Shaking off the blow: plant adjustments during submergence and post-stress growth in Lotus forage species
Florencia B. Buraschi




A
B
C
D
Handling Editor: Ole Pedersen
Abstract
Flooding significantly hampers global forage production. In flood-prone regions, Lotus tenuis and Lotus corniculatus are common forage legumes, yet little is known about their responses to partial or complete submergence. To address this, we evaluated 10 Lotus accessions subjected to 11 days of either partial or complete submergence, analysing growth traits related to tolerance and recovery after de-submergence. Principal component analyses revealed that submergence associated growth parameters were linked to L. corniculatus accessions, whereas recovery was associated with L. tenuis accessions. Notably, in L. tenuis, recovery from complete submergence positively correlated with leaf mass fraction but negatively with root mass fraction, showing an opposite pattern than in L. corniculatus. Encouragingly, no trade-off was found between inherent growth capacity and submergence tolerance (both partial and complete) or recovery ability, suggesting genetic selection for increased tolerance would not compromise growth potential. L. tenuis exhibited accessions with both partial and complete submergence tolerance, making them versatile for flood-prone environments, whereas L. corniculatus accessions were better suited for partial submergence. These findings offer valuable insights to enhance forage production in flood-prone areas and guide the selection of appropriate Lotus accessions for specific flood conditions.
Keywords: complete submergence, correlations, Lotus corniculatus, Lotus tenuis, partial submergence, PCA, recovery, submergence tolerance.
Introduction
Flooding is a significant constraint on crop and forage production worldwide, causing detrimental effects on terrestrial plants (Bailey-Serres and Voesenek 2008; Colmer and Voesenek 2009; Voesenek and Bailey-Serres 2013). The Intergovernmental Panel on Climate Change (http://www.ipcc.ch/) predicts that global warming will lead to more erratic weather patterns and increased precipitation frequency (Kundzewicz et al. 2019). Consequently, the occurrence of water excess and varying degrees of plant submergence in flooded plains and cultivated lands is expected to rise in the near future. Complete submergence of the shoot is one of the most challenging situations for plants in flood-prone environments (Voesenek et al. 2006). Rapid oxygen depletion and the shift from aerobic to fermentative metabolism (Colmer and Voesenek 2009) can disrupt plant energy balance, resulting in biomass losses and eventual death of submerged individuals.
Legume species, in conjunction with grasses, are essential components of pastures (Striker and Colmer 2017). Pastures that include legumes exhibit higher digestibility and crude protein content, as well as lower neutral detergent fibre levels compared to pastures consisting solely of grasses (Graham and Vance 2003). Additionally, these legume-based pastures demonstrate a more consistent yield distribution throughout the season. The genus of Lotus plants comprises over 180 legume species distributed worldwide (Pajuelo and Stougaard 2005). Lotus spp. are currently used to improve pastures in challenging environments that are susceptible to flooding, where other traditional legumes such as alfalfa or red clover do not thrive (Dear et al. 2003). Selective breeding has been carried out to domesticate and improve legumes like Lotus corniculatus and Lotus tenuis. These species showed varying degrees of tolerance to waterlogged soils (James and Crawford 1998; Striker et al. 2005; Teakle et al. 2006) and submergence (Striker et al. 2012; Di Bella et al. 2020), with L. tenuis displaying the highest tolerance to oxygen deficiency. Only one study has been conducted to investigate intraspecific responses to submergence within Lotus accessions (Di Bella et al. 2020). Di Bella et al. (2020) focused on the effects of complete submergence for 7 days on Lotus plants that were 35 days old and cultivated in a nutrient solution. The study identified differences in stem extension, petiole hyponastic growth and shoot-to-root ratio among various accessions (Di Bella et al. 2020). Nevertheless, the specific traits that contribute to submergence tolerance and the underlying mechanisms involved in plant recovery from this stress in Lotus forage species are still poorly understood. This paper aims to bridge these knowledge gaps.
Biomass allocation to different plant organs is influenced by species, ontogeny and the plant’s environment (Poorter and Nagel 2000). Stressful scenarios like partial and complete submergence can alter biomass allocation in plants (Poorter et al. 2012). The shoot-to-root ratio (S/R) is often used to express changes in allocation, indicating the distribution of biomass above and below the ground (Wilson 1988; see Buraschi et al. (2020) for Lotus japonicus accessions). However, there is a conceptual limitation with using S/R values. Describing allocation in terms of only two compartments, by combining stems and leaves, fails to acknowledge the distinct functions of these organs. To address this, we will consider three compartments – leaves, stems and roots – in our allocation analyses, following the suggestion by Poorter and Nagel (2000). This approach allows us to characterise allocation using fractions that represent the biomass of each organ relative to the total plant: leaf mass fraction (LMF), stem mass fraction (SMF) and root mass fraction (RMF). The use of biomass fractions offers another advantage: they are less sensitive to minor changes in allocation compared to S/R values, especially when roots contribute a smaller proportion of the total biomass. This aspect is particularly relevant to our study, as complete submergence can represent a severe impact on the root system of Lotus plants and can also accelerate leaf senescence upon re-oxygenation (Signorelli et al. 2013; Steffens et al. 2013; Nasrullah et al. 2022). Consequently, the resumption of growth after the stress, expressed as the post-submergence relative growth rate (RGR), can be significantly influenced by two factors: the fraction of leaves contributing to current carbon fixation and the fraction of roots that survive submergence, particularly if water uptake is the primary limiting factor. Thus, one of our objectives was to determine which organ fraction better explains the recovery process from submergence in the forage species being investigated.
A classical ecological theory suggests that there is a trade-off between growth under non-limiting conditions and tolerance to abiotic stress in plants (Chapin et al. 1993; Weih 2001; Assmann 2013). This usually implies resource allocation shifts in order to optimise plant growth under different environmental conditions. In simpler terms, this theory suggests that plants that grow quickly under non-stressful conditions are less capable of tolerating abiotic stress, like submergence. Conversely, plants that exhibit high growth under stressful conditions show lower growth rates when they are in non-stressful growing conditions. As an alternative possibility, it is conceivable that we may not discover any correlation between inherent growth traits and submergence tolerance, as breeding efforts could have focused not only on stress-tolerant accessions but also on those with higher productivity. Additionally, it has been observed that investing resources to sustain growth during submergence can compromise subsequent growth in drained conditions, as demonstrated in a specific accession of L. japonicus (MG20) where accelerated leaf senescence upon re-oxygenation was associated with poor recovery after complete submergence (Striker et al. 2012). To investigate these hypotheses further, we examined the response correlations of various accessions of L. corniculatus and L. tenuis when exposed to both non-stressful (control) conditions and submergence. Our study also aimed to explore the potential link between growth responses during submergence stress and the subsequent recovery from stress, with a special focus on investigating the hypothesis of a trade-off in growth between different periods.
The selection of appropriate species is crucial for achieving successful pasture performance in environments characterised by a predominant stress condition (Nichols et al. 2010, 2012). Furthermore, the selection of accessions within the same species that exhibit variability in their response to specific stresses of varying intensity, such as partial versus complete submergence, is also critical for fine-tuning genotypes to soil conditions. This helps to determine a better adaptation of these materials to the specific environment in which they will be grown. Examples of interesting variations in response to hypoxia, salinity and their combination were found for seven accessions of the forage grass Festuca arundinacea (Menon-Martínez et al. 2021). These findings led to specific recommendations for each stress combination/environment, highlighting the suitability of the accessions. Thus, from a practical point of view, exploring the tolerance of different accessions of the legumes of this study, which are commonly used for forage purposes, across different submergence scenarios (partial vs complete submergence) can assist in selecting the most appropriate materials for optimal productivity under prevailing pasture conditions.
So, we propose the following research questions in relation to the preceding antecedents:
What morphological and growth traits can account for the variability in the response of L. tenuis and L. corniculatus accessions in each submergence treatment? Are the accessions/species differentially grouped with the growth variables during the stress and the recovery period?
Have there been any changes observed in biomass allocation between these Lotus species because of submergence treatments? Is there a correlation between the recovery from submergence, as measured by the relative growth rate (RGR), and the proportions of leaves, stems and roots, or the shoot-to-root ratio in plants at the end of the stress period?
