stress effects on enzymes involved in nitrogen assimilation pathway in tomato “Lycopersicon esculentum” seedlings. Journal of Plant Physiology 163(12), 1247-1258.
| Crossref | Google Scholar |
Dionisio-Sese ML, Tobita S (1998) Antioxidant responses of rice seedlings to salinity stress. Plant Science 135, 1-9.
| Crossref | Google Scholar |
Dorion S, Ouellet JC, Rivoal J (2021) Glutathione metabolism in plants under stress: beyond reactive oxygen species detoxification. Metabolites 11(9), 641.
| Crossref | Google Scholar |
Du Y, Zhao Q, Chen L, Yao X, Xie F (2020a) Effect of drought stress at reproductive stages on growth and nitrogen metabolism in soybean. Agronomy 10, 302.
| Crossref | Google Scholar |
Du Y, Zhao Q, Chen L, Yao X, Zhang W, Zhang B, Xie F (2020b) Effect of drought stress on sugar metabolism in leaves and roots of soybean seedlings. Plant Physiology and Biochemistry 146, 1-12.
| Crossref | Google Scholar |
El-Yazied AA, Ibrahim MFM, Ibrahim MAR, Nasef IN, Al-Qahtani SM, Al-Harbi NA, Alzuaibr FM, Alaklabi A, Dessoky ES, Alabdallah NM, Omar MMA, Ibrahim MTS, Metwally AA, Hassan KM, Shehata SA (2022) Melatonin mitigates drought induced oxidative stress in potato plants through modulation of osmolytes, sugar metabolism, ABA homeostasis and antioxidant enzymes. Plants 11(9), 1151.
| Crossref | Google Scholar |
Fales FW (1951) The assimilation and degradation of carbohydrates by yeast cells. Journal of Biological Chemistry 193, 113-124.
| Crossref | Google Scholar |
Gao H, Huang L, Gong Z, Wang X, Qiao X, Xiao F, Yang Y, Yu B, Guo X, Yu C, Zhang H (2022) Exogenous melatonin application improves resistance to high manganese stress through regulating reactive oxygen species scavenging and ion homeostasis in tobacco. Plant Growth Regulation 98, 219-233.
| Crossref | Google Scholar |
Gaudinier A, Rodriguez-Medina J, Zhang L, Olson A, Liseron-Monfils C, Bågman A-M, Foret J, Abbitt S, Tang M, Li B, Runcie DE, Kliebenstein DJ, Shen B, Frank MJ, Ware D, Brady SM (2018) Transcriptional regulation of nitrogen-associated metabolism and growth. Nature 563, 259-264.
| Crossref | Google Scholar |
Gräf M, Immitzer M, Hietz P, Stangl R (2021) Water-stressed plants do not cool: leaf surface temperature of living wall plants under drought stress. Sustainability 13(7), 3910.
| Crossref | Google Scholar |
Grieve CM, Grattan SR (1983) Rapid assay for determination of water soluble quaternary ammonium compounds. Plant and Soil 70, 303-307.
| Crossref | Google Scholar |
Groat RG, Vance CP (1981) Root nodule enzymes of ammonia assimilation in alfalfa (Medicago sativa L.) developmental patterns and response to applied nitrogen. Plant Physiology 67(6), 1198-1203.
| Crossref | Google Scholar |
Gruffman L, Jämtgård S, Näsholm T (2014) Plant nitrogen status and co-occurrence of organic and inorganic nitrogen sources influence root uptake by Scots pine seedlings. Tree Physiology 34(2), 205-213.
| Crossref | Google Scholar |
Guidi L, Tattini M (2021) Antioxidant defenses in plants: a dated topic of current interest. Antioxidants 10(6), 855.
| Crossref | Google Scholar |
Hasan MM, Ali MA, Soliman MH, Alqarawi AA, Abd_Allah EF, Fang XW (2020) Insights into 28-homobrassinolide (HBR)-mediated redox homeostasis, AsA–GSH cycle, and methylglyoxal detoxification in soybean under drought-induced oxidative stress. Journal of Plant Interactions 15(1), 371-385.
| Crossref | Google Scholar |
Hasanuzzaman M, Nahar K, Hossain MS, Anee TI, Parvin K, Fujita M (2017) Nitric oxide pretreatment enhances antioxidant defense and glyoxalase systems to confer PEG-induced oxidative stress in rapeseed. Journal of Plant Interactions 12, 323-331.
| Crossref | Google Scholar |
He J, Hu W, Li Y, Zhu H, Zou J, Wang Y, Meng Y, Chen B, Zhao W, Wang S, Zhou Z (2022) Prolonged drought affects the interaction of carbon and nitrogen metabolism in root and shoot of cotton. Environmental and Experimental Botany 197, 104839.