Is there a trade-off between the intrinsic growth rate under control conditions and the growth rate during or after submergence stress? Is there a trade-off between growth during the submergence stress period and growth after the stress period?
Do the accessions exhibit similar tolerance and responses to partial submergence and complete submergence? Which species/accessions are more suitable for environments prone to shallow floods (resulting in partial plant submergence) and which ones are better adapted for deeper floods (leading to complete plant submergence)?
Materials and methods
Plant material
L. tenuis Waldst. and Kit. ex Willd., commonly known as narrowleaf birdsfoot trefoil, is a perennial legume with an extended growing season. Typically, it has a prostrate growth habit and possesses a well-developed taproot with extensive shallow lateral root branching. The stems bear pentafoliate leaves, with two of the leaflets at the base of the petiole resembling stipules. This plant plays a significant role as a forage crop in flood-prone soils and has naturalised in various plant communities within the Flooding Pampa grasslands of Argentina (Striker et al. 2005, 2008). It is cultivated in areas where the soil conditions can be severely affected by alkalinity, salinity or periodic flooding (Dear et al. 2003; Striker et al. 2011; Escaray et al. 2012).
L. corniculatus L., commonly known as birdsfoot trefoil, is a short to medium-lived perennial legume. It is sparsely pubescent and can exhibit a range of growth habits from prostrate to erect. It has a taproot and abundant lateral roots, primarily within the top 60 cm of soil, with the potential to extend as deep as 120 cm. The stems emerge from the roots or from basal nodes on the remaining stubble after grazing or cutting. Among the domesticated Lotus species, L. corniculatus holds the greatest importance for fodder production. It is well-adapted to tolerate certain levels of salinity, acidity, low fertility and poorly drained soils (Dear et al. 2003; Striker et al. 2005; Escaray et al. 2012).
Both forage legumes, L. tenuis and L. corniculatus, were selected for this experiment. These species are commonly used as the legume component in pastures and are also present in natural grasslands, providing a source of protein in the cattle diet (Escaray et al. 2012; Antonelli et al. 2019). Specifically, five accessions of L. tenuis (A: Aguape, L: Larrañaga, C: Chaja, P: Pampa INTA, E: Esmeralda) and five accessions of L. corniculatus (N: Nilo, IN: Inia Draco, SG: San Gabriel, K: Kontact, T: Toro) were chosen due to their widespread use in sowing, interseeding and research (Manzur et al. 2009; Striker et al. 2012; Marinoni et al. 2017, among others).
Experimental details
Germination of the 10 Lotus accessions was initiated by placing seeds in Petri dishes containing cotton and filter paper soaked in distilled water. Petri dishes were then placed in a growth chamber in darkness, with a temperature regime diurnally alternating between 20°C and 30°C (Manzur et al. 2009). Two seedlings from each accession and species were transplanted into pots (0.6 L) filled with a substrate composed of 50% sand and 50% soil from the top horizon of a typical Natraquoll soil and transferred to a greenhouse at the Faculty of Agronomy, University of Buenos Aires, Argentina. Seedlings were allowed to grow in the greenhouse until they reached the stage of developing five true leaves prior to the application of treatments.
Three treatments were applied to 30-day-old seedlings for a duration of 11 days (stress period), following a fully randomised design with 10 replicates: (1) control (C) – daily watering to maintain field capacity; (2) partial submergence (PS) of the seedlings, where the water level reached approximately half the height of the seedlings (approximately 3–4 cm above the soil); and (3) complete submergence (CS) of the seedlings, with the water level kept 5 cm above the height of the seedlings. Plastic containers (0.8 m × 0.5 m × 0.4 m depth) were used to facilitate the application of submergence treatments and maintain consistent water levels throughout the experimental period.
After the 11-day treatment period, pots were removed from the containers, and the performance of the plants was assessed for an additional 14 days (recovery period). Throughout this period, pots were watered to field capacity, allowing the evaluation of their recovery capacity following exposure to the different submergence treatments. To avoid any potential variations in sunlight or temperature gradients within the greenhouse that could affect the results, all pots were rotated on a weekly basis during the experiment. The average daily temperature in the greenhouse during the experiment ranged between 18°C and 25°C.
Measured variables
Measurements were taken at the beginning (Day 0), after the stress period (Day 11) and following the recovery period (Day 25). For each measurement period, morphological variables were quantified, including (1) stem number, (2) plant height and total plant biomass divided into (3) leaf biomass, (4) stem biomass and (5) root biomass. Harvested seedlings were dried in an oven at 80°C for 72 h and weighed on a precision balance. By separating them into different compartments, the shoot-to-root ratio (S/R) and leaf-to-stem ratio (L/Stem) were calculated for each treatment and harvest period. The shoot compartment included both leaves and stems for the S/R calculation.
The relative growth rate (RGR) of the plants was determined for each accession under the various treatments and experimental periods (stress and recovery) using the equation developed by Hunt (1982):
In the equation, W2 and W1 are the dry weights of plant biomass for the corresponding treatment at Times 2 and 1, respectively, with the value of W1 being the average biomass of the treatment (C, PS and CS) and t2 – t1 is the number of days between sampling times (i.e. 11 days for the stress period and 14 days for the recovery period). We calculated the RGR for the entire plants, as well as the RGR of different plant compartments, including leaves, stems and roots, allowing the comparison of potential differential effects of the treatments on growth and recovery from submergence among plant organs.
Statistical analyses
We conducted different analyses to provide evidence to address each one of the four research questions we had planned. To describe and summarise the underlying trends in the data related to submergence and recovery responses (Question 1), we employed a multivariate ordination method known as principal component analysis (PCA). The variables utilised in this analysis encompassed the relative growth rate (RGR) of various biomass response variables, including the overall plant biomass and the biomass of individual components such as leaves, stems, and roots. Additionally, we considered ratios such as shoot-to-root ratio and leaf-to-stem ratio. Furthermore, morphological variables such as the number of stems (and its change over time) and stem height were taken into account. These variables were assessed for both the stress period and the subsequent recovery period, considering both partial and complete submergence scenarios.
To address the second question regarding potential changes in biomass allocation among Lotus species caused by submergence treatments (C, PS and CS), we utilised boxplots that included all plants from each accession per species. This approach allowed us to observe the variability and distribution of the data, as well as conduct means comparisons using ANOVA followed by Tukey’s post hoc tests (P < 0.05). This analysis was carried out for both the stress period and the subsequent recovery period, enabling us to assess the extent of adjustment made by the plants during the post-stress period. Given the observed changes in biomass allocation resulting from the submergence treatments, we further conducted regression analyses to explore relationships between the relative growth rate (RGR) of total biomass during the recovery period, including leaf mass fraction (LMF), stem mass fraction (SMF), root mass fraction (RMF) and shoot-to-root ratio (S/R), all measured at the de-submergence stage (i.e. recovery period). The biomass fractions were computed based on the percentage of leaves, stems and roots relative to the total biomass for each replicate of every accession (see Poorter and Nagel 2000). These percentages were subsequently averaged for each treatment and time frame, both during stress and recovery periods. These additional analyses aimed to provide insight into the associations between biomass allocation patterns and the overall recovery performance of the plants.
The third question was addressed by using regression analysis to examine the potential trade-off between the inherent growth capacity of the accessions (measured as the RGR of controls) and the RGR under submergence treatments, as well as the ability to recover from the stresses.
The fourth question was investigated by plotting the relative growth rate (RGR) responses to complete submergence against the RGR under partial submergence. This approach enabled us to simultaneously observe the accessions’ responses to both stresses in relation to the average response. The quadrants formed by lines representing the average responses facilitated the identification of accessions that displayed RGR responses either above or below the species average under these conditions. It is noteworthy that comparing both the stress and recovery periods allowed for the assessment of the consistency in the accessions’ responses. This approach provided a clear and straightforward means to assess whether the accessions demonstrated comparable tolerance and responses to both partial submergence and complete submergence. Moreover, it facilitated the identification of accessions that were well-suited for specific submergence scenarios, based on their performance and adaptability.
In all cases, statistical tests and graphical analyses were performed using GraphPad Prism ver. 9.0.0 for Windows (GraphPad Software, San Diego, California USA).