| Crossref | Google Scholar |
Hu W, Zhang J, Yan K, Zhou Z, Zhao W, Zhang X, Pu Y, Yu R (2021) Beneficial effects of abscisic acid and melatonin in overcoming drought stress in cotton (Gossypium hirsutum L.). Physiologia Plantarum 173(4), 2041-2054.
| Crossref | Google Scholar |
Huang Z, Liu X, Sun S, Tang Y, Yuan X, Tang Q (2021) Global assessment of future sectoral water scarcity under adaptive inner-basin water allocation measures. Science of The Total Environment 783, 146973.
| Crossref | Google Scholar |
Huang X, Tanveer M, Min Y, Shabala S (2022) Melatonin as a regulator of plant ionic homeostasis: implications for abiotic stress tolerance. Journal of Experimental Botany 73(17), 5886-5902.
| Crossref | Google Scholar |
Ibrahim MFM, Elbar OHA, Farag R, Hikal M, El-Kelish A, El-Yazied AA, Alkahtani J, El-Gawad HGA (2020) Melatonin counteracts drought induced oxidative damage and stimulates growth, productivity and fruit quality properties of tomato plants. Plants 9(10), 1276.
| Crossref | Google Scholar |
Ichsan CN, Basyah B, Zakaria S, Efendi E (2020) Differences of water status and relationship with roots growth and yield of rice under water stress. Systematic Reviews in Pharmacy 11(8), 611-618.
| Google Scholar |
Iqbal H, Yaning C, Waqas M, Shareef M, Raza ST (2018) Differential response of quinoa genotypes to drought and foliage-applied H2O2 in relation to oxidative damage, osmotic adjustment and antioxidant capacity. Ecotoxicology and Environmental Safety 164, 344-354.
| Crossref | Google Scholar |
Kaya C, Ugurlar F, Ashraf M, Alyemeni MN, Bajguz A, Ahmad P (2022) The involvement of hydrogen sulphide in melatonin-induced tolerance to arsenic toxicity in pepper (Capsicum annuum L.) plants by regulating sequestration and subcellular distribution of arsenic, and antioxidant defense system. Chemosphere 309, 136678.
| Crossref | Google Scholar |
Kerchev PI, Van Breusegem F (2022) Improving oxidative stress resilience in plants. The Plant Journal 109(2), 359-372.
| Crossref | Google Scholar |
Khan N, Bano A, Babar MA (2019) Metabolic and physiological changes induced by plant growth regulators and plant growth promoting rhizobacteria and their impact on drought tolerance in Cicer arietinum L. PLoS ONE 14(3), e0213040.
| Crossref | Google Scholar |
Li Z-G (2019) Methylglyoxal: a novel signaling molecule in plant responses to abiotic stresses. In ‘Plant signaling molecules’. (Eds Khan MIR, Reddy PS, Ferrante A, Khan NA) pp. 219–233. (Woodhead Publishing: Sawston, UK) https//doi.org/10.1016/B978-0-12-816451-8.00013-7
Li G, Li Y, Zhu Y, Zheng W, Li M, Hu J, Zhu S (2023) Exogenous application of melatonin to mitigate drought stress-induced oxidative damage in Phoebe sheareri seedlings. PeerJ 11, e15159.
| Crossref | Google Scholar |
Liu Q, Zhang S, Zhang H, Bai Y, Zhang J (2020) Monitoring drought using composite drought indices based on remote sensing. Science of The Total Environment 711, 134585.
| Crossref | Google Scholar |
Liu L, Li D, Ma Y, Shen H, Zhao S, Wang Y (2021) Combined application of arbuscular mycorrhizal fungi and exogenous melatonin alleviates drought stress and improves plant growth in tobacco seedlings. Journal of Plant Growth Regulation 40(3), 1074-1087.
| Crossref | Google Scholar |
Majeed S, Nawaz F, Naeem M, Ashraf MY (2018) Effect of exogenous nitric oxide on sulfur and nitrate assimilation pathway enzymes in maize (Zea mays L.) under drought stress. Acta Physiologiae Plantarum 40(12), 206.
| Crossref | Google Scholar |
Molins-Legua C, Meseguer-Lloret S, Moliner-Martinez Y, Campíns-Falcó P (2006) A guide for selecting the most appropriate method for ammonium determination in water analysis. TrAC Trends in Analytical Chemistry 25(3), 282-290.
| Crossref | Google Scholar |
Nawaz K, Chaudhary R, Sarwar A, Ahmad B, Gul A, Hano C, Abbasi BH, Anjum S (2021) Melatonin as master regulator in plant growth, development and stress alleviator for sustainable agricultural production: current status and future perspectives. Sustainability 13(1), 294.