Results
Groups of morphological and growth traits in the response of L. tenuis and L. corniculatus under submergence treatments
During the stress period, PCA analysis for partial submergence (Fig. 1a) revealed that the first two axes explained 71% of the total variance among the accessions based on the measured traits (see also Supplementary Fig. S1 for responses under control conditions). Principal Component 1 (PC1) accounted for 51% of the variation and separated accessions with high relative growth rates (RGR) for total biomass (TB), stems, roots, leaves and stem number (N stems), predominantly grouping L. corniculatus accessions. On the other hand, Principal Component 2 (PC2) contributed to 20% of the variance and effectively distinguished accessions with high shoot-to-root ratios (S/R) and plant height (H, see also Fig. S2 for more details on this variable), which were inversely related to leaf-to-stem ratios (L/Stem), predominantly grouping L. tenuis. Under complete submergence (Fig. 1c), the two axes explained 70% of the total variance. The first axis (PC1) accounted for 46% of this variance and differentiated accessions with high RGR for total biomass (TB), leaves, stems and stem number (N stem). PC2 explained 24% of the variance and displayed associations between H and S/R, which were inversely related to L/Stem. The distribution of accessions along the first PCA axis revealed greater variation for L. corniculatus under partial submergence and for L. tenuis under complete submergence (Fig. 1a, c).
Principal component analysis (PCA) plots depicting variables and accessions of Lotus tenuis and L. corniculatus under treatments involving partial submergence (a, b) and complete submergence (c, d) during the stress period (a, c) and the recovery period (b, d). The plots were generated using nine variables and 10 accessions. The variables analysed include the relative growth rate (RGR) of total biomass (TB), leaves (Leaves), stems (Stems) and roots (Roots), as well as the variation in the number of stems during the period (Δ N stems), the number of stems per plant (N stems), the shoot-to-root ratio (S/R), the leaf-to-stem ratio (L/Stem) and plant height (H). L. tenuis accessions are indicated by violet triangles: Aguape (A), Larrañaga (L), Chaja (C), Pampa INTA (P) and Esmeralda (E). Lotus corniculatus accessions are represented by green circles: San Gabriel (SG), Nilo (N), Inia Draco (IN), Kontac (K) and Toro (T). The percentage of explained variability is given in each axis.
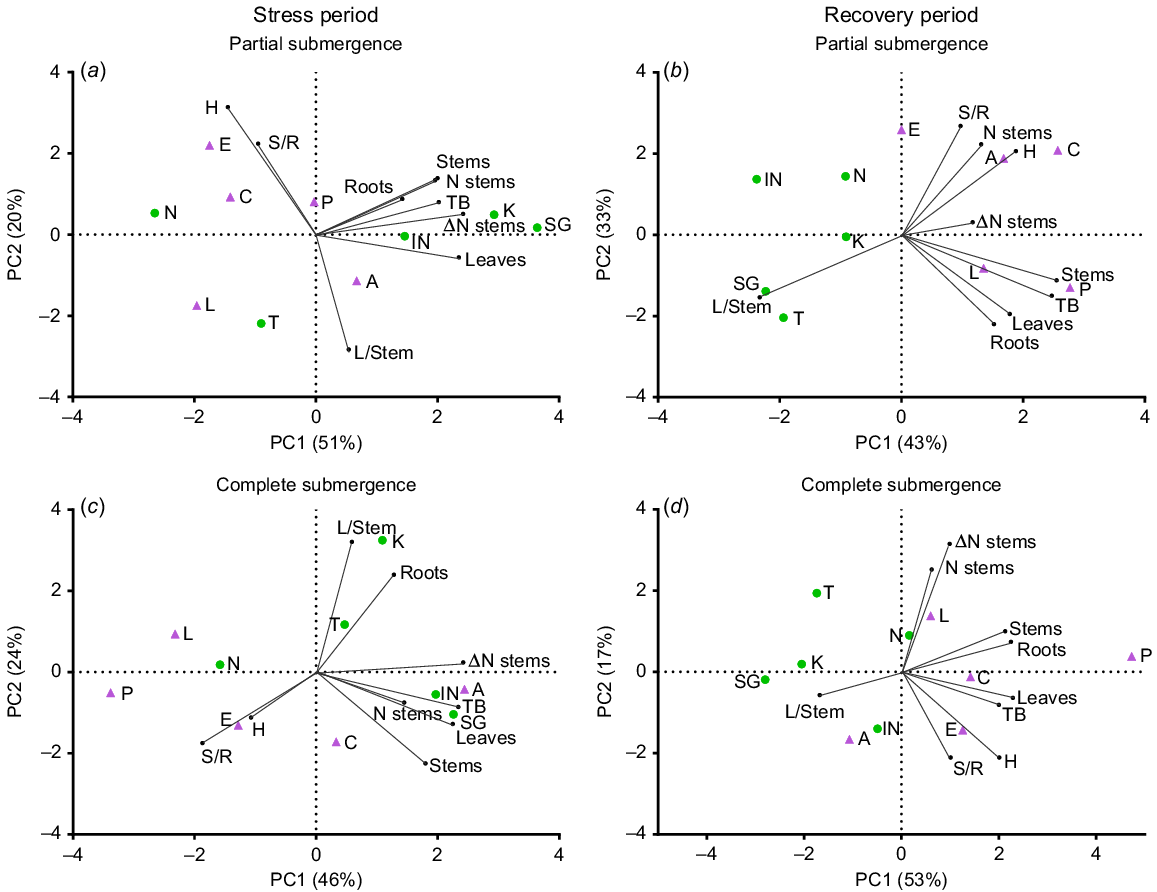
During the recovery period, PCA analysis revealed that the first two axes accounted for 76% and 70% of the total variance for the previously partially and completely submerged plants, respectively (Fig. 1b, d). The plots clearly demonstrate that a significant number of L. corniculatus accessions were positioned on the negative side of the PC1 axis, whereas most L. tenuis accessions were located on the positive side of the same axis, coinciding with the traits responsible for plant growth (RGRs). This distinct contrast between the two species was evident during the post-stress period and remained consistent for both submergence treatments. After partial submergence, the RGR of leaves, stems and roots is associated with explaining the total RGR (TB). Conversely, the change in stem number (ΔN stems) and the stem number are less associated with explaining growth than during the stress. Plant height and the S/R are inversely related to L/stem (Fig. 1b). After complete submergence, the RGR of roots is associated with the RGR of stems (and less to leaves; Fig. 1d). The stem number and its change over time are not clearly associated with biomass accumulation (i.e. TB). Additionally, plant height and the S/R are positively associated, as observed during the recovery from partial submergence, but they were not related to L/Stem ratios (Fig. 1d). Similar relationships applied in the control during the same recovery period (Fig. S1).
Changes in biomass allocation due to submergence treatments
There were variations in biomass allocation among different plant parts depending on the submergence treatment, as well as differences in allocation during the recovery period (Fig. 2). In the case of L. tenuis, leaf mass fraction (LMF) was reduced by 13% due to partial submergence, whereas complete submergence did not significantly affect LMF (Fig. 2a). After the recovery period, LMF was 18% higher under complete submergence compared to the other treatments, whereas partially submerged plants maintained the lowest LMF (34.6%) even 2 weeks after the stress period (Fig. 2a). Stem mass fraction (SMF) was 17.8% lower under complete submergence compared to control and partial submergence during the stress period (Fig. 2c). However, it recovered during the post-stress period, with all treatments showing similar values for SMF (33–35.5%). Root mass fraction (RMF) did not change significantly due to submergence during the stress period, but it was 18.5% lower at the end of the recovery period for plants previously subjected to complete submergence (Fig. 2e).
Leaf mass fraction (a, b), stem mass fraction (c, d) and root mass fraction (e, f) during the stress and recovery periods under control (C), partial submergence (PS) and complete submergence (CS) conditions in Lotus tenuis in violet colour (left panels) and L. corniculatus in green colour (right panels), respectively. The measurements are presented using box plots, where each box represents 50% of the data. The median is indicated by a horizontal line within each box, the mean is denoted by ‘+’, and the bars extending from each box represent the 10th and 90th percentiles. Error bars depict values below the 25th percentile and those exceeding the 75th percentile of the data. Data from the accessions (10 replicates for each of the five accessions, n = 50) were aggregated for each treatment within the species. Different letters indicate significant differences among treatments based on the Tukey test (P < 0.05) for both the stress period (lowercase letters) and the recovery period (uppercase letters) within species.