| Crossref | Google Scholar |
Ould said C, Boulahia K, Eid MAM, Rady MM, Djebbar R, Abrous-Belbachir O (2021) Exogenously used proline offers potent antioxidative and osmoprotective strategies to re-balance growth and physio-biochemical attributes in herbicide-stressed Trigonella foenum-graecum. Journal of Soil Science and Plant Nutrition 21(4), 3254-3268.
| Crossref | Google Scholar |
Ozturk M, Turkyilmaz Unal B, García-Caparrós P, Khursheed A, Gul A, Hasanuzzaman M (2021) Osmoregulation and its actions during the drought stress in plants. Physiologia Plantarum 172(2), 1321-1335.
| Crossref | Google Scholar |
Principato GB, Rosi G, Talesa V, Giovanni E, Uotila L (1987) Purification and characterization of two forms of glyoxalase II from the liver and brain of wistar rats. Biochimica et Biophysica Acta (BBA) – Protein Structure and Molecular Enzymology 911, 349-355.
| Crossref | Google Scholar |
Rawat S, Ganapathy A, Agarwal A (2022) Drought characterization over Indian sub-continent using GRACE-based indices. Scientific Reports 12(1), 15432.
| Crossref | Google Scholar |
Ren J, Yang X, Zhang N, Feng L, Ma C, Wang Y, Yang Z, Zhao J (2022) Melatonin alleviates aluminum-induced growth inhibition by modulating carbon and nitrogen metabolism, and reestablishing redox homeostasis in Zea mays L. Journal of Hazardous Materials 423, 127159.
| Crossref | Google Scholar |
Rizwan M, Usman K, Alsafran M, Jabri HA, Samreen T, Saleem MH, Tu S (2022) Nickel toxicity interferes with NO3−/NH4+ uptake and nitrogen metabolic enzyme activity in rice (Oryza sativa L.). Plants 11(11), 1401.
| Crossref | Google Scholar |
Rocha J, Carvalho-Santos C, Diogo P, Beça P, Keizer JJ, Nunes JP (2020) Impacts of climate change on reservoir water availability, quality and irrigation needs in a water scarce Mediterranean region (Southern Portugal). Science of The Total Environment 736, 139477.
| Crossref | Google Scholar |
Rodríguez A, Peña-Fleitas MT, Gallardo M, de Souza R, Padilla FM, Thompson RB (2020) Sweet pepper and nitrogen supply in greenhouse production: critical nitrogen curve, agronomic responses and risk of nitrogen loss. European Journal of Agronomy 117, 126046.
| Crossref | Google Scholar |
Rosen H (1957) A modified ninhydrin colorimetric analysis for amino acids. Archives of Biochemistry and Biophysics 67(1), 10-15.
| Crossref | Google Scholar |
Schäfer N, Maierhofer T, Herrmann J, Jørgensen ME, Lind C, von Meyer K, Lautner S, Fromm J, Felder M, Hetherington AM, Ache P, Geiger D, Hedrich R (2018) A tandem amino acid residue motif in guard cell SLAC1 anion channel of grasses allows for the control of stomatal aperture by nitrate. Current Biology 28(9), 1370-1379.e5.
| Crossref | Google Scholar |
Silalert P, Pattanagul W (2021) Foliar application of melatonin alleviates the effects of drought stress in rice (Oryza sativa L.) seedlings. Notulae Botanicae Horti Agrobotanici Cluj-Napoca 49(3), 12417.
| Crossref | Google Scholar |
Smith PK, Krohn RI, Hermanson GT, Mallia AK, Gartner FH, Provenzano MD, Fujimoto EK, Goeke NM, Olson BJ, Klenk DC (1985) Measurement of protein using bicinchoninic acid. Analytical Biochemistry 150(1), 76-85.
| Crossref | Google Scholar |
Sohag AAM, Tahjib-Ul-Arif M, Brestic M, Afrin S, Sakil MA, Hossain MT, Hossain MA, Hossain MA (2020) Exogenous salicylic acid and hydrogen peroxide attenuate drought stress in rice. Plant, Soil and Environment 66(1), 7-13.
| Crossref | Google Scholar |
Strain HH, Svec WA (1966) Extraction, separation, estimation, and isolation of the chlorophylls. In ‘The chlorophylls’. (Eds LP Vernon, GR Seely) pp. 21–66. (Academic Press: New York, USA). doi:10.1016/B978-1-4832-3289-8.50008-4
Sun Y, Li Y, Wang M, Wang C, Ling N, Mur LAJ, Shen Q, Guo S (2018) Redox imbalance contributed differently to membrane damage of cucumber leaves under water stress and Fusarium infection. Plant Science 274, 171-180.