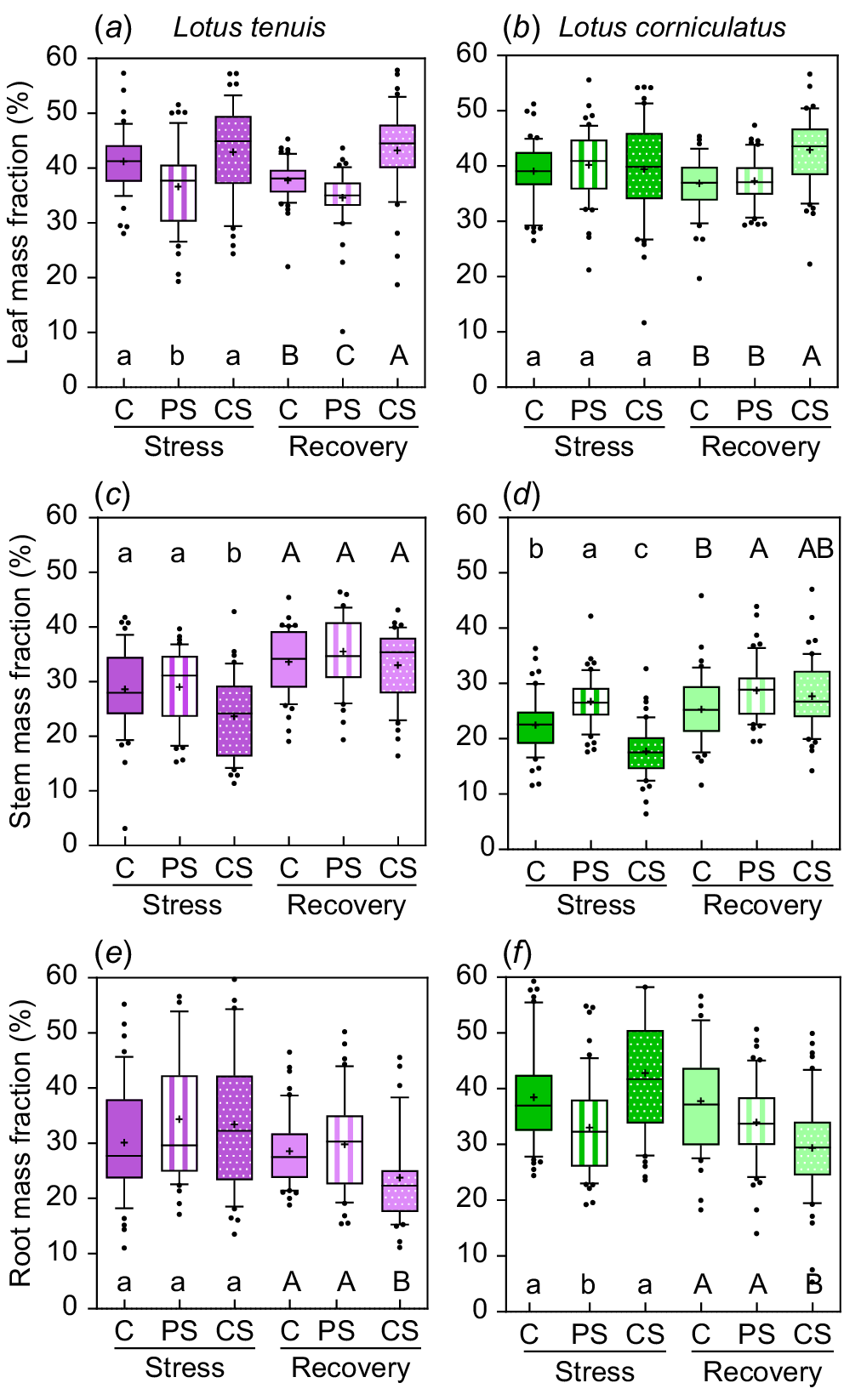
In L. corniculatus, LMF was unaffected by submergence treatments during the stress period. However, it is worth noting that previously completely submerged plants exhibited a 15.7% higher LMF at the end of the recovery period (Fig. 2b). SMF during the stress period was 18.8% higher under partial submergence, whereas it was 21.1% lower under complete submergence compared to the control group. Following the recovery period, SMF remained slightly higher (8.5%) under partial submergence (Fig. 2d). RMF was 18.6% lower under partial submergence during the stress period, with no significant differences observed between control and complete submergence groups. However, at the end of the recovery period, RMF was 18.1% lower only for plants previously subjected to complete submergence (Fig. 2f).
Correlation between recovery from submergence and biomass allocation
There were interesting correlations observed between RGRs of the recovery period and the analysed biomass fractions (i.e. LMF, SMF, RMF), or shoot-to-root ratio (S/R) of the stress period, specifically in the case of complete submergence conditions (CS) (Fig. 3). Regarding the resumption of plant growth, there was a divergent correlation with LMF upon de-submergence (i.e. recovery period) between the two Lotus species. In L. tenuis, an increase in the proportion of leaves at the beginning of the recovery period was positively associated with higher RGR (Fig. 3a). In contrast to findings in L. tenuis, there was a negative correlation between these variables in L. corniculatus, suggesting that higher RGR were associated with lower LMF (Fig. 3b). No correlation was found between RGR and SMF upon de-submergence in either species (Fig. 3c, d). However, a negative correlation was observed between RGR and RMF, only in L. tenuis (Fig. 3e, f). As expected, in L. tenuis, the positive and negative correlations between RGR and LMF, as well as RMF, were reflected in a positive correlation with S/R upon de-submergence (Fig. 3g), which was not observed in L. corniculatus (Fig. 3h). Finally, no relationship was observed between the RGR for the recovery from partial submergence (PS) and any of the analysed potential growth predictors (LMF, SMF, RMF or S/R) (Fig. S3).
Relative growth rate (RGR) of entire plants of Lotus tenuis (left panels) and L. corniculatus (right panels) accessions, during the recovery period after 11 days of complete submergence, are shown as a function of the leaf mass fraction (a, b), stem mass fraction (c, d), root mass fraction (e, f) and shoot/root ratio (g, h) at de-submergence (i.e. at the end of the stress period). Accession codes for L. tenuis accessions are indicated by violet triangles: Aguape (A), Larrañaga (L), Chaja (C), Pampa INTA (P) and Esmeralda (E). The codes for L. corniculatus are indicated by green circles: San Gabriel (SG), Nilo (N), Inia Draco (IN), Kontac (K) and Toro (T). The adjusted parameters of the regressions are presented within each graph. Error bars indicate the standard error of the mean (s.e.m.).