| Crossref | Google Scholar |
Talaat NB, Todorova D (2022) Antioxidant machinery and glyoxalase system regulation confers salt stress tolerance to wheat (Triticum aestivum L.) plants treated with melatonin and salicylic acid. Journal of Soil Science and Plant Nutrition 22, 3527-3540.
| Crossref | Google Scholar |
Velikova V, Yordanov I, Edreva A (2000) Oxidative stress and some antioxidant systems in acid rain-treated bean plants: protective role of exogenous polyamines. Plant Science 151, 59-66.
| Crossref | Google Scholar |
Virnodkar SS, Pachghare VK, Patil VC, Jha SK (2020) Remote sensing and machine learning for crop water stress determination in various crops: a critical review. Precision Agriculture 21(5), 1121-1155.
| Crossref | Google Scholar |
Wang Y, Zhang X, Chen J, Chen A, Wang L, Guo X, Niu Y, Liu S, Mi G, Gao Q (2019) Reducing basal nitrogen rate to improve maize seedling growth, water and nitrogen use efficiencies under drought stress by optimizing root morphology and distribution. Agricultural Water Management 212, 328-337.
| Crossref | Google Scholar |
Wang F, Gao J, Yong JWH, Liu Y, Cao D, He X (2020) Glutamate over-accumulation may serve as an endogenous indicator of tricarboxylic acid (TCA) cycle suppression under NH4+ nutrition in wheat (Triticum aestivum L.) seedlings. Environmental and Experimental Botany 177, 104130.
| Crossref | Google Scholar |
Weisany W, Sohrabi Y, Heidari G, Siosemardeh A, Ghassemi-Golezani K (2012) Changes in antioxidant enzymes activity and plant performance by salinity stress and zinc application in soybean (Glycine max L.). Plant Omics Journal 5, 60-67.
| Google Scholar |
Wen B, Li C, Fu X, Li D, Li L, Chen X, Wu H, Cui X, Zhang X, Shen H, Zhang W, Xiao W, Gao D (2019) Effects of nitrate deficiency on nitrate assimilation and chlorophyll synthesis of detached apple leaves. Plant Physiology and Biochemistry 142, 363-371.
| Crossref | Google Scholar |
Wild R, Ooi L, Srikanth V, Münch G (2012) A quick, convenient and economical method for the reliable determination of methylglyoxal in millimolar concentrations: the N-acetyl-L-cysteine assay. Analytical and Bioanalytical Chemistry 403, 2577-2581.
| Crossref | Google Scholar |
Xia H, Xu T, Zhang J, Shen K, Li Z, Liu J (2020) Drought-induced responses of nitrogen metabolism in Ipomoea batatas. Plants 9(10), 1341.
| Crossref | Google Scholar |
Xia Q, Fu LJ, Tang H, Song L, Tan JL, Guo Y (2022) Sensing and classification of rice (Oryza sativa L.) drought stress levels based on chlorophyll fluorescence. Photosynthetica 60(1), 102-109.
| Crossref | Google Scholar |
Yamasaki S, Dillenburg LC (1999) Measurements of leaf relative water content in Araucaria angustifolia. Revista Brasileira de Fisiologia Vegeta 11, 69-75.
| Google Scholar |
Yan F, Zhang J, Li W, Ding Y, Zhong Q, Xu X, Wei H, Li G (2021) Exogenous melatonin alleviates salt stress by improving leaf photosynthesis in rice seedlings. Plant Physiology and Biochemistry 163, 367-375.
| Crossref | Google Scholar |
Yoneyama T, Suzuki A (2019) Exploration of nitrate-to-glutamate assimilation in non-photosynthetic roots of higher plants by studies of 15N-tracing, enzymes involved, reductant supply, and nitrate signaling: a review and synthesis. Plant Physiology and Biochemistry 245-254.
| Crossref | Google Scholar |
Zahoor R, Zhao W, Abid M, Dong H, Zhou Z (2017) Potassium application regulates nitrogen metabolism and osmotic adjustment in cotton (Gossypium hirsutum L.) functional leaf under drought stress. Journal of Plant Physiology 215, 30-38.
| Crossref | Google Scholar |
Zhang N-N, Zou H, Lin X-Y, Pan Q, Zhang W-Q, Zhang J-H, Wei G-H, Shangguan Z-P, Chen J (2020) Hydrogen sulfide and rhizobia synergistically regulate nitrogen (N) assimilation and remobilization during N deficiency-induced senescence in soybean. Plant, Cell & Environment 43(5), 1130-1147.
| Crossref | Google Scholar |
Zou J, Yu H, Yu Q, Jin X, Cao L, Wang M, Zhang Y (2021) Physiological and UPLC-MS/MS widely targeted metabolites mechanisms of alleviation of drought stress-induced soybean growth inhibition by melatonin. Industrial Crops and Products 163, 113323.
| Crossref | Google Scholar |