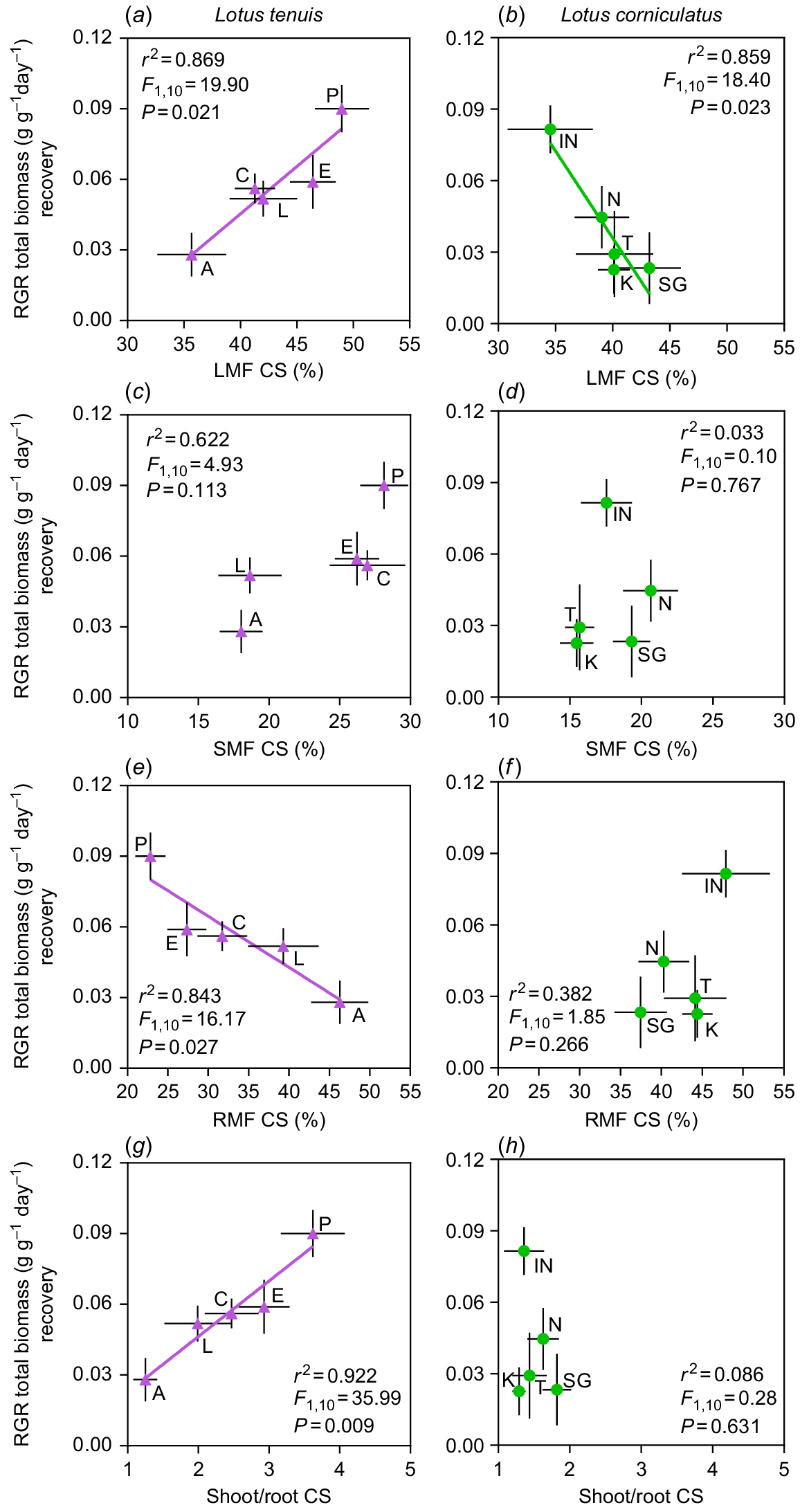
Assessing trade-offs in intrinsic growth rate at control and submergence conditions
For L. tenuis, the relationship between the RGR of accessions under non-stressful conditions (C) and RGR under partial submergence (PS) was positive (Fig. S4a). A similar trend was observed, but it was not statistically significant, under complete submergence (CS) during the stress period (Fig. S4c). However, during the recovery period, there was no association between the inherent growth (i.e. RGR under control conditions) and the growth of plants recovering from either submergence treatment (Fig. S4e, g).
In the case of L. corniculatus, no correlation was found between the inherent growth of its accessions (i.e. RGR of controls) and the RGR of the plants during or after submergence. This lack of relationship was consistent for both partial and complete submergence treatments, as well as during both the stress and recovery periods (Fig. S4b, d, f and h).
Variation among Lotus species/accessions in response to partial and complete submergence
In the case of L. tenuis, there were divergent responses among accessions between the stress and recovery periods for complete submergence (Fig. 4a, b). Accessions Aguape (A) and Chaja (C), which initially displayed above-average performance under complete submergence (CS) (Fig. 4a), showed poor performance during the recovery period (Fig. 4b). On the other hand, accession Pampa INTA (P), despite having the lowest RGR during the stress period, emerged as the most promising accession in terms of coping with complete submergence by the end of the experiment. In this species, accessions’ responses were more consistent for partial submergence (PS), with the same accessions exhibiting high RGR during the stress period also performing well after the recovery period. Notably, during recovery, none of the L. tenuis accessions displayed a clear negative behaviour in terms of RGR under either partial submergence or complete submergence (Fig. 4b, void in quadrant −−), as observed in the prior period.
Relative growth rate (RGR) of entire plants from Lotus tenuis and L. corniculatus accessions during (a, c) or recovering from (b, d) complete submergence (CS), plotted against the RGR of the same accessions during (or recovering from) partial submergence (PS). The L. tenuis accessions are denoted by A (Aguape), L (Larrañaga), C (Chaja), P (Pampa INTA) and E (Esmeralda) indicated by violet triangles. The L. corniculatus accessions are represented by the codes SG (San Gabriel), N (Nilo), IN (Inia Draco), K (Kontac) and T (Toro) indicated by green circles. Vertical dashed lines indicate the average RGR of plants under PS and horizontal dashes represent the average RGR of plants under CS, whether during the stress or during recovery. The quadrants formed by these lines allow for the observation of accessions that display RGR responses either above or below the species average under these conditions. Error bars indicate the standard error of the mean (s.e.m.).
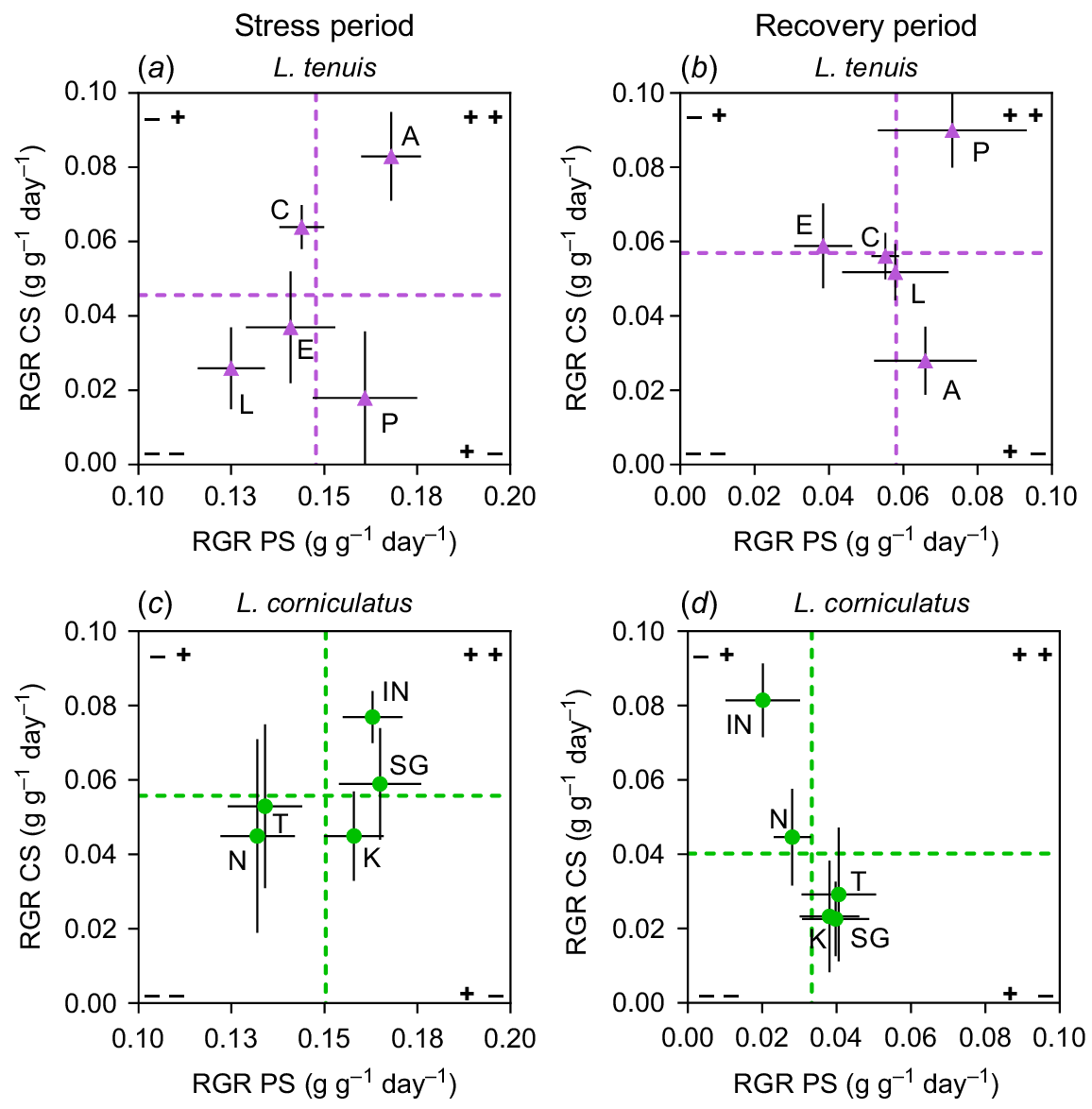
In the case of L. corniculatus, there were more contrasting responses among accessions to complete submergence (CS) compared to partial submergence (PS) in terms of range of variability in RGR (Fig. 4c, d). Interestingly, none of the accessions demonstrated ability to perform well under either submergence scenario when assessed after the recovery period (Fig. 4d, void in quadrant ++). Regarding partial submergence, variation among accessions observed during the stress period (indicated by the dispersion of points on the x-axis) was nearly eliminated after the recovery period. Accessions exhibited very similar RGR responses, with one exception: Inia Draco (IN) consistently displayed above-average RGR for complete submergence in both stress and recovery periods. However, it showed below-average RGR when subjected to partial submergence. A similar case was observed with San Gabriel (SG), but in the case of partial submergence. It showed good performance under this stress condition, but not under complete submergence after considering the recovery period. The results indicate a trade-off among the accessions concerning their ability to recover from complete submergence (Fig. 4d, quadrant −+) compared to their recovery from partial submergence (Fig. 4d, quadrant +−).
Discussion
This study aimed to identify primary variables that impact tolerance of L. tenuis and L. corniculatus accessions, which are forage legumes, to varying water depths resulting in partial and complete submergence of the plants. It also focused on identifying major growth/allocation traits that assist in the recovery of these plants from such stresses. To guide this research, four questions were formulated and their answers led to the following main findings. First, the principal component analyses showed that growth parameters during submergence were associated with L. corniculatus accessions, whereas during recovery they were mostly associated with L. tenuis accessions (Fig. 1). Second, in L. tenuis, recovery from complete submergence was positively correlated with leaf mass fraction and negatively correlated with root mass fraction at the beginning of the post-stress period, whereas the opposite pattern was observed in L. corniculatus (Figs 2 and 3). Third, there was no trade-off between the inherent growing capacity of the accessions and their tolerance to submergence or recovery ability (Fig. S4). Lastly, the performance of the accessions under partial submergence differed from their performance under complete submergence (Fig. 4). Notably, L. tenuis exhibited more promising materials capable of tolerating both submergence scenarios compared to L. corniculatus. Each one of these main findings will be discussed in the following sections.
Contrasting performances of L. tenuis and L. corniculatus during recovery period
The assessment of plant performance in response to submergence should consider both the stress and the subsequent recovery period. It is during this latter period that the true tolerance to submergence can be determined (Striker 2012). This study reveals that the recovery period clearly differentiated the two species. All L. tenuis accessions (excluding Aguape) were grouped together with the variables that explain growth (such as plant RGRs and its compartments), whereas L. corniculatus accessions were positioned on the opposite quadrants. So, the behaviour in response to submergence differs between species, although some variability can be found among accessions within species. Comparing our results is challenging due to the limited availability of studies that have implemented submergence treatments across multiple accessions of these species. Only one such study, conducted by Di Bella et al. (2020), is available for comparison. Nevertheless, our findings demonstrate a considerable range of variability among our five accessions concerning the relative growth rate (RGR) during the recovery period, with values spanning from mild to high (i.e. 0.03–0.09 g g−1 day−1). This variability aligns with the observations made by Di Bella et al. (2020) for 10 accessions, despite differences in the specific accessions studied and the focus of their research being on the submergence period. Additionally, other studies provide valuable insights into the individual responses of specific accessions, allowing for meaningful comparisons. The high ability of L. tenuis accessions Pampa INTA (P), Chaja (C) and Larrañaga (L) to recover from partial submergence aligns with previously reported findings (Striker et al. 2005; Teakle et al. 2006; Antonelli et al. 2019), as well as the ability of Pampa INTA (P) to recover from complete submergence (Striker et al. 2012). On the other hand, the poor ability of L. corniculatus accessions to recover from both partial and complete submergence matches the findings of previous studies on the San Gabriel (SG) accession, where biomass accumulation was reduced by 41–67% compared to controls (Striker et al. 2005, 2012; Antonelli et al. 2019). Our findings clearly demonstrate that the perception of L. tenuis as highly tolerant and L. corniculatus as moderately tolerant to either partial or complete submergence is a result of their distinct recovery ability. However, on the positive side, L. corniculatus exhibits better forage quality, with improved protein assimilation, reduced enteric fermentation and decreased cattle swelling (Sivakumaran et al. 2006; Escaray et al. 2012).
Allocation changes during submergence impact plant recovery
The allocation of biomass among leaves, stems and roots displayed variations in response to partial submergence, revealing general patterns within the species (Fig. 2). Specifically, in the case of L. tenuis, the fraction of biomass allocated to leaves (LMF) decreased due to partial submergence, with a slight trend towards higher stem mass fraction (SMF) and no apparent changes were observed in the fraction allocated to roots (RMF). In L. corniculatus, SMF increased due to partial submergence, but at the expense of RMF. The increase in stem fraction can be considered a strategic resource allocation by plants, aiming to promote taller plant growth, which enhances likelihood of positioning a greater proportion of leaves above the water surface for effective carbon fixation. This notion is supported by studies conducted by Striker et al. (2005) on these Lotus spp. and Striker et al. (2019) on 15 accessions of Melilotus siculus. Additionally, Di Bella et al. (2020) observed a corresponding increase after partial submergence in the growth rate of primary stems in various accessions of L. tenuis and one accession of L. corniculatus, further supporting this hypothesis.
Complete submergence resulted in significant changes in biomass allocation in both Lotus species. Under complete submergence, the LMF was slightly elevated, with RMF slightly reduced compared to other treatments in both species. These patterns are likely tied to the plant’s prioritised investment in leaf biomass to support carbon fixation (Striker et al. 2014), thereby assisting in plant recovery at the expense of root proportion. This minor reduction in the root fraction does not appear to compromise the plants’ water balance (cf. Buraschi et al. 2020). Interestingly, we found variation in biomass fractions among different accessions, which was correlated with their regrowth ability following submergence stress (Fig. 3). Particularly for submergence-tolerant L. tenuis, accessions that displayed an increase in LMF, a decrease in RMF, or a high shoot-to-root ratio (S/R) exhibited the most robust growth after complete submergence. These findings indicate that assimilation by remaining leaves plays a crucial role in post-submergence recovery. This response aligns with observations in other submergence-tolerant species, such as Phalaris aquatica (Striker and Ploschuk 2018), Hemarthria altissima (Luo et al. 2009) and Chloris gayana (Striker et al. 2017). Hence, the current study highlights the significance of the remaining functional leaves in enhancing recovery ability of previously submerged plants. We also found that the positive correlation between shoot-to-root ratio (S/R) and post-stress growth in our study contrasts with findings we reported for L. japonicus accessions (Buraschi et al. 2020) using a similar approach. We speculate that the range of S/R values explored in both studies contributes to the seemingly contradictory results between both reports. In our current study, S/R ratio ranged from 1 to 4 for L. tenuis accessions (depending on the specific accession, as shown in Fig. 3g), whereas the previous study on L. japonicus had a wider S/R range of 2 to 10 (Buraschi et al. 2020). Upon further analysis of the previous report, it becomes apparent that, based on the range of S/R values investigated in our study, there was no distinct correlation between post-stress relative growth rate (RGR) and S/R values in L. japonicus (within the range of S/R: 2 to 5). Consequently, it seems that S/R values observed in L. tenuis following de-submergence did not result in an imbalanced transpiration-to-water uptake ratio that could impact the plants water status (Striker et al. 2012). This, in turn, facilitated rapid growth, especially when a significant proportion of leaves contributed to the plant’s carbon fixation process.
In the less submergence-tolerant L. corniculatus, we did not observe significant relationships for most fractions of plant biomass (SMF, RMF, S/R), except for a negative correlation between recovery growth and LMF. However, we believe that this correlation is unlikely to be attributed to the functionality of the leaves or the water relations of the plants. In this regard, the limited range of flexibility in modifying the shoot-to-root ratio upon complete submergence (S/R ranging from 1 to 2), compared to L. tenuis (S/R ranging from 1 to 4), supports this idea. It suggests that the ratio of transpiration to water uptake, approximated by S/R, is not significantly affected in L. corniculatus. Therefore, it is more plausible that the impaired functionality of the leaves in terms of carbon assimilation influences the plants’ performance during the recovery process. To gain deeper insights into the contrasting recovery capacities from complete submergence in L. tenuis and L. corniculatus, it is recommended to explore their respective abilities to cope with reactive oxygen species (ROS) that are produced after de-submergence during post-anoxic injury (Sasidharan et al. 2018). Conducting future studies that compare ROS production and the mitigation of oxidative damage between these species would offer valuable information in unravelling this aspect. Additionally, it is worth noting that differences among genotypes within these legume species have been observed in terms of anthocyanin and other flavonoid accumulation when exposed to various abiotic stresses (e.g. cold, salinity; Escaray et al. 2019). Considering the suggested role of these compounds as ROS scavengers in enhancing plant abiotic stress tolerance, investigating their involvement in submergence recovery could be of significance.
Lotus accessions tolerant to complete submergence differ from those for partial submergence
The tolerance to partial and complete submergence exhibited variation among different accessions, and responses of these accessions diverged when assessed at the end of the stress period or at the end of the recovery. This observation further supports the idea that tolerance should be determined by evaluating the plants after they have had an opportunity to recover from stress (Striker 2012; Sasidharan et al. 2017). To illustrate this point regarding L. tenuis, accessions with the highest (Aguape) and lowest RGR (Pampa INTA) during complete submergence exhibited contrasting behaviour during the recovery period. Interestingly, these same accessions displayed above-average RGR when partially submerged in both the stress and recovery periods. Moreover, among all the accessions examined, Pampa INTA (P) consistently exhibited above-average RGR for both stresses, making it an exceptionally promising candidate for further assessment in breeding programs targeting the development of materials with tolerance to varying water-depth scenarios involving submergence. This accession has demonstrated remarkable flexibility, employing either vigorous shoot elongation to avoid partial submergence, or a non-elongating quiescent strategy when fully submerged, relying on stored reserves (Manzur et al. 2009). The possession of these alternative growth strategies may help elucidate the accession’s potential success in environments characterised by unpredictable flooding depths.
In the case of L. corniculatus, no accessions demonstrated tolerance to both stresses when evaluated at the end of the recovery period. However, there were accessions that exhibited interesting tolerance to partial submergence, such as San Gabriel (SG) and Toro (T), and other accessions that displayed good recovery from complete submergence, such as Inia Draco (IN). This indicates that for these accessions, which are commonly used for pasture in field conditions (Rebuffo 1998; Artola et al. 2003; Antonelli et al. 2019), the selection process involves a trade-off in choosing materials specifically suited for either partial or complete submergence, but not both simultaneously. Our overall findings align well with the species’ reputation for having intermediate tolerance to water excess in comparison to L. tenuis (Striker et al. 2005, 2012; Teakle et al. 2006; Antonelli et al. 2019).
Finally, genetic variation plays a crucial role for selection of materials with enhanced abiotic tolerance of pasture crops, such as water excess and water deficit, through breeding programs (Chapman et al. 2012). In this study, we have presented evidence that challenges the expected trade-off between a plant’s intrinsic growth ability under non-stressful conditions (i.e. controls) and its growth response when confronted with submergence-induced stress (Chapin et al. 1993; Weih 2001). Surprisingly, our findings reveal no apparent correlation between these two traits. However, it is important to note that this lack of correlation should not be perceived as negative news. The genetic selection for increased tolerance to water excess may not necessarily compromise the growth potential of these genotypes in the absence of stress.
In conclusion, our principal component analyses highlighted a connection between submergence-related growth parameters and L. corniculatus accessions, whereas recovery traits were more prominent in L. tenuis accessions. Interestingly, within L. tenuis, a positive correlation was observed between recovery post-submergence and leaf mass fraction, whereas an inverse relationship was noted with root mass fraction. Contrarily, L. corniculatus demonstrated the opposite trend. A promising observation was the absence of any trade-off between inherent growth capability and tolerance to both partial and complete submergence or the ability to recover, indicating that selecting for greater tolerance would not adversely affect growth potential. Moreover, L. tenuis accessions showcased tolerance to both partial and complete submergence, positioning them as ideal candidates for flood-prone areas of uncertain water depth. In contrast, L. corniculatus accessions were more adept at handling only partial submergence. These findings offer valuable perspectives that could significantly enhance forage production in flood-prone areas and inform the selection of Lotus accessions best suited for specific flood conditions.
Data availability
The data that supports this study will be shared upon reasonable request to the corresponding authors.
Declaration of funding
This study was funded by grants from University of Buenos Aires (UBA 20020170100319BA) and ‘Agencia Nacional de Promoción Científica y Tecnológica’ (ANPCyT PICT-2021-00056).
References
Antonelli CJ, Calzadilla PI, Vilas JM, Campestre MP, Escaray FJ, Ruiz OA (2019) Physiological and anatomical traits associated with tolerance to long-term partial submergence stress in the Lotus genus: responses of forage species, a model and an interspecific hybrid. Journal of Agronomy and Crop Science 205, 65-76.
| Crossref | Google Scholar |
Artola A, de los Santos GG, Castañeda GC (2003) A seed vigour test for birdsfoot trefoil (Lotus corniculatus L.). Seed Science and Technology 31, 753-757.
| Crossref | Google Scholar |
Assmann SM (2013) Natural variation in abiotic stress and climate change responses in Arabidopsis: implications for twenty-first-century agriculture. International Journal of Plant Sciences 174, 3-26.
| Crossref | Google Scholar |
Bailey-Serres J, Voesenek LACJ (2008) Flooding stress: acclimations and genetic diversity. Annual Review of Plant Biology 59, 313-339.
| Crossref | Google Scholar | PubMed |
Buraschi FB, Mollard FPO, Grimoldi AA, Striker GG (2020) Eco-physiological traits related to recovery from complete submergence in the model legume Lotus japonicus. Plants 9, 538.
| Crossref | Google Scholar | PubMed |
Chapin FS, III, Autumn K, Pugnaire F (1993) Evolution of suites of traits in response to environmental stress. The American Naturalist 142, S78-S92.
| Crossref | Google Scholar |
Chapman SC, Chakraborty S, Dreccer MF, Howden SM (2012) Plant adaptation to climate change – opportunities and priorities in breeding. Crop & Pasture Science 63, 251-268.
| Crossref | Google Scholar |
Colmer TD, Voesenek LACJ (2009) Flooding tolerance: suites of plant traits in variable environments. Functional Plant Biology 36, 665-681.
| Crossref | Google Scholar | PubMed |
Dear BS, Moore GA, Hughes SJ (2003) Adaptation and potential contribution of temperate perennial legumes to the southern Australian wheatbelt: a review. Australian Journal of Experimental Agriculture 43, 1-18.
| Crossref | Google Scholar |
Di Bella CE, Kotula L, Striker GG, Colmer TD (2020) Submergence tolerance and recovery in Lotus: variation among fifteen accessions in response to partial and complete submergence. Journal of Plant Physiology 249, 153180.
| Crossref | Google Scholar | PubMed |
Escaray FJ, Menendez AB, Gárriz A, Pieckenstain FL, Estrella MJ, Castagno LN, Carrasco P, Sanjuán J, Ruiz OA (2012) Ecological and agronomic importance of the plant genus Lotus. Its application in grassland sustainability and the amelioration of constrained and contaminated soils. Plant Science 182, 121-133.
| Crossref | Google Scholar | PubMed |
Escaray FJ, Antonelli CJ, Carrasco P, Ruiz OA (2019) Interspecific hybridization improves the performance of Lotus spp. under saline stress. Plant Science 283, 202-210.
| Crossref | Google Scholar | PubMed |
Graham PH, Vance CP (2003) Legumes: importance and constraints to greater use. Plant Physiology 131, 872-877.
| Crossref | Google Scholar | PubMed |
James EK, Crawford RMM (1998) Effect of oxygen availability on nitrogen fixation by two Lotus species under flooded conditions. Journal of Experimental Botany 49, 599-609.
| Crossref | Google Scholar |
Kundzewicz ZW, Su B, Wang Y, Wang G, Wang G, Huang J, Jiang T (2019) Flood risk in a range of spatial perspectives–from global to local scales. Natural Hazards and Earth System Sciences 19, 1319-1328.
| Crossref | Google Scholar |
Luo F-L, Nagel KA, Zeng B, Schurr U, Matsubara S (2009) Photosynthetic acclimation is important for post-submergence recovery of photosynthesis and growth in two riparian species. Annals of Botany 104, 1435-1444.
| Crossref | Google Scholar | PubMed |
Manzur ME, Grimoldi AA, Insausti P, Striker GG (2009) Escape from water or remain quiescent? Lotus tenuis changes its strategy depending on depth of submergence. Annals of Botany 104, 1163-1169.
| Crossref | Google Scholar | PubMed |
Marinoni L, Zabala JM, Patiño J, Pensiero JF (2017) Efecto de la temperatura y salinidad en la germinación y crecimiento inicial de un material naturalizado de Lotus tenuis Waldst. & Kit. Fave Sección Ciencias Agrarias 16, 47-60 [In Spanish].
| Google Scholar |
Menon-Martínez FE, Grimoldi AA, Striker GG, Di Bella CE (2021) Variability among Festuca arundinacea cultivars for tolerance to and recovery from waterlogging, salinity and their combination. Crop & Pasture Science 72, 75-84.
| Crossref | Google Scholar |
Nasrullah , Ali S, Umar M, Sun L, Naeem M, Yasmin H, Khan N (2022) Flooding tolerance in plants: from physiological and molecular perspectives. Brazilian Journal of Botany 45, 1161-1176.
| Crossref | Google Scholar |
Nichols P, Craig A, Bonython A, Rogers M-J, Ballard R, Charman N, Hughes S, Colmer T, McClements D, Barrett-Lennard E (2010) Development of Melilotus siculus – a new salt and waterlogging-tolerant annual fodder legume species for Mediterranean-type climates. In ‘Sustainable use of genetic diversity in forage and turf breeding’. (Ed. C Huyghe) pp. 131–135. (Springer: Dordrecht, Netherlands)
Nichols PGH, Revell CK, Humphries AW, Howie JH, Hall EJ, Sandral GA, Ghamkhar K, Harris CA (2012) Temperate pasture legumes in Australia – their history, current use, and future prospects. Crop & Pasture Science 63, 691-725.
| Crossref | Google Scholar |
Poorter H, Nagel O (2000) The role of biomass allocation in the growth response of plants to different levels of light, CO2, nutrients and water: a quantitative review. Functional Plant Biology 27, 1191.
| Crossref | Google Scholar |
Poorter H, Niklas KJ, Reich PB, Oleksyn J, Poot P, Mommer L (2012) Biomass allocation to leaves, stems and roots: meta-analyses of interspecific variation and environmental control. New Phytologist 193, 30-50.
| Crossref | Google Scholar | PubMed |
Sasidharan R, Bailey-Serres J, Ashikari M, Atwell BJ, Colmer TD, Fagerstedt K, Fukao T, Geigenberger P, Hebelstrup KH, Hill RD, Holdsworth MJ, Ismail AM, Licausi F, Mustroph A, Nakazono M, Pedersen O, Perata P, Sauter M, Shih M-C, Sorrell BK, Striker GG, van Dongen JT, Whelan J, Xiao S, Visser EJW, Voesenek LACJ (2017) Community recommendations on terminology and procedures used in flooding and low oxygen stress research. New Phytologist 214, 1403-1407.
| Crossref | Google Scholar | PubMed |
Sasidharan R, Hartman S, Liu Z, Martopawiro S, Sajeev N, van Veen H, Yeung E, Voesenek LACJ (2018) Signal dynamics and interactions during flooding stress. Plant Physiology 176, 1106-1117.
| Crossref | Google Scholar | PubMed |
Signorelli S, Corpas FJ, Borsani O, Barroso JB, Monza J (2013) Water stress induces a differential and spatially distributed nitro-oxidative stress response in roots and leaves of Lotus japonicus. Plant Science 201-202, 137-146.
| Crossref | Google Scholar | PubMed |
Sivakumaran S, Rumball W, Lane GA, Fraser K, Foo LY, Yu M, Meagher LP (2006) Variation of proanthocyanidins in Lotus species. Journal of Chemical Ecology 32, 1797-1816.
| Crossref | Google Scholar | PubMed |
Steffens B, Steffen-Heins A, Sauter M (2013) Reactive oxygen species mediate growth and death in submerged plants. Frontiers in Plant Science 4, 179.
| Crossref | Google Scholar | PubMed |
Striker GG (2012) Time is on our side: the importance of considering a recovery period when assessing flooding tolerance in plants. Ecological Research 27, 983-987.
| Crossref | Google Scholar |
Striker GG, Colmer TD (2017) Flooding tolerance of forage legumes. Journal of Experimental Botany 68, 1851-1872.
| Crossref | Google Scholar | PubMed |
Striker GG, Ploschuk RA (2018) Recovery from short-term complete submergence in temperate pasture grasses. Crop & Pasture Science 69, 745-753.
| Crossref | Google Scholar |
Striker GG, Insausti P, Grimoldi AA, Ploschuk EL, Vasellati V (2005) Physiological and anatomical basis of differential tolerance to soil flooding of Lotus corniculatus L. and Lotus glaber Mill. Plant and Soil 276, 301-311.
| Crossref | Google Scholar |
Striker GG, Insausti P, Grimoldi AA (2008) Flooding effects on plants recovering from defoliation in Paspalum dilatatum and Lotus tenuis. Annals of Botany 102, 247-254.
| Crossref | Google Scholar | PubMed |
Striker GG, Manzur ME, Grimoldi AA (2011) Increasing defoliation frequency constrains regrowth of the forage legume Lotus tenuis under flooding. The role of crown reserves. Plant and Soil 343, 261-272.
| Crossref | Google Scholar |
Striker GG, Izaguirre RF, Manzur ME, Grimoldi AA (2012) Different strategies of Lotus japonicus, L. corniculatus and L. tenuis to deal with complete submergence at seedling stage. Plant Biology 14, 50-55.
| Crossref | Google Scholar | PubMed |
Striker GG, Casas C, Manzur ME, Ploschuk RA, Casal JJ (2014) Phenomic networks reveal largely independent root and shoot adjustment in waterlogged plants of Lotus japonicus. Plant, Cell & Environment 37, 2278-2293.
| Crossref | Google Scholar | PubMed |
Striker GG, Casas C, Kuang X, Grimoldi AA (2017) No escape? Costs and benefits of leaf de-submergence in the pasture grass Chloris gayana under different flooding regimes. Functional Plant Biology 44, 899-906.
| Crossref | Google Scholar | PubMed |
Striker GG, Kotula L, Colmer TD (2019) Tolerance to partial and complete submergence in the forage legume Melilotus siculus: an evaluation of 15 accessions for petiole hyponastic response and gas-filled spaces, leaf hydrophobicity and gas films, and root phellem. Annals of Botany 123, 169-180.
| Crossref | Google Scholar | PubMed |
Teakle NL, Real D, Colmer TD (2006) Growth and ion relations in response to combined salinity and waterlogging in the perennial forage legumes Lotus corniculatus and Lotus tenuis. Plant and Soil 289, 369-383.
| Crossref | Google Scholar |
Voesenek LACJ, Bailey-Serres J (2013) Flooding tolerance: O2 sensing and survival strategies. Current Opinion in Plant Biology 16, 647-653.
| Crossref | Google Scholar | PubMed |
Voesenek LACJ, Colmer TD, Pierik R, Millenaar FF, Peeters AJM (2006) How plants cope with complete submergence. New Phytologist 170, 213-226.
| Crossref | Google Scholar | PubMed |
Weih M (2001) Evidence for increased sensitivity to nutrient and water stress in a fast-growing hybrid willow compared with a natural willow clone. Tree Physiology 21, 1141-1148.
| Crossref | Google Scholar | PubMed |
Wilson JB (1988) Shoot competition and root competition. Journal of Applied Ecology 25, 279-296.
| Crossref | Google Scholar